Abstract
Context Siolmatra brasiliensis (Cogn.) Baill (Cucurbitaceae) is a climbing plant widely used for the treatment of diabetes mellitus symptoms.
Objective This work evaluates the antidiabetic activity of an extract of S. brasiliensis in streptozotocin-diabetic rats and promotes the phytochemical investigation to isolate the major compounds of the same extract.
Materials and methods Male Wistar rats were divided into normal (N) and diabetic rats (DC) treated with water; diabetic rats treated with 3U insulin (DI) or with 250 (DSb250) or 500 mg/kg (DSb500) of hydroalcoholic extract of the stalks of S. brasiliensis, via oral gavage, for 21 days. Physiological and biochemical parameters classically altered in diabetes were monitored. The triterpenoids were isolated from the ethyl acetate fraction under silica gel column chromatography and Sephadex-LH20 methods and their structures were determined by NMR, HR-ESI-MS and DC analysis.
Results When compared with DC, DSb250 rats showed a reduction in the hyperglycemia (DC: 26.46 ± 0.69 versus DSb250: 19.67 ± 1.06 mmol/L) and glycosuria (DC: 43.02 ± 3.19 versus DSb250: 28.46 ± 2.14 mmol/24 h) and increase in hepatic glycogen (DC: 14.44 ± 1.26 versus DSb250: 22.08 ± 4.26 mg/g). Three known cucurbitacins were isolated from a hydroalcoholic extract of S. brasiliensis, i.e., cayaponosides A1, B4, D, and a new dammarane saponin 3-O-β-d-gentiobiosyl-26-O-β-d-glucopyranosyl-20-hydroxydammar-24-ene. The structures of these compounds were elucidated by spectral data analysis of the natural products and their acetyl derivatives.
Discussion and conclusion The known cucurbitacins and/or the new identified saponin may be related with the antidiabetic activity of S. brasiliensis.
Introduction
Siolmatra brasiliensis (Cogn.) Baill (Cucurbitaceae) is a climbing plant that occurs in the cerrado and pantanal of Mato Grosso, Brazil, known as ‘taiuiá’ or ‘cipó-tauá’. It is widely used as analgesic for toothache, it is considered a purifying and antisyphilitic agent (Pott & Pott Citation1994), its infusion is used to treatment of ulcer (Biesky et al. Citation2012); the plant has been also used as an antidiabetic agent. Cytotoxic studies showed that the ethanolic extract of the stems is toxic in low doses for S180 tumor cells (5 mg/mL) and macrophages (2.5 and 5 mg/mL) (Lima et al. Citation2006). In low doses (10–100 mg/kg), the ethanolic extract of the stems of S. brasiliensis does not show toxicity in mice, but at higher doses (1000–2000 mg/kg) some adverse effects, even mortality, have been observed (Lima et al. Citation2010). There are no studies about the chemical profile of this species and the research about the biological and pharmacological potential is limited. This study was conducted to evaluate the antidiabetic activity of an extract of S. brasiliensis in streptozotocin (STZ)-diabetic rats and to isolate the major compounds.
Materials and methods
General experimental procedures
Plant material
Siolmatra brasiliensis was collected by Libério Amorim Neto and Dr. Amanda Martins Baviera in March 2011, at Jangada, Mato Grosso state, Brazil. The plant material was authenticated by Dr. Vali Joana Pott (Federal University of Mato Grosso do Sul – Department of Biology). A voucher specimen is stored at the herbarium of the Federal University of Mato Grosso do Sul (CGMS: 31.643).
Extraction and isolation
Dried and powdered stalks (6.7 kg) of S. brasiliensis were subjected to extraction with a mixture of EtOH–H2O (7:3) (6 × 6 L). The extract was concentrated under reduced pressure. The crude extract was used for the study of the antidiabetic activity of S. brasiliensis. A part of the crude extract (56 g) was filtered through a silica gel with CHCl3, ethyl acetate (EtOAc) and methanol (MeOH) (isocratic and exhaustive flow). The EtOAc extract (16 g) was applied to a silica gel 60 column (6 cm × 38 cm; 70-230 mesh) with a stepwise elution gradient of EtOAc–MeOH, (10:0) to (0:10). Ninety-five fractions (125 mL) were collected and pooled by TLC similarity to generate eight fractions (A1–A8). Group A4 (1.2 g) was subject to chromatography on a Sephadex LH20 column (4 cm × 39 cm) using dichloromethane–methanol [DCM–MeOH (7:3)] as the mobile phase, affording three groups (A41–A43). A41 (35 mg) was purified by preparative TLC in DCM–MeOH (7:3) to give compound 2 (24 mg). A5 (3.1 g) was fractioned on a Sephadex LH20 column (4 cm × 39 cm) in MeOH; 110 fractions were collected and analysed by TLC to generate two groups (A51 and A52). A51 group (2.1 g) was subjected to a silica gel 60 column (2.5 cm × 22 cm; 70–230 mesh) eluted with a gradient of DCM–MeOH, (10:0) to (0:10). Fifty-seven fractions were collected and analysed by TLC to generate nine fractions (A511–A519). A517 (243 mg) was subjected to a flash chromatography column (3 cm × 24 cm; 230–400 mesh) eluted with a gradient of CHCl3–MeOH, (85:15) to (1:1). Thirty-four fractions were collected and analysed by TLC; fraction 26 was pure, giving compound 3 (13 mg). A52 (900 mg) was subjected to a silica gel 60 column (2.5 cm × 23 cm; 230–400 mesh) eluted in a gradient of DCM–MeOH, (9:1) to (0:10); 80 fractions were collected and analysed by TLC to generate four groups (A521–A524). A524 (20 mg) was purified by preparative TLC to generate compound 4 (12 mg). A7 (2.2 g) was subjected to a silica gel 60 column (3 cm × 22 cm; 70–230 mesh) eluted in a gradient of DCM–MeOH, (6:4) to (4:6). Fifty-four fractions were collected and analysed by TLC to generate four groups (A71–A74). A73 (850 mg) was subjected to flash chromatography using a silica gel 60 column (2 cm × 32 cm; 230–400 mesh) eluted in DCM–MeOH (65:35). Twenty-nine fractions were collected and analysed by TLC. Between fractions 16 and 25, the precipitation of an amorphous white solid occurred, which was washed with MeOH to generate compound 1 (45 mg).
Subchronic evaluation of the antidiabetic activity of S. brasiliensis extract in STZ-diabetic rats
Male Wistar rats (180–210 g, 38–40 days old) were housed in individual metabolic cages, under environmentally controlled conditions for temperature (24 ± 1 °C) and with a 12:12 h light–dark cycle. Rats had free access to water and commercial lab chow (Purina®Labina). All experimental procedures were performed between 08:00 and 10:00 a.m. and were done according to the Brazilian College of Animal Experimentation (COBEA) and received prior institutional approval by the Committee for Ethics in Animal Experimental from UFMT (protocol number 23108.029613/09-3).
STZ (Sigma Aldrich, St. Louis, MO; 40 mg/kg) dissolved in 0.01 mol/L citrate buffer (pH 4.5) was administered through a single intravenous injection in 14 h fasted rats. Normal rats received only citrate buffer, in the same manner. Five days after STZ administration, rats with post-prandial glycemia values of 25–28 mmol/L were used in the experiments to compose the diabetic groups. The rats were divided into five groups (5–8 rats per group): N, normal, non-diabetic group, treated with vehicle (water); DC, diabetic rats treated with water; DSb250, diabetic rats treated with 250 mg/kg b.w. of S. brasiliensis extract; DSb500, diabetic rats treated with 500 mg/kg b.w. of S. brasiliensis extract; DI, diabetic rats treated with 3U insulin/rat. All groups (except DI) received vehicle or freshly prepared extract once a day, by oral gavage, for 21 days. Animals from DI group received 3 U insulin/rat (Insulin NPH, Lilly, s.c.), at 08:00 a.m. and 06:00 p.m., for 21 days.
Body weight, food and water intake and urinary volume were monitored daily. Every 5 days, plasma glucose levels were determined (Bergmeyer et al. Citation1974), as well as the urea (Bernt & Bergmeyer Citation1965) and glucose urinary levels (Summerson et al. Citation1947), in 24 h urine. At the end of the experiment, the rats were euthanised and white adipose tissues (retroperitoneal, perirenal, epididymal), skeletal muscles (soleus, extensor digitorum longus) and livers were removed and weighted. Liver glycogen was extracted with 30% KOH and precipitated with ethanol (Sjörgren et al. Citation1938) and the quantity recovered was measured by the colorimetric phenol–sulphuric acid method (Montgomery Citation1957).
Statistical analysis
Data are expressed as mean ± standard error of mean (SEM). One-way analysis of variance (ANOVA) followed by the Student–Newman–Keuls test was used to compare the temporal inter-group differences. Differences were considered significant at p < 0.05. Statistical analyses were performed using the program GraphPad Instat® 3.05 (GraphPad Software, La Jolla, CA).
Results and discussion
The saponin (1) and the cucurbitacins (2, 3 and 4) () were isolated from the hydroalcoholic extract obtained from the stalks of S. brasiliensis, using the procedure described in the “Extraction and isolation” section.
The analysis of 1H and 13C NMR (BBD and DEPTQ) along with the COSY, HMQC and HMBC spectra of 1 allowed us to identify 48 carbons. The chemical shift values, allied with the 2D data, permitted us to identify 7 methyl groups, (CH3)7, 14 methylene carbons with (CH2)10(CH2–O)4, 21 methynic carbons with (CH)4(CH–O)13(OCHO)3(=CH)1 and 6 quaternary carbons with (C)4(C–O)1(=C–O)1. These data are compatible with three glucosyl unities and a double bond in the dammarane skeleton, which allowed to propose the partial molecular formula (CH3)7(CH2)20(CH)21C6O18. The values of the quasi-molecular ions of 1, detected in the HR-ESI-MS in negative mode, m/z 945.5463 ([M – H]-), and in positive mode m/z 969.5384 ([M + Na]+), are consistent with the proposed molecular formula C48H82O18 (calc. m/z 945.5423 for M − H and m/z 969.5398 for M + Na). The analysis of the HMBC spectrum was used to locate one O-glucosyl at CH2-25 and one O-gentiobiosyl moiety at CH-3. The additional analysis of this spectrum confirmed the location of HO-20 and Δ24,25. The acetylation of 1 (1a) was carried to confirm the number of hydroxyl groups in the saponin structure. Although it was not possible to define the exact number of methyl groups of the formed acetate due to overlap of signals in the 1H (δ 1.98–2.08 ppm) and 13C (δ 20.5–20.7 ppm) spectra. However, it was possible to identify 11 signals of carbonyl groups in the 13C spectrum of the acetyl derivative [δ 169.15, 169.25 (×2), 169.40 (×2), 169.61, 170.16, 170.23, 170.35, 170.59 and 170.64] confirming the acetylation of all hydroxyl groups of the saponin. The HR-ESI-MS of this acetyl derivative in positive mode revealed an ion peak at m/z 1431.3537 ([M + Na]+) consistent with the molecular formula C70H104NaO29 (calc. 1431.65609), confirming the structure with glucose and gentiobiose moieties. These analyses and a comparison with the literature data (Yang et al. Citation1938; Orihara & Furuya Citation1993) permitted to make the complete NMR data assignments of 1 in pyridine-d5 (), and 1a (CDCl3, see the “Experimental” section) which are described for the first time.
Table 1. NMR spectroscopic data of compound 1 (400 MHz, pyridine-d5).
The 13C and 1H NMR spectroscopic data in combination with analysis of the DEPTQ and HSQC spectra, the ESI mass spectrum and comparison with the literature allowed us to identify 2 as the known non-aromatised nor-cucurbitacin cayaponoside A1 (Himeno et al. Citation1993). As well, compounds 3 and 4 were identified by 1H and 13C NMR data, including HMQC and HMBC analysis, and HR-ESI-MS as the known aromatised nor-cucurbitacins cayaponoside B4 and D (Valente et al. Citation1993; Himeno et al. Citation1994), respectively.
The NOE NMR experiments and the CD spectrum analysis of 2 allowed us to define the absolute stereochemistry of these cucurbitacins (2–4). No similar chromophore to dienone 2 was found by circular dichroism analysis, so considering part of chromophore as 1,3-heteronuclear dienes, a positive signal for the Cotton effect () was found according to the proposed structure with allylic axial chirality concepts (Nakanishi et al. Citation1994). However, considering the dienone as a planar chromophore, the quadrant projection rule (DeAngelis & Wildman Citation1969), in addition to the octant rule for carbonyls, can be used to define the disposition of groups according to the relative configuration for the positive Cotton effect at λmax 305 nm (π–π*) for the quadrant rule and the negative Cotton effect at λmax 340 nm () for n–π* from the C-11 carbonyl chromophore. Thus, the structure of 2 with an absolute configuration was established as 3-O-β-d-glucopyranosyl-(8S,9R,10R,13R,14S,16R,17R,20R)-2,11,22-trioxo-16,20-dihydroxy-25-acetyl-29-nor-cucurbita-3,5-diene. The same analysis was performed for structures 3 and 4 using the aromatic quadrant rule; the projections were in agreement with the positive Cotton effect detected in the CD spectrum of both compounds (). These analyses allowed us to define the structures with an absolute configuration as 2-O-β-d-glucopyranosyl-(8S,9R,13R,14S,16R,17R,20R,22R)-3,16,20,22-tetrahydroxy-25-methoxy-11-oxo-29-nor-1,2,3,4,5,10-hexadehydrocucurbit-23-ene and 2-O-β-d-glucopyranosyl-(8S,9R,13R,14S,16R,17R,20R,22R)-3,16,20,22,25-pentahydroxy-11-oxo-29-nor-1,2,3,4,5,10-hexadehydrocucurbit-23-ene.
In the evaluation of the antidiabetic activity of S. brasiliensis extract, it was observed that the administration of STZ in rats was effective at inducing diabetes mellitus (DM), since the DC group showed various impairments related to the disease. DC rats showed hyperglycemia (), which led to increased urinary glucose excretion (); a lower hepatic glycogen content was also observed (). DC rats also showed a reduced body weight gain (), which was corroborated by the reduced weight of various tissues (), reflecting a catabolic profile typical of an insulin-deficient state. The increased rate of amino acid catabolism observed in diabetic rats explained the increase in the urinary urea levels (), which is a consequence of the increased use of α-ketoacids for glucose synthesis (via gluconeogenesis), so an increased amount of amino groups is available for urea production. Finally, throughout the 21 days of the experiment, other classical symptoms of DM were observed in DC rats, such as polyphagia (), polydipsia () and polyuria (), in comparison with the N group. As expected, treatment with insulin reversed all disturbances related to DM, and the profile of the DI group was similar that of the N group.
Figure 3. Glycemia (A; mmol/L), glycosuria (B; mmol/24 h) and urinary urea (C; mmol/24 h) of STZ-diabetic rats treated for 21 days with S. brasiliensis extract. Values are expressed as means ± SEM, n = 5–8 per group. Differences between groups were analysed with one-way ANOVA followed by Student–Newman–Keuls test (p < 0.05). *Differences with N; #differences with DC; Φdifferences with DSb250; ɛdifferences with DSb500.
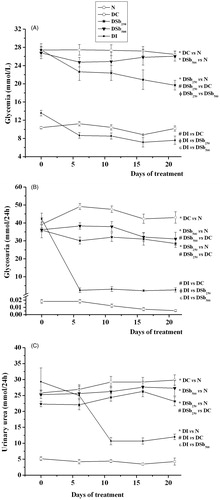
Figure 4. Body weight (A; g), food intake (B; g/24 h), water intake (C; mL/24 h) and urinary volume (D; mL/24 h) of STZ-diabetic rats treated for 21 days with S. brasiliensis extract. Values are expressed as means ± SEM, n = 5–8 per group. Differences between groups were analysed with one-way ANOVA followed by Student–Newman–Keuls test (p < 0.05). *Differences with N; #differences with DC; Φdifferences with DSb250; ɛdifferences with DSb500.
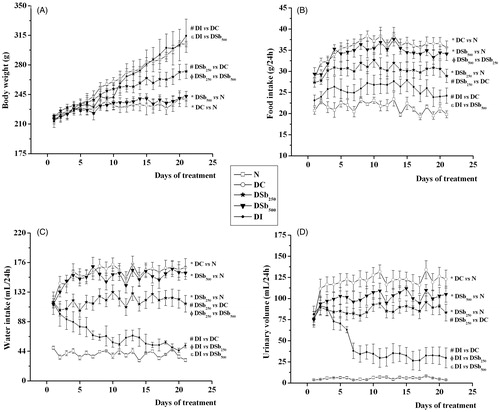
Table 2. Weight of liver, white adipose tissues (retroperitoneal, perirenal, epididymal), skeletal muscles (soleus, extensor digitorum longus, gastrocnemius) and liver glycogen content of STZ-diabetic rats treated for 21 days with S. brasiliensis extract.
The administration of 250 mg/kg of S. brasiliensis extract significantly reduced the glycemia of diabetic rats, from the 16th day of treatment (), and reduced the urinary excretion of glucose () and urea (), in comparison with DC. The liver glycogen content was also increased after S. brasiliensis treatment (). The treatment of diabetic rats with 250 mg/kg of S. brasiliensis extract also improved body weight gain (), which was associated with the increased weight of all studied tissues (). Beneficial effects of the extract were also observed in the food () and water () intake and in the urinary volume (). The antidiabetic activity of many plant species has been attributed to the presence of cucurbitacins (Sharma & Arya Citation2011), which could explain the beneficial effects of S. brasiliensis against DM disturbances. In addition, recent studies have demonstrated that dammarane saponins participate in the antidiabetic activity of some plant species, since they inhibit the activity of the protein tyrosine phosphatase 1B (PTP1B) (Zhang et al. Citation2013; Li et al. Citation2014). Inhibition of PTP1B is considered a promise target to enhance the insulin sensitivity in peripheral tissues (Koren & Fantus Citation2007). The increased glycogen content found in the livers of diabetic rats treated with 250 mg/kg of S. brasiliensis could be related to an increase in the insulin sensitivity of this tissue, contributing to the reduction in glycemia.
It is interesting to note that the highest dose of the S. brasiliensis extract did not improve the disturbances related to DM. It can be suggested that the crude extract possesses compounds that, at higher levels, could interfere with the biological action of those responsible for the antidiabetic activity, in many ways, such as increasing the biotransformation and/or elimination of the active(s) compound(s), impairing its interaction with target tissues and/or even antagonising its beneficial effects.
3-O-β-d-Gentiobiosyl-26-O-β-d-glucopyranosyl-20-hydroxydammar-24-ene (1): Amorphous white solid. MP. 223–228 °C. NMR 1H (400 MHz) and 13C (100 MHz) (). HRESI-MS m/z 969.5384 [M + Na]+ (calc. for C48H82NaO18, 969.53988); HR-ESI-MS m/z 945.5463 [M − H]− (calc. for C48H81O18, 945.54229).
Acetylation of (1): 15 mg of 1 was stirred in Ac2O/pyridine (1:1) overnight at room temperature. The extraction with CHCl3 yielded 12 mg of the peracetyl derivative (1a). HR-ESI-MS m/z 1431.3537 [M + Na]+ (calc. for C70H104NaO29, 1431.6561). 1H NMR (CDCl3, 500 MHz) δ 4.53 (d, 8.2 Hz, H-1′), 4.57 (d, 7.9 Hz, H-1″), 4.45 (d, 8.15, H-1‴), 1.98–2.08 (s, O2C-CH3). 13C NMR (CDCl3, 125 MHz) δ 102.92 (C-1′), 100.61 (C-1″), 98.39 (C-1‴), 169.15-170.64 (O2C–CH3×11), 20.55–2.73 (O2C–CH3). Aglycone moiety: δC/δH, from 1D and 2D spectra: 38.90/1.69 (m)–0.90 (m) (CH2-1); 27.46/1.80 (m)–1.23 (m) (CH2-2); 90.56/3.08 (dd; 4.1, 11.6 Hz) (CH-3); 39.02 (C-4); 56.06/0.73 (m) (CH-5); 18.05/1.53 (m)–1.43 (m) (CH2-6); 31.09/1.08 (m)–1.47 (m) (CH2-7); 40.29 (C-8); 49.73/1.75 (m); 36.75 (C-10); 21.46/1.22 (m)–1.46 (m) (CH2-11); 25.86/1.78 (m)–1.71 (m) (CH2-12); 42.19/1.61 (m) (CH-13); 50.53 (C-14); 35.12/1.49 (m)–1.24 (m) (CH2-15); 16.34/2.12–2.02 (m) (CH2-16); 50.54/1.31 (m) (CH-17); 16.34/0.86 (s) (CH3-18); 16.08/0.72 (s) (CH3-19); 75.16 (C-20); 27.54/0.89 (s) (CH3-21); 40.03/1.60–1.40 (m) (CH2-22); 29.62/1.27–1.24 (m) (CH2-23); 129.96/5.43 (m) (CH-24); 120.22 (C-25); 74.86/3.90 (m) (CH2-26); 13.53/1.61 (s) (CH3-27); 25.20/1.14 (s) (CH3-28); 16.08/0.84 (s) (CH3-29); 15.41/0.94 (s) (CH3-30).
Conclusions
Although these structural types of compounds are common in Cucurbitaceae, new information continues to be disclosed. In addition to a newly identified saponin, analysis of the chiroptical properties (CD) of the three chromophoric cucurbitacins was performed to propose the absolute stereochemistry. The extract of S. brasiliensis showed antidiabetic activity, which may be attributed to the presence of these metabolites.
Declaration of interest
The authors report no declarations of interest. The authors are grateful to Fundação Carlos Chagas Filho de Amparo à Pesquisa do Estado do Rio de Janeiro (FAPERJ), Conselho Nacional de Desenvolvimento Científico e Tecnológico (CNPq), Centro de Pesquisas do Pantanal (CPP), Instituto Nacional de Ciência e Tecnologia em Áreas Úmidas (INAU - MT) and Coordenação de Aperfeiçoamento de Pessoal de Nível Superior (CAPES) for scholarships and financial support.
References
- Bergmeyer HU, Bernt E, Schmidt F, Stork H. 1974. In: Bergmeyer HU, editor. Methods of enzymatic analysis. Weinheim: Verlag Chemie. p. 1196–1201.
- Bernt E, Bergmeyer HU. 1965. Urea. In: Bergmeyer HU, editor. Methods of enzymatic analysis. New York: Academic Press. p. 401–406.
- Biesky IGC, Santos FR, Oliveira RM, Espinosa MM, Macedo M, Albuquerque UP, Martions DTO. 2012. Ethnopharmacology of medicinal plants of the pantanal region (Mato Grosso, Brazil). Evid Based Complem Altern Med. 2012:1–36.
- DeAngelis GG, Wildman WC. 1969. Circular dichroism studies – I: a quadrant rule for the optically active aromatic chromophore in rigid polycyclic systems. Tetrahedron. 25:5099–5112.
- Himeno E, Nagao T, Honda J, Okabe H, Irini N, Nakasumi T. 1993. Structures of new non-aromatized nor-cucurbitacin glucosides in the roots of Cayaponia tayuya. Chem Pharm Bull. 41: 986–988.
- Himeno E, Nagao T, Honda J, Okabe H, Irini N, Nakasumi T. 1994. Studies on the constituents of the root of Cayaponia tayuya (VELL.) COGN. I. Structures of cayaponosides, new 29-nor-1,2,3,4,5,10-hexadehydrocucurbitacin glucosides. Chem Pharm Bull. 42:2295–2300.
- Koren S, Fantus IG. 2007. Inhibition of the protein tyrosine phosphatase PTP1B: potential therapy for obesity, insulin resistance and type-2 diabetes mellitus. Best Pract Res Clin Endocrinol Metab. 21:621–640.
- Li D, Cao J, Bi X, Xia X, Li W, Zhao Y. 2014. New dammarane-type triterpenoids from the leaves of Panax notoginseng and their protein tyrosine phosphatase 1B inhibitory activity. J Ginseng Res. 38:28–33.
- Lima AP, Barbosa CES, Pereira FC, Vilanova-Costa CAST, Ribeiro ASBB, Silva HD, Azavedo NR, Gomes-Klein VL, Silveira-Lacerda EP. 2010. Siolmatra brasiliensis (Cogn.) Baill., Cucurbitaceae, acute toxicity in mice. Rev Bras Farm. 20:917–921.
- Lima AP, Pereira FC, Vilanova-Costa CAST, Ribeiro ASBB, Silveira-Lacerda EP. 2006. Avaliação da atividade antitumoral e citotóxica da planta Siolmatra brasiliensis. Rev Eletr Farm. 3:44–46.
- Montgomery R. 1957. Determination glycogen. Arch Biochem Biophys. 67:378–386.
- Nakanishi K, Berova N, Woody RW. 1994. Circular dichroism principles and applications. Deerfiel Beach (FL): VCH.
- Orihara Y, Furuya T. 1993. Biotransformation of (−)-borneol by cultured cells of Eucalyptus perriniana. Phytochemistry 34:1045–1048.
- Pott A, Pott VJ. 1994. Plantas do pantanal. Brasília: Embrapa.
- Sharma R, Arya VJ. 2011. A review on fruits having anti-diabetic potential. J Chem Pharm Res. 3:204–212.
- Sjörgren B, Nordenskjöld T, Holmgren H, Möllerström J. 1938. Beitrag zur Kenntnis der Leberrhythmik: Glykogen, Phosphor und Calcium in Der Kaninchenleber. Pflugers Arch Eur J Phys. 240:427–448.
- Summerson WH, Hank PB, Oser BL. 1947. Practical physiological chemistry. New York: Blakiston Co.
- Valente LMM, Gunatilaka AAL, Glass TE, Kingston DG, Pinto AC. 1993. New norcucurbitacin and heptanorcucurbitacin glucosides from Fevillea trilobata. J Nat Prod. 56:1772–1778.
- Yang TR, Kasai R, Zhou J, Tanaka O. 1938. Dammarane saponins of leaves and seeds of Panax notoginseng. Phytochemistry 22:1473–1478.
- Zhang XS, Bi XL, Wan X, Cao JQ, Xia XC, Diao YP, Zhao YQ. 2013. Protein tyrosine phosphatase 1B inhibitory effect by dammarane-type triterpenes from hydrolyzate of total Gynostemma pentaphyllum saponins. Bioorg Med Chem Lett. 23:297–300.