Abstract
Context Andrographolide containing Andrographis paniculata (Burm. F.) Wall. Ex Nees (Acanthaceae) extracts is often used for treatments of diabetes and other inflammatory disorders commonly accompanying cognitive and other psychiatric disorders.
Objective To compare the efficacies of a standardised A. paniculata extract (AP) and pure andrographolide on cognitive functions, oxidative stress and cholinergic function in diabetic rats.
Materials and methods Streptozotocin-induced diabetic Charles Foster albino rats treated orally with a hydro-methanolic A. paniculata leaf extract (50, 100 and 200 mg/kg/day), or with pure andrographolide (15, 30 and 60 mg/kg/day) for 10 consecutive days, were subjected to Morris water maze test. After the test, acetylcholinesterase, superoxide dismutase (SOD), and catalase (CAT) activities and lipid peroxidation (LPO) in brain tissues were assessed.
Results Acetylcholinesterase activity in pre-frontal cortex and hippocampus of diabetic rats was 2.1 and 2.6 times higher compared to nondiabetic rats. LPO was 1.6 times higher and decreased SOD (56.3%) and CAT (44.9%) activities in pre-frontal cortex of diabetic rats compared to nondiabetic rats. AP or andrographolide treatments dose dependently attenuated cognitive deficits, reduced acetylcholinesterase activity, oxidative stress, improved diabetic hyperglycemia and insulin deficiency. All observed effects of AP were quantitatively almost equal to those expected from its analytically quantified andrographolide content.
Discussion and conclusion Reported observations are the very first ones suggesting beneficial effects of andrographolide against diabetes associated cognitive deficits, increased acetylcholinesterase activity and deteriorated antioxidative status. Efforts to exploit A. paniculata extracts enriched in andrographolide as preventive measures against such disorders can be warranted.
Introduction
Diabetes is a silently and slowly progressing metabolic disorder eventually affecting almost all bodily organs including the brain (Ducat et al. Citation2014). A vast majority of global population now suffering from, or at risk to, diabetes and associated comorbidities live in China, India and other economically developing or underdeveloped countries (Chen et al. Citation2012), where due to socio-economic, cultural and diverse other reasons traditionally known medicinal herbs are often used for prevention and cure of such multi-morbidities. Andrographis paniculata (Burm. F.) Wall. Ex Nees (Acanthaceae) is one of the numerous traditionally known and more extensively studied ayurvedic medicinal plants now often used for the treatment of diabetic hyperglycemia (Hossain et al. Citation2014). Andrographolide, a labdane diterpenoid (), is quantitatively the major, but not the only, bioactive secondary metabolite of the plant involved in antidiabetic, hepatoprotective, antioxidant, anti-inflammatory and diverse other therapeutically interesting bioactivities of its extracts (Akbar Citation2011; Parixit et al. Citation2012; Jayakumar et al. Citation2013). Although a number of pre-clinical reports suggesting antidiabetic activities of andrographolide and A. extracts have consistently increased during more recent years, to date only one preliminary clinical report suggesting such efficacy of A. paniculata leaf powder in diabetic patients has appeared (Agarwal et al. Citation2005). Most reports on clinical studies with A. paniculata extracts have concentrated mainly on their therapeutic potential for the treatment of upper respiratory tract infections and common cold (Saxena et al. Citation2010; Panossian & Wikman Citation2013). A recently published assessment report of European Medicine Agency (HMPC Citation2014) has concluded though, that a well-established monograph cannot be granted to A. paniculata, leaf, and the efficacy is not plausible because of the lack of evidence of traditional application.
In ayurvedic and other traditionally known Indian systems of medicine, A. paniculata is often used as a tonic or rejuvenating herb in combination with other herbs and minerals. Several such medicinal plants are now pharmacologically classified as stress response modulators or adaptogenic herbs (Panossian & Wikman Citation2009; Pawar & Shivakumar Citation2012). Recent observations in our laboratories have not only revealed adaptogens like stress response modulating, anxiolytic, antidepressant activities of a medicinally used A. paniculata extract and andrographolide, but also have reconfirmed their anti-hyperglycemic activities in diabetic rats (Kumar et al. Citation2014; Thakur et al. Citation2014a,Citation2014b,Citation2015; Thakur, Soni, et al. Citation2014). An independent report has revealed nootropic and cerebro-protective effects of methanolic extract of A. paniculata leaves (Radhika et al. Citation2012), and neuroprotective and cognitive function improving effects of intraperitoneally administered pure andrographolide has also been reported (Chan et al. Citation2010; Serrano et al. Citation2014). However, the questions concerning the role of andrographolide in brain function modulating effects of A. paniculata extracts, or on the role of oxidative and cholinergic processes in their effects observed after their repeated oral doses remain unanswered or at best can have speculative answers only.
The very first observations revealing cognitive function improving effects of repeated daily oral doses of the analytically well-standardised A. paniculata extract (KalmCold®) studied in our laboratories, and suggesting that andrographolide is indeed the quantitatively major, if not the only, brain function modulating constituent of the medicinally used extract in diabetic rats is described and discussed in this communication. Doses and treatment regimen of the extract and andrographolide used in this study were the same used in on our earlier study revealing its metabolic function improving effects in diabetic and obese rats (Thakur et al. Citation2014a), and on analytically quantified andrographolide content of the extract. Since a report has revealed nootropics like cognitive function improving effects of an A. paniculata extract in Morris water maze test (Radhika et al. Citation2012), the clinically used nootropic drug piracetam was used as a reference drug in this study.
Materials and methods
Animals
Male adult Charles Foster albino rats (150 ± 10 g) were from Central Animal House of the Institute of Medical Sciences, Banaras Hindu University, Varanasi, India (Registration Number: 542/AB/CPCSEA). Six randomly selected animals were assigned to each experimental group used in the study. They were housed in groups of six in polypropylene cages and maintained in ambient temperature of 25 ± 1 °C and 45–55% relative humidity, with a 12:12 h light/dark cycle. They were supplied with commercial food pellets (Pranav Agro Industries Ltd., Sangali, India) and water ad libitum, and were acclimatised to laboratory conditions for 1 week before subjecting them to experimental conditions. Principles of laboratory animal care (NIH publication number 85-23, revised in 1985) guidelines were always followed. Prior approval from the Central Animal Ethical Committee of Banaras Hindu University, Varanasi, India was obtained for the experimental protocols (Dean 11-12/CAEC/325, dated 30.11.2011).
Drugs and chemicals
Standardised hydro-methanolic A. paniculata leaf extract (AP) and andrographolide (99.0% pure by HPLC; ) were generously supplied by Natural Remedies Pvt. Ltd. (Bangalore, India). The leaves of plant were collected in the month of March and identified as A. paniculata (Burm. F.) Wall. Ex Nees by in-house botanist Dr. Gopal Sangli at R&D Center of Natural Remedies Pvt. Ltd., Bangalore, India, and a voucher herbarium specimen (No. NR582) is available in their laboratory. Extraction procedure and analytical methods used for obtaining and standardising AP and andrographolide have been described in detail elsewhere (Chandrasekaran et al. Citation2011; Thakur, Soni, et al., Citation2014; Thakur et al. Citation2015). AP was analytically characterised to contain andrographolide (32.2%, w/w), isoandrographolide (0.5%, w/w), neoandrographolide (2.7%, w/w), andrograpanin (0.9%, w/w), and 14-deoxy-11,12-didehydroandrographolide (4.7%, w/w), and skullcapflavone I (0.06%, w/w) by HPLC. Other chemicals and reagents used were from commercial sources.
Streptozotocin-induced diabetes
Diabetes was induced in overnight fasted rats by a single intra-peritoneal (i.p.) injection of 65 mg/kg streptozotocin (STZ; HiMedia, Mumbai, India). As described elsewhere (Thakur et al. Citation2014a), STZ injections were given 15 min after the i.p. administration of 120 mg/kg nicotinamide (SD Fine Chemical Ltd., Mumbai, India). After STZ injections, the animals were returned to their cages and were provided with normal food and 10% sucrose water (during in the first 48 h only) to minimise hypoglycemic shock. Only pre-selected diabetic animals with blood glucose level higher than 250 mg/dL on the seventh day after STZ injection were used as diabetic animals for the experiments. Plasma glucose and insulin level were quantified by the method described below.
Animal grouping and drug treatments
Nine randomly assigned experimental groups of six animals each were: (1) Nondiabetic control (vehicle treated); (2) Diabetic control (vehicle treated); (3) Diabetic + AP 50 mg/kg/day; (4) Diabetic + AP 100 mg/kg/day; (5) Diabetic + AP 200 mg/kg/day; (6) Diabetic + andrographolide 15 mg/kg/day; (7) Diabetic +andrographolide 30 mg/kg/day; (8) Diabetic + andro-grapholide 60 mg/kg/day and (9) Diabetic + piracetam 100 mg/kg/day (reference or positive control group). AP and piracetam were suspended in 0.3% carboxymethylcellulose (CMC) for once daily oral administrations for 10 consecutive days. For oral administrations, andrographolide was first macerated with Tween 80 before suspending it in water (final Tween 80 concentration in the suspension was 0.2%). Oral application volume was 10 ml/kg, and the control groups were treated with 0.3% CMC only. All oral treatments were given 60 min before performing behavioural tests. Other details of the experiments are summarised in .
Morris water maze test
The hidden platform version of this test (D'Hooge & De Deyn Citation2001) was used for assessing the effects of AP and andrographolide treatments on learning ability and short-term memory of diabetic rats. A locally fabricated metal semicircular pool maze (120 cm in diameter, 45 cm deep) filled with water to a depth of approximately 25 cm was used. The pool was divided into four equal quadrants, and a platform (15 cm in diameter) was submerged 0.5 cm below the opaque surface in the centre of one of the quadrants. The pool was located in a test room and many cues external to the maze were visible from the pool (e.g., pictures and statues), which could be used by the rats for spatial orientation. The positions of the cues were kept constant throughout the task on all observational days. Animals of all groups were habituated to the maze on the sixth treatment day. As described below, spatial reference memory tests were conducted on the seventh, eighth and ninth treatment days, and the probe trials for retention test were given on day 10 of treatments. A blinded observer recorded escape latencies during training sessions and time spent in target quadrant during the probe trial.
Habituation: Twenty-four hours prior to the start of spatial reference memory test (on day 6 of drugs treatment), all rats used in the study were habituated to the pool by allowing them to perform a 60-s swim without the platform.
Spatial reference memory test: An individual of a given group was placed by hand into the water facing the wall of the pool and was allowed up to 60 s to find the hidden platform. When successful, the rat was given 20 s stay on the platform to watch the spatial cues. If the animal failed to find the platform, it was manually guided to stay there for 20 s. Each animal had eight trials per day (separated by 10 min), for three consecutive days (days 7–9) on which the escape latencies were recorded. The animals were returned to their home cages after each trial.
Probe trial for retention test: This trial consisted of a 60-s free swim period without a platform. The blind observer recorded the number of times a rat crossed the platform location and total time spent by the rat in the target quadrant (i.e., the quadrant where hidden platform was placed during spatial reference memory tests).
Blood glucose and insulin assays
Blood samples were collected by the retro-orbital venous plexus sampling method on day 10 after performing probe trial test. Plasma was prepared by cold centrifugation (5 °C) at 3000 rpm (845g) for 5 min (Compufuge CPR-30 Plus, with Rotor No. 8; REMI, Mumbai, India) and stored in a freezer (−20 °C) till use for biochemical analysis. A commercially available test kit, based on glucose oxidase–peroxidase method (Autospan Glucose test kit; Beacon Diagnostic Pvt. Ltd., Navasari, India), was used for quantifying plasma glucose levels. Plasma insulin level was quantified by using an enzyme linked immunosorbent assay test kit (DRG Instruments GmbH, Marburg, Germany). Both glucose and insulin analyses were performed by using an absorbance micro-plate reader (iMark™, Bio-Rad Laboratories, Hercules, CA) according to instruction manuals of the test kits.
Brain tissues sampling
Immediately after collections of blood samples (after probe trial test) on the 10th treatment day, rats were sacrificed by cervical dislocation, and their brain pre-frontal cortex and hippocampus were dissected out and weighed. Pre-frontal cortex was used for assaying brain oxidative status, and pre-frontal cortex and hippocampus samples were used for estimating brain acetylcholinesterase activity. The dissected samples were stored at −80 °C in a laboratory deep freeze until used for the assays.
Brain oxidative status
The pre-frontal cortex was homogenised in 10 volumes (1:10 w/v) of 20 mM sodium phosphate buffer (pH 7.4) containing 140 mM KCl using a Teflon-glass homogeniser. The homogenates were centrifuged at 750g for 10 min at 4 °C. Lipid peroxidation (LPO), and superoxide dismutase (SOD) and catalase (CAT) activities were analyzed in the supernatants of tissue homogenates by using a microplate absorbance reader (iMark-Bio-Rad Laboratories). LPO was quantified by measuring malondialdehyde (MDA) formed by LPO, and expressed as nmol MDA/mg protein (Ohkawa et al. Citation1979). For SOD and CAT activities, the methods described by Kakkar et al. (Citation1984) and Luck (Citation1963) were strictly followed. CAT activities are expressed as μmol H2O2 decomposed/min/mg protein, and SOD activities are expressed in units of SOD activity/mg protein. Protein contents in the homogenates were quantified by the method of described by Lowry et al. (Citation1951).
Acetylcholinesterase activity
Acetylcholinesterase activities were quantified in 20% of homogenates of pre-frontal cortex and hippocampus in phosphate buffer (0.1 M; pH 8) using actyl-thiocholine as a substrate and following the method described by Ellman et al. (Citation1961). Thiocholine generated by hydrolysis of the substrate by the cholinesterases, and forming yellow coloured product by reacting with 5,5′-dithiobis(2-nitrobenzoic acid), was quantified. Intensity of the colour formed was measured at 415 nm using the iMark™ Micro-plate Reader. Enzymatic activities were expressed as μmol substrate hydrolyzed/min/mg protein in the homogenates.
Statistical analysis
Mean ± standard error of mean (SEM) was calculated for the observed values in each experimental group (n=6). Statistical analysis was performed by one-way analysis of variance (ANOVA) followed by Student–Newman–Keuls multiple comparison test for all data except for those shown in , the analysis for which was done by two-way ANOVA followed by Bonferroni post-tests. GraphPad Prism-6 software (GraphPad Software Inc., La Jolla, CA) was used for statistical analysis. p Value less than 0.05 was always considered as statistically significant.
Figure 3. Effects of A. paniculata extract (AP), andrographolide (Andro), or piracetam on escape latencies to find the platform (on days 7–9) during Morris water maze test. Data are shown as the mean ± SEM (n=6). *p < 0.05 versus nondiabetic (ND) control; ¥p < 0.05 versus diabetic (D) control (two-way ANOVA followed by Bonferroni post tests).
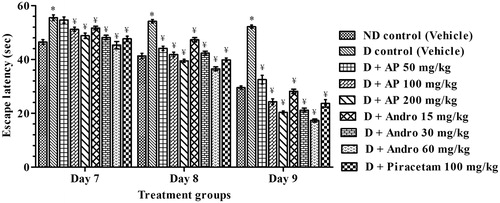
Results
Morris water maze test
Amnesic behaviour of vehicle-treated diabetic control rats was apparent from significant increases in escape latencies to find the platform during spatial reference memory test and less time spent in targeted quadrant during retention test (probe trial) compared to nondiabetic control rats. In comparison to the CMC-treated diabetic group, the escape latencies of AP (50, 100 and 200 mg/kg), or andrographolide (15, 30 and 60 mg/kg), or piracetam (100 mg/kg) treated ones on day 7 [F(8, 45) = 12.90, p < 0.05], day 8 [F(8, 45) = 44.20, p < 0.05] and day 9 [F(8,45) = 117.00, p < 0.05] of spatial reference memory test were significantly lower. Statistically significant interaction between drug-treated groups [F(8, 135) = 133.00, p < 0.05] and treatment days [F (2, 135) = 1406.00, P < 0.05] were asserted by further analysis of reference memory test data by two-way ANOVA analysis (). Post hoc analysis revealed that on days 7, 8 and 9 of the test, treatment with AP, andrographolide or piracetam significantly decreased escape latencies compared to diabetic control indicating increased memory functions. During the probe trial (), the mean numbers of crossings of the drug treated diabetic groups in the target quadrant where the platform had been located and the mean time they spent there were significantly higher than those observed in the diabetic control group (one-way ANOVA analysis; [F(8, 45) = 4.28, p < 0.05] and [F(8, 45) = 48.40, p < 0.05], respectively). Qualitatively, the observed effects of AP and andrographolide were similar to those of piracetam, and quantitatively the observed efficacies of the AP doses tested were quite close to those expected from its analytically quantified andrographolide content.
Figure 4. Effects of A. paniculata extract (AP), andrographolide (Andro), or piracetam on number of crossing (N) and time spent (s) in targeted quadrant (probe trial on day 10) during Morris water maze test. Data are shown as the mean ± SEM (n=6). *p<0.05 versus nondiabetic (ND) control; ¥p<0.05 versus diabetic (D) control (one-way ANOVA followed by Student–Newman–Keuls multiple comparison test).
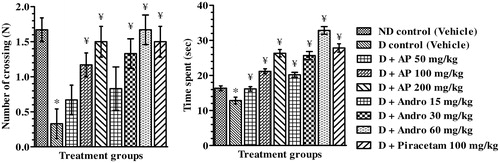
Blood glucose and insulin level
Mean blood glucose and insulin levels of the different groups quantified in blood samples collected just after completion of probe trials in Morris water maze test are summarised in . As compare to the vehicle-treated nondiabetic group, mean blood glucose levels of the vehicle treated diabetic group was almost three times higher, and their mean circulating insulin levels were ca. four folds lower. No statistically significant effects of 100 mg/kg/day piracetam treatments to diabetic rats on their blood glucose or insulin levels were observed. However, in comparison to the vehicle-treated diabetic control group, AP or andrographolide treated ones had significantly (one-way ANOVA analysis) lower blood glucose levels [F(8, 45) = 671.00, p < 0.05], and higher blood insulin level [F(8, 45) = 99.40, p < 0.05]. These dose-dependant effects of AP in diabetic rats observed in this study were quite similar in magnitude to those of the extract reported earlier (Thakur et al. Citation2014a).
Figure 5. Effects of A. paniculata extract (AP), andrographolide (Andro), or piracetam on blood glucose and insulin level on day 10 after performing probe trial in Morris water maze test. Data are shown as the mean ± SEM (n=6). *p<0.05 versus nondiabetic (ND) control; ¥p<0.05 versus diabetic (D) control (one-way ANOVA followed by Student–Newman–Keuls multiple comparison test).
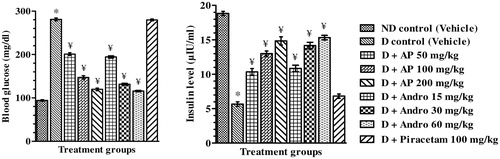
Antioxidative status in pre-frontal cortex
The results summarised in revealed that in comparison to the nondiabetic control group, mean LPO levels in the pre-frontal cortex of the diabetic control group were significantly higher (1.6 times), and the mean SOD and CAT activities in the brain region of diabetic rats were significantly lower (by 56.3% and 44.9%, respectively). These hyperglycemia and hypoinsulinemia associated alterations in brain LPO levels and SOD and CAT activities were significantly less severe in piracetam-treated diabetic rats. In comparison to the corresponding mean values of vehicle-treated diabetic control group, LPO levels in AP- or andrographolide-treated diabetic ones were significantly lower [F(8, 45) = 33.70, p < 0.05]. SOD and CAT activities in AP- or andrographolide-treated diabetic groups were significantly [F(8, 45) = 23.70, p < 0.05 for SOD and F(8, 45) = 38.80, p < 0.05 for CAT] higher (one-way ANOVA analysis) that those of the vehicle-treated diabetic control group. Quantitatively, the efficacies of AP treatments were usually similar in magnitude to those expected from its analytically quantified andrographolide content.
Figure 6. Effects of A. paniculata extract (AP), andrographolide (Andro), or piracetam on brain oxidative status, viz. LPO (nmol MDA/mg protein), SOD (unit/mg protein) and CAT (μmol H2O2/min/mg protein), assessed after completion of probe trial in Morris water maze test. Data are shown as the mean ± SEM (n=6). *p < 0.05 versus nondiabetic (ND) control; ¥p < 0.05 versus diabetic (D) control (one-way ANOVA followed by Student–Newman–Keuls multiple comparison test).
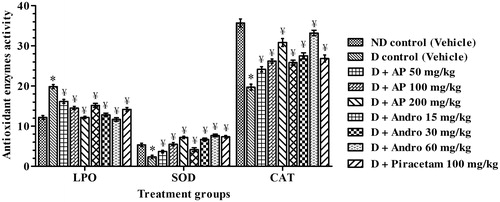
Acetylcholinesterase enzyme activity
The results summarised in revealed that acetylcholinesterase activities in cortex and hippocampus of diabetic rats were significantly higher (2.1 and 2.6 times, respectively) than those quantified in the same regions of vehicle-treated nondiabetic ones. These results are quite analogous to those reported by others (Bhutada et al. Citation2011). In comparison to the corresponding values of the diabetic control group, the mean acetylcholinesterase activities in pre-frontal cortex [F(8, 45) = 68.69, p < 0.05] and in hippocampus [F(8, 45) = 65.09, p < 0.05], of AP-, andrographolide- or piracetam-treated ones, were significantly lower (one-way ANOVA analysis). Observed dose-dependent efficacies of AP in lowering acetylcholinesterase activities in both the brain regions of diabetic rats were almost identical to those expected from its andrographolide contents. Efficacy of 60 mg/kg/day andrographolide quite similar in magnitude to those observed for 100 mg/kg/day dose of the reference nootropic drug piracetam used in the study. Enzymatic activities in both the brain regions of diabetic rats treated with the highest tested daily doses of AP (200 mg/kg), or of andrographolide (60 mg/kg), were quite close to those of the nondiabetic ones.
Figure 7. Effects of A. paniculata extract (AP), andrographolide (Andro) or piracetam on acetylcholinesterase enzyme activities in brain regions quantified after completion of probe trials in Morris water maze test. Data are shown as the mean ± SEM (n=6). *p < 0.05 versus nondiabetic (ND) control; ¥p < 0.05 versus diabetic (D) control (one-way ANOVA followed by Student–Newman–Keuls multiple comparison test).
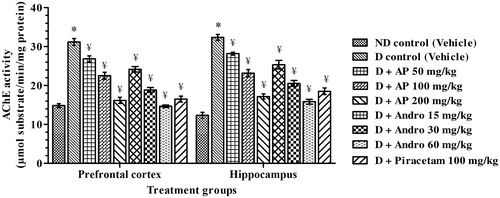
Discussion
Observations reported in this communication reveal that STZ-induced diabetic rats used in this and our earlier studies develop cognitive deficits and suggest that such deficits are most probably due to deteriorated oxidative status and elevated acetylcholinesterase activities in their brains. They are also the very first ones revealing cognitive function improving potentials of andrographolide and of the A. paniculata extract studied. Although this medicinally used extract (KalmCold®, or AP) is highly enriched in andrographolide (32.2%, w/w), apart from such labdane terpenes this and other andrographolide enriched extracts of the plant also contain numerous other bioactive phytochemical with andrographolide like anti-inflammatory and other bioactivities (Chandrasekaran et al. Citation2011; Low et al. Citation2015). However, the observed efficacies of AP and pure andrographolide in counteracting cognitive deficits and all biochemical abnormalities in the brain quantified in this study were always qualitatively as well as quantitatively almost equal. Therefore, it seems reasonable to assume that andrographolide is the only cognitive function-modulating constituent of the extract tested.
Obesity and even pre-diabetes state are well-recognised risk factor for dementia and Alzheimer’s disease (Gudala et al. Citation2013; Butterfield et al. Citation2014), and during recent years, suggestions have often made that structurally and functionally diverse secondary plant metabolites are potential therapeutic leads for prevention and cure of diabetes-associated dementia (Davinelli et al. Citation2012; Recio et al. Citation2012; Apetz et al. Citation2014). By far a vast majority of such suggestive reports concentrate on plant polyphenolics with antioxidative properties, and a more recent report concludes that inhibition of acetylcholinesterase could also be involved in memory function improving effects of structurally diverse phytochemicals and plant polyphenolics (Huang et al. Citation2013). Antioxidative activities of A. paniculata and andrographolide and their other constituents have been described (Lin et al. Citation2009; Chao & Lin Citation2010), and acetylcholinesterase inhibitory activities of AP and andrographolide in rat brain homogenates have recently been observed in our laboratories (results not shown). However, concentrations of andrographolide necessary for observing its effects in bioassays conducted in vitro were several folds higher than that of its maximal blood levels quantified after its oral intake in rats (Panossian et al. Citation2000). Therefore, the presented study was conducted to verify in vivo relevance of these in vitro observations made in our laboratories and elsewhere.
Results of the experiment reconfirmed that 50 and 15 mg/kg/day are the minimally effective oral anti-hyperglycemic doses of AP and andrographolide in diabetic rats, and reveal that their learning and memory function improving effects are qualitatively quite similar to those of piracetam. Their observed effects in reversing diabetes-associated elevation in brain acetylcholinesterase activity and oxidative status in diabetic rats were also qualitatively similar to those of the nootropic drug. However, unlike piracetam, oral AP and andrographolide treatments also had beneficial effects against diabetes associated hyperglycemia and insulin deficiencies. Therefore, it seems reasonable to assume that the modes of actions of andrographolide or AP are not identical to the reference nootropic and memory-improving drug, and that unlike piracetam both AP and andrographolide are regulators of peripheral glucose homeostasis in diabetic rats.
Dose–response relationships of both AP and andrographolide for their observed effects on all behavioural and biochemical parameters quantified in the reported experiment were quite similar to those reported earlier for their antidepressant like activity of AP in such animals (Thakur et al. Citation2014c). In the last mentioned report, observed dose dependency of beneficial effects of AP against abnormal brain monoamine levels and monoamine oxidase activities in diabetic rats was also similar. Therefore, it could as well be that modulation of glucose homeostasis in diabetic rats by AP or andrographolide is involved in their peripheral as well as central effects in such animals. However, further studies that are more detailed will be necessary for a more definitive conclusion.
In any case, it seems certain that andrographolide is the major, if not the only, bioactive constituent involved in cognitive function modulating and other beneficial effects of AP in diabetic rats. This assertion must not necessarily be true though, for diverse other therapeutically interesting bioactivities of AP observed in nondiabetic animals (or in patients and volunteers). Initial observation made in our laboratories for assessing safety ranges and chronic mild stress response suppressing doses and treatment regimen of AP and andrographolide in normal mice had revealed that pharmacologically interesting and effective dose range of pure andrographolide is lower than that of AP (Thakur et al. Citation2014b, Citation2015). In another study, 50 mg/kg/day oral doses of AP for 21 consecutive days were high enough for its maximal possible efficacy in suppressing the biomarkers of inflammation in chronically stressed nondiabetic rats (Thakur, Soni, et al. Citation2014). In contrast to these observations, minimally effective daily oral doses of AP in diabetic animals were always 50 mg/kg/day and that of andrographolide 15 mg/kg/day (Thakur et al. Citation2014a, Citation2014c), i.e., their minimally effective daily oral dose for their cognitive function improving and brain acetylcholinesterase activating modulating effects in diabetic rats observed in this study. Taken together, these observations indicate either that efficacies of andrographolide and AP in diabetic animals are lower than in nondiabetic ones, or that their stress response regulating and anti-inflammatory activities are not involved in their observed effects in diabetic rats.
It is now well established that gut microbiota plays a crucial role in aetiology pathogenesis and progression of metabolic disorders and mental health problems (Thakur, Shakya, et al. Citation2014), and that numerous phytochemicals can not only alter gut microbial ecology (Abreu et al. Citation2012; Borges et al. Citation2015), but also are extensively anabolised and catabolised inside the gastrointestinal tract (Laparra & Sanz Citation2010). Andrographolide and diverse other extractable secondary metabolites of A. paniculata possess bactericidal, fungicidal and antiviral activities (Sareer et al. Citation2012), and oral bioavailability of andrographolide (as judged by its observed blood levels after oral intake) is low (Panossian et al. Citation2000; Ye et al. Citation2011). Andrographolide modulates physiological functions of diverse drug metabolising enzymes in liver and other peripheral organs as well (Pekthong et al. Citation2008), and binds covalently with cellular metabolites and enzymes (Xia et al. Citation2004). Andrographolide–lipoic acid conjugate possesses andrographolide like and longer lasting antidiabetic activity in mice (Zhang et al. Citation2009). Therefore, it could as well be that the observed beneficial effects of andrographolide on memory functions and AChE and antioxidative enzymes in the brain observed in this study are due to its conjugates. However, direct effects of andrographolide on drug metabolising enzymes, glucose homeostasis and oxidative processes could also contribute to its broad spectrum of therapeutically interesting bioactivities. Since gut microbial ecology in STZ diabetic rats is altered (Wirth et al. Citation2014), it could as well be that lower efficacy of andrographolide and AP in such animals is due to differences in their microbicidal activity against different microbial colonies (Xu et al. Citation2006), and that their primary sites of actions lie inside the gastrointestinal tract.
Efforts to clarify possible involvements of such processes in the experimentally observed differences between the efficacies of andrographolide and AP, or to better define the role of andrographolide and other extractable secondary metabolites of A. paniculata in traditionally known and other medicinal uses of the plant, will be necessary for more definitive assertions. Such efforts will not only be useful for pinpointing pharmacological targets and sites of actions of andrographolide involved in its broad spectrum of therapeutically interesting activity profiles, but also for obtaining novel therapeutic leads and pharmacological strategies urgently needed for prevention and cure of diabetes and other metabolic disorders associated cognitive disorders and other mental health problems.
Conclusion
Observations reported in this communication strongly suggest that AP and andrographolide could be a therapeutic option for prevention or cure of cognitive disorders often encountered in diabetic patients. Efforts to identify the biological processes and mechanisms involved in their observed and diverse other effects could not only lead to novel pharmacological targets and strategies urgently needed for prevention and cure of diabetes associated comorbidities, but also to better understanding of pharmacological principles involved in widespread uses of A. paniculata in traditionally known systems of medicine.
Acknowledgement
The authors acknowledge Natural Remedies Pvt. Ltd. (Bangalore, India) for providing complementary samples of standardised extract of A. paniculata and pure andrographolide.
Declaration of interest
The authors declare that there are no conflicts of interest in this study. A.K.T. greatly acknowledge the Department of Science and Technology, Government of India, New Delhi for awarding INSPIRE Fellowship (IF110595).
References
- Abreu AC, McBain AJ, Simoes M. 2012. Plants as sources of new antimicrobials and resistance-modifying agents. Nat Prod Rep. 29:1007–1021.
- Agarwal R, Sulaiman SA, Mohamed M. 2005. Open label clinical trial to study adverse effects and tolerance to dry powder of the aerial part of Andrographis paniculata in patients type 2 with diabetes mellitus. Malays J Med Sci. 12:13–19.
- Akbar S. 2011. Andrographis paniculata: a review of pharmacological activities and clinical effects. Altern Med Rev. 16:66–77.
- Apetz N, Munch G, Govindaraghavan S, Gyengesi E. 2014. Natural compounds and plant extracts as therapeutics against chronic inflammation in Alzheimer's disease – a translational perspective. CNS Neurol Disord Drug Targets. 13:1175–1191.
- Bhutada P, Mundhada Y, Bansod K, Tawari S, Patil S, Dixit P, Umathe S, Mundhada D. 2011. Protection of cholinergic and antioxidant system contributes to the effect of berberine ameliorating memory dysfunction in rat model of streptozotocin-induced diabetes. Behav Brain Res. 220:30–41.
- Borges A, Saavedra MJ, Simoes M. 2015. Insights on antimicrobial resistance, biofilms and the use of phytochemicals as new antimicrobial agents. Curr Med Chem. 22:2590–2614.
- Butterfield DA, Di Domenico F, Barone E. 2014. Elevated risk of type 2 diabetes for development of Alzheimer disease: a key role for oxidative stress in brain. Biochim Biophys Acta. 1842:1693–1706.
- Chan SJ, Wong WS, Wong PT, Bian JS. 2010. Neuroprotective effects of andrographolide in a rat model of permanent cerebral ischaemia. Br J Pharmacol. 161:668–679.
- Chandrasekaran CV, Thiyagarajan P, Deepak HB, Agarwal A. 2011. In vitro modulation of lps/calcimycin induced inflammatory and allergic mediators by pure compounds of Andrographis paniculata (king of bitters) extract. Int Immunopharmacol. 11:79–84.
- Chao WW, Lin BF. 2010. Isolation and identification of bioactive compounds in Andrographis paniculata (Chuanxinlian). Chin Med. 5:1–15.
- Chen L, Magliano DJ, Zimmet PZ. 2012. The worldwide epidemiology of type 2 diabetes mellitus-present and future perspectives. Nat Rev Endocrinol. 8:228–236.
- Davinelli S, Sapere N, Zella D, Bracale R, Intrieri M, Scapagnini G. 2012. Pleiotropic protective effects of phytochemicals in Alzheimer's disease. Oxid Med Cell Longev. 2012:1–11.
- D'Hooge R, De Deyn PP. 2001. Applications of the Morris water maze in the study of learning and memory. Brain Res Brain Res Rev. 36:60–90.
- Ducat L, Philipson LH, Anderson BJ. 2014. The mental health comorbidities of diabetes. JAMA. 312:691–692.
- Ellman GL, Courtney KD, Andres V Jr, Feather-Stone RM. 1961. A new and rapid colorimetric determination of acetylcholinesterase activity. Biochem Pharmacol. 7:88–95.
- Gudala K, Bansal D, Schifano F, Bhansali A. 2013. Diabetes mellitus and risk of dementia: a meta-analysis of prospective observational studies. J Diabetes Investig. 4:640–650.
- HMPC. 2014. Committee on Herbal Medicinal Products (HMPC). Assessment report on Andrographis paniculata Nees, folium [Internet]; [cited 2015 Jun 3]. Available from: http://www.ema.europa.eu/docs/en_GB/document_library/Herbal_-_HMPC_assessment_report/2014/06/WC500168112.pdf.
- Hossain MS, Urbi Z, Sule A, Hafizur Rahman KM. 2014. Andrographis paniculata (Burm. F.) Wall. ex Nees: a review of ethnobotany, phytochemistry, and pharmacology. Sci World J. 2014:1–28.
- Huang L, Su T, Li X. 2013. Natural products as sources of new lead compounds for the treatment of Alzheimer's disease. Curr Top Med Chem. 13:1864–1878.
- Jayakumar T, Hsieh CY, Lee JJ, Sheu JR. 2013. Experimental and clinical pharmacology of Andrographis paniculata and its major bioactive phytoconstituent andrographolide. Evid Based Complement Alternat Med. 2013:1–16.
- Kakkar P, Das B, Viswanathan PN. 1984. A modified spectrophotometric assay of superoxide dismutase. Indian J Biochem Biophys. 21:130–132.
- Kumar V, Thakur AK, Chatterjee SS. 2014. Perspective of Andrographis paniculata in neurological disorders. Clin Pharmacol Biopharm. S2:1–4.
- Laparra JM, Sanz Y. 2010. Interactions of gut microbiota with functional food components and nutraceuticals. Pharmacol Res. 61:219–225.
- Lin FL, Wu SJ, Lee SC, Ng LT. 2009. Antioxidant, antioedema and analgesic activities of Andrographis paniculata extracts and their active constituent andrographolide. Phytother Res. 23:958–964.
- Low M, Khoo CS, Munch G, Govindaraghavan S, Sucher NJ. 2015. An in vitro study of anti-inflammatory activity of standardised Andrographis paniculata extracts and pure andrographolide. BMC Complement Altern Med. 15:1–9.
- Lowry OH, Rosebrough NJ, Farr AL, Randall RJ. 1951. Protein measurement with the Folin phenol reagent. J Biol Chem. 193:265–275.
- Luck H. 1963. Catalaze. In: Bergmeyers HU, editor. Methods of enzymatic analysis. Weinheim and New York: Verlag Chemie, Academic Press. p. 885–888.
- Ohkawa H, Ohishi N, Yagi K. 1979. Assay for lipid peroxides in animal tissues by thiobarbituric acid reaction. Anal Biochem. 95:351–358.
- Panossian A, Hovhannisyan A, Mamikonyan G, Abrahamian H, Hambardzumyan E, Gabrielian E, Goukasova G, Wikman G, Wagner H. 2000. Pharmacokinetic and oral bioavailability of andrographolide from Andrographis paniculata fixed combination kan jang in rats and human. Phytomedicine. 7:351–364.
- Panossian A, Wikman G. 2009. Evidence-based efficacy of adaptogens in fatigue, and molecular mechanisms related to their stress-protective activity. Curr Clin Pharmacol. 4:198–219.
- Panossian A, Wikman G. 2013. Efficacy of Andrographis paniculata in upper respiratory tract infectious diseases and the mechanism of action. In: Wagner H, Ulrich-Merzenich G, editors. Evidence and rational based research on Chinese drugs. Vienna: Springer. p. 137–179.
- Parixit B, Bharath C, Rajarajeshwari N, Ganapaty S. 2012. The genus Andrographis – a review. Int J Pharm Sci. 4:1835–1856.
- Pawar VS, Shivakumar H. 2012. A current status of adaptogens: natural remedy to stress. Asian Pac J Trop Dis. 2:S480–S490.
- Pekthong D, Martin H, Abadie C, Bonet A, Heyd B, Mantion G, Richert L. 2008. Differential inhibition of rat and human hepatic cytochrome p450 by Andrographis paniculata extract and andrographolide. J Ethnopharmacol. 115:432–440.
- Radhika P, Annapurna A, Rao SN. 2012. Immunostimulant, cerebroprotective & nootropic activities of Andrographis paniculata leaves extract in normal & type 2 diabetic rats. . Indian J Med Res. 135:636–641.
- Recio MC, Andujar I, Rios JL. 2012. Anti-inflammatory agents from plants: progress and potential. Curr Med Chem. 19:2088–2103.
- Sareer O, Ahad A, Umar S. 2012. Prophylactic and lenitive effects of Andrographis paniculata against common human ailments: an exhaustive and comprehensive reappraisal. J Pharm Res Opin. 2:138–162.
- Saxena RC, Singh R, Kumar P, Yadav SC, Negi MP, Saxena VS, Joshua AJ, Vijayabalaji V, Goudar KS, Venkateshwarlu K, et al. 2010. A randomized double blind placebo controlled clinical evaluation of extract of Andrographis paniculata (KalmCold®) in patients with uncomplicated upper respiratory tract infection. Phytomedicine. 17:178–185.
- Serrano FG, Tapia-Rojas C, Carvajal FJ, Hancke J, Cerpa W, Inestrosa NC. 2014. Andrographolide reduces cognitive impairment in young and mature AβPPswe/PS-1 mice. Mol Neurodegener. 9:1–18.
- Thakur AK, Chatterjee SS, Kumar V. 2014a. Therapeutic potential of traditionally used medicinal plant Andrographis paniculata (Burm. F.) against diabesity: an experimental study in rats. TANG (Humanitas Med). 4:1–8.
- Thakur AK, Chatterjee SS, Kumar V. 2014b. Neuropsychopharmacology of a therapeutically used Andrographis paniculata extract: a preclinical study. Orient Pharm Exp Med. 14:181–191.
- Thakur AK, Chatterjee SS, Kumar V. 2014c. Antidepressant-like activity of Andrographis paniculata in type-2 diabetic rats. Clin Pharmacol Biopharm. S2:1–9.
- Thakur AK, Chatterjee SS, Kumar V. 2015. Adaptogenic potential of andrographolide: an active principle of the king of bitters (Andrographis paniculata). J Tradit Complement Med. 5:42–50.
- Thakur AK, Shakya A, Husain GM, Emerald M, Kumar V. 2014. Gut-microbiota and mental health: current and future perspectives. J Pharmacol Clin Toxicol. 2:1–15.
- Thakur AK, Soni UK, Rai G, Chatterjee SS, Kumar V. 2014. Protective effects of Andrographis paniculata extract and pure andrographolide against chronic stress-triggered pathologies in rats. Cell Mol Neurobiol. 34:1111–1121.
- Wirth R, Bodi N, Maroti G, Bagyanszki M, Talapka P, Fekete E, Bagi Z, Kovacs KL. 2014. Regionally distinct alterations in the composition of the gut microbiota in rats with streptozotocin-induced diabetes. PLoS One. 9:e110440.
- Xia YF, Ye BQ, Li YD, Wang JG, He XJ, Lin X, Yao X, Ma D, Slungaard A, Hebbel RP, et al. 2004. Andrographolide attenuates inflammation by inhibition of NF-kappa B activation through covalent modification of reduced cysteine 62 of p50. J Immunol. 173:4207–4217.
- Xu Y, Marshal RL, Mukkur TKS. 2006. An investigation on the antimicrobial activity of Andrographis paniculata extracts and andrographolide in vitro. Asian J Plant Sci. 5:527–530.
- Ye L, Wang T, Tang L, Liu W, Yang Z, Zhou J, Zheng Z, Cai Z, Hu M, Liu Z. 2011. Poor oral bioavailability of a promising anticancer agent andrographolide is due to extensive metabolism and efflux by p-glycoprotein. J Pharm Sci. 100:5007–5017.
- Zhang Z, Jiang J, Yu P, Zeng X, Larrick JW, Wang Y. 2009. Hypoglycemic and beta cell protective effects of andrographolide analogue for diabetes treatment. J Transl Med. 7:1–13.