Abstract
Context Considering the role of cellular oxidative stress in mutations and subsequent transformation, phytochemicals with antioxidant potential has become a primary choice as chemopreventives. Apodytes dimidiata E. Mey. Ex. Arn (Icacinaceae), a widely used plant in Zulu traditional medicine, is reported to possess antioxidant activity.
Objective To investigate the chemopreventive efficacy of methanol extract of A. dimidiata leaf (AMF).
Materials and methods Antimutagenic potential of AMF (25, 50 and 75 μg/plate) was evaluated by the Ames test. The ability of AMF (100 and 250 mg/kg orally) on restoration of depleted antioxidant status by sodium fluoride (NaF) was analysed on BALB/c mice. 7,12-Dimethylbenz[a]anthracene/croton oil induced mouse skin papilloma model was studied up to 20 weeks to analyse the anticarcinogenic effect of AMF (1%, 3% and 5% topically, twice weekly for 6 weeks). Phytochemicals of AMF were characterized by GC–MS.
Results AMF (75 μg/plate) reverted 4-nitro-o-phenylenediamine (NPDA) induced mutations in Salmonella typhimurium strains, TA 98, 100 and 102 by 74.8%, 72.5% and 69.3%, respectively. Against sodium azide, the percentage reversion was 80.4, 71.3 and 71.3. In mice, AMF (250 mg/kg for 4 days) increased the serum superoxide dismutase (SOD) and catalase activities by 48.71% and 30.3% against the NaF-induced drop. GSH level was improved by 48.59% with a concomitant decrease in TBARS (57.67%). The skin papilloma reduction was 79.32% for 5% AMF. Squalene, dodecanoic, tetradecanoic and hexadecanoic acids are the known antioxidant and chemopreventive molecules identified by GC–MS.
Discussion and conclusion Antioxidant and antimutagenic activities of AMF might have contributed to its anticarcinogenic potential.
Introduction
Constant exposure of carcinogenic chemicals and radiation are considered to be the etiological factors (Gupta & Mukhtar Citation2002) in many malignancies. The generation of free radicals, its exerted oxidative stress on cellular events and subsequent mutations are known to be the crucial mechanisms in carcinogenic process (Clayson et al. Citation1994). Hence, compounds with antioxidant potency could act as revertants of carcinogenesis by blocking the effect of mutagens/carcinogens (Ruan Citation1989) or perhaps preventing the initiation, promotion and progression processes (Edenharder et al. Citation1993). Thus, the chemoprevention strategy focuses on antioxidant, antimutagenic or anticarcinogenic agents to control the development of mutant cell clones (Sporn Citation1993; Kim et al. Citation2001).
Considering the effective scavenging property of free radicals, the phytochemicals has become a primary choice in developing chemopreventive agents. According to Moron and Ames (Citation1983), natural molecules contain factors, which act to lower the mutation rate either by inactivating mutagens or by interfering in the process of mutagenesis. Antioxidant vitamins such as tocopherols inactivate the free radicals and prevent carcinogen formation from inactive precursors and thereby reduce the cancer risk by 20–30% in humans (Hennekens Citation1994). Various plant metabolites such as polyphenols, flavonoids, terpenes, steroids, saponins, alkaloids etc. are found to act as free radical scavengers and also exhibit other bioactivities such as antimutagenic, anticarcinogenic, antimicrobial and anti-inflammatory properties (Yen et al. Citation1993).
Apodytes dimidiata E. Mey. Ex. Arn (Icacinaceae) is a tree species, which is endemic to the Western Ghats of India. In Southern Africa, Zulu people use the root bark of the plant as enema for removing intestinal parasites and the leaves are used for ear inflammation (Watt & Breyer-Brandwijk Citation1962; Hutchings et al. Citation1996). In a phytochemical analysis, it was evidenced that the acetone extract of A. dimidiata contained various phytoconstituents like saponins, tannins, terpenes, steroids and flavonoids and also found to scavenge 2,2-diphenyl-1-picrylhydrazyl (DPPH) free radicals in vitro (Masoko & Nxumalo Citation2013). The plant is also used as a molluscicide for the control of schistosomiasis in rural communities of South Africa and in acute and sub-acute mammal toxicity studies, it was evidenced that A. dimidiata was non-toxic and non-irritating (Brackenbury et al. Citation1997). Six saponins showing antiprotozoal property were elucidated from this plant (Foubert et al. Citation2011). Considering the biological properties reported, the present study investigated the chemopreventive effect of A. dimidiata. Since the free radical mediated mutation is considered to be the prime cause of cancer, the anticarcinogenic potential of A. dimidiata is examined under the limelight of its antioxidant potential against NaF induced oxidative stress and antimutagenic activity against 4-nitro-o-phenylendiamin (NPDA) and sodium azide (NaN3) induced mutagenicity.
Materials and methods
Plant material
The leaves of A. dimidiata used for the study were collected from Periya, Wayanad District of Kerala, India (Altitude: 810 m, Geographical location: Lat. N 11°51′03.19″, Long. 75°48′05.54″) during January 2014 and identified by Dr. Sujanapal P, Taxonomist, Kerala Forest Research Institute (KFRI) Peechi, Thrissur, Kerala. A voucher specimen (No. KFRI 28024) was lodged in the Herbarium of KFRI.
Preparation of extract
Leaves of A. dimidiata were dried under shade and powdered using a mixer grinder. Approximately, 30 g of the powder was extracted separately with 250 mL of petroleum benzene, chloroform, acetone and methanol using Soxhlet apparatus (Kerala, India) for 24 h. The extracts obtained were concentrated to dryness.
Column chromatography analysis
Crude methanol extract (4 g) was loaded on to a 600 mm × 30 mm silica gel (60–120 mesh) column and eluted successively by passing various solvents (150 mL each) of increasing polarities such as petroleum benzene, chloroform, acetone and methanol. The fraction obtained in methanol (AMF), which was found most effective in scavenging free radicals, was used for further studies.
Chemicals
7,12-Dimethylbenz[a]anthracene (DMBA) was purchased from Sigma Chemicals (St. Louis, MO). DMBA was dissolved at a concentration of 100 μg/100 μL acetone. Croton oil was also dissolved in acetone to give a solution of 1% dilution. Nutrient broth was purchased from Hi-media Laboratories, Mumbai, India. Agar agar, l-histidine, biotin, glucose-6-phosphate, dimethyl sulphoxide and NaN3 were obtained from Sisco Research Laboratories, Mumbai, India. NPDA was purchased from Sigma Chemicals. All other chemicals used were of the analytical grade.
Animals
Male BALB/c mice (25–27 g) were purchased from Small Animal Breeding Station, Kerala Veterinary and Animal Sciences University (KVASU), Thrissur, Kerala. They were housed in well ventilated cages under controlled conditions of light and humidity and fed with normal mouse chow (Sai Durga Food and Feeds, Bangalore, India) and water ad libitum. The entire animal experiment in the study was carried out with the prior approval from Institutional Animal Ethics Committee by strictly following the guidelines of Committee for the Purpose of Control and Supervision of Experiments on Animals (CPCSEA) (Reg. No. 149/1999/CPCSEA) constituted by Animal Welfare Division, Government of India.
Bacterial strains
The strains of Salmonella typhimurium, TA 98 and TA 100, were purchased from Microbial Type Tissue Collection and Gene Bank (MTCC), Institute of Microbial Technology Sector, Chandigarh, India in lyophilized forms. TA 102 strain was gifted by Dr. Padma Ambalam, Sourashtra University, Gujarat, India. The strains were sub-cultured in nutrient broth and dispensed in small vials and frozen in presence of 9% DMSO and kept at −70 °C. The bacterial cultures were inoculated in fresh nutrient broth and grown for 12 h at 37 °C before onset of the experiment.
Determination of antimutagenic activity
Anti-mutagenic effect of AMF was analysed using bacterial cultures of S. typhimurium TA 98, TA 100 and TA 102. The strains in a concentration of 1–2 × 109 cells/mL mixed with 2 mL of molten agar containing 0.045 mM histidine/biotin solution. Non-toxic concentrations of AMF were added into minimal agar plates along with the direct acting mutagens such as NaN3 (2.5 μg/plate, dissolved in distilled water) and NPDA (20 μg/plate, dissolved in DMSO). Furthermore, it was spread over minimal glucose agar plates and incubated for 48 h at 37 °C. Revertant colonies formed spontaneously or by various treatments in plates were counted using a colony counter (Garner et al. Citation1972), and antimutagenic effect of AMF was expressed as the difference in the number of colonies between a positive control and AMF-treated plates. Each data are the mean of three plates with standard deviation. The percentage of inhibition of mutagenicity was calculated using the formula [(R1−SR) − (R2−SR)] × 100/(R1−SR), where R1 is the number of revertants in the presence of mutagen alone, R2 is the number of revertants in the presence of drug and SR is the spontaneous revertants (Moron & Ames Citation1983).
In vivo antioxidant property analysis
Male BALB/c mice were divided into eight groups of six each. The first group kept as normal without any treatment. Second, third, fourth and fifth groups were pretreated with propylene glycol (vehicle control), vitamin C (15 mg/kg b wt), AMF (100 mg/kg b wt) and AMF (250 mg/kg b wt), respectively, for 4 days followed by the administration of 600 mg NaF/L in water for 7 days. Sixth group was kept as NaF alone treated control. After treatment, all the animals were sacrificed and blood was collected into the heparinised tubes by cardiac puncture for haematological and other biochemical analysis.
Blood samples were centrifuged at 1000g for 15 min and the upper portion of the centrifuged samples was removed and the packed erythrocytes at the bottom were washed three times with phosphate buffer saline (pH 7.4). A known volume of red blood cells was lysed with hypotonic phosphate buffer. After removing the red blood cell debris by centrifugation of the mixtures (3000g for 15 min), the hemolysates were recovered.
Total WBC and haemoglobin counts were estimated by the method of Chaudhari (Citation2000) and Drabkin and Austin (Citation1932). Superoxide dismutase (SOD) activity was carried out by the method of McCord and Fridovich (Citation1969). Catalase activity in the blood was determined by Aebi (Citation1974) and the reduced glutathione (GSH) by Moron et al. (Citation1979). Lipid peroxidation of hemosylate was measured by the method of Okhawa et al. (Citation1979).
Effect of AMF on DMBA/croton oil induced papilloma in mice
Male Balb/c mice were used for the study. The hairs on the dorsal side (2 cm diameter) of the mice were shaved with rasor at least 2 days before the application of chemicals. Animals were divided into seven groups having six animals each and treatment was as follows. Group I – DMBA + croton oil, group II – DMBA + croton oil + propylene glycol, group III – DMBA + croton oil + 1% AMF, group IV – DMBA + croton oil + 3% AMF, group V – DMBA + croton oil + 5% AMF, group VI – DMBA alone and group VII – croton oil alone. DMBA was applied topically in a concentration of 470 nmol/mouse in 200 μL acetone as single dose. Animals in groups I–VII were applied with 1% croton oil in acetone (200 μL/animal) as well as AMF (200 μL/animal) dissolved in propylene glycol topically 2 weeks after DMBA treatment. Croton oil and drug were applied twice weekly for 6 weeks. AMF and propylene glycol were applied 30 min before each croton oil application. The animals in all groups were examined during the entire period of the study for food intake as well as any toxicity such as weight loss or mortality. Skin papilloma greater than 1 mm diameter size persisted for 2 weeks or more was recorded and included in the cumulative total. Delays in the onset of tumours and number of papilloma per cage in various groups were also recorded (Reddy & Failkow Citation1983). After the experiment, the animals were sacrificed.
GC–MS analysis
AMF was subjected to GC–MS analysis. Chromatographic separation was carried out with the instrument GC-MS-QP 2010 (Shimadzu, Tokyo, Japan) with Db 30.0 column (0.25 μm diameter × 0.25 μm thickness). The oven temperature was programmed from 50°C (isothermal for 5 min), with an increase of 10 °C/min to 200 °C, then 5°C/min to 250 °C, ending with a 35 min isothermal at 250 °C. Mass spectra were taken at 70 eV; a scan interval of 0.5 s and scan range from 40 to 1000 m/z. Helium was used as a carrier gas at 99.999% pressure with flow 1.0 mL/min and electronic pressure control on. Samples were dissolved in methanol and injected automatically (2 μL). Interpretation on GC–MS analysis was done using the database of National Institute Standard and Technology (NIST). The spectrum of the unknown components was compared with the spectrum of known components stored in the NIST library. The name, molecular weight and structure of the components of the test materials were ascertained.
Statistical analysis
The values were expressed as mean ± standard deviation (SD). Statistical evaluation of the data was done by one-way ANOVA followed by Tukey test (post hoc) using Instat 3 software package (San Diego, CA).
Results
Effect of AMF on NaF induced oxidative stress in mice
NaF treatment induced a significant reduction in antioxidant enzymes, SOD (U/g Hb) and catalase (K/g Hb) and GSH (nmol/mL) levels in mice, while the administration of AMF prior to intoxication with NaF significantly prevented the depletion of antioxidant marker levels. NaF intoxication led to significant reduction in GSH level (35.23 ± 9.34) and treatment of AMF (higher dose) elevated the level of GSH to 68.5 ± 10.32 and the depleted level of SOD (302.45 ± 45.98) and catalase (28.56 ± 5.34) was enhanced to 589.75 ± 87.32 and 40.98 ± 6.56 by the administration of AMF. In the NaF-treated animals, the MDA level (nmol/mg protein) was raised to 2.67 ± 0.16 and a significant reduction in lipid peroxidation status was observed as inferred from the value of MDA (1.13 ± 0.25) by the administration of AMF. The effect of AMF on the normalization of these antioxidant markers was comparable to that of vitamin C (standard drug) treated animals (). Counts of WBC and haemoglobin were decreased in NaF alone treated control animals. The count of WBC (cells/mm3) of NaF treated animals (3800 ± 253.56) was significantly increased to 13 000 ± 387.61 by the administration of 250 mg/kg b. wt AMF. Similarly, the reduced haemoglobin level in g/dL (9.12 ± 1.34) was significantly elevated to 14.12 ± 1.34 by the administration of AMF ().
Antimutagenic effect of AMF
The mutagenic effect of NaN3 and NPD in the tester strains TA 98, TA 100 and TA 102 was found inhibited by AMF. The percentage of inhibition of AMF at the concentrations of 25, 50 and 75 μg extract/plate was 66.9, 76.6 and 80.4 with TA 98 strain, 54.2, 68.6 and 71.3, respectively, for TA 100, and 47.2, 69.6, 71.3 for the strain, TA 102, respectively, against sodium azide ().
Table 1. Antimutagenic activity of AMF on mutagenicity induced by sodium azide in S. typhimurium strains TA 98, TA 100 and TA 102.
AMF also showed decrease in the number of colonies of NPDA induced revertant tester strains. The extract was found to be effective in preventing mutagenicity by 61.4%, 73% and 74.8% for TA 98 in the respective concentrations of 25, 50 and 75 μg extract/plate. It was found that for TA 100, the inhibition percentage varied from 51% to 72% for the tested concentrations and for TA 102 the percentage inhibition was observed between 61% and 69%. The antimutagenic activity of the AMF was found to be concentration dependent ().
Table 2. Antimutagenic activity of AMF on mutagenicity induced by NPD in S. typhimurium strains TA 98, TA 100 and TA 102.
Anticarcinogenic effect of AMF on DMBA and croton oil induced papilloma in mice
Topical application of DMBA followed by 1% croton oil twice weekly for 6 weeks resulted in skin papilloma, which started appearing from the fifth week in the control group. The onset of papilloma was found to be significantly delayed or prevented by AMF treatment. In the animals treated with 1% AMF, the papilloma appeared from the eighth week of DMBA application and in 3% AMF group it was found to appear in the ninth week. In 5% AMF treated group, the papilloma formation was considerably reduced () and the onset was delayed up to 11th week (). Out of the total seven animals kept in each group all the animals in the carcinogenic control group developed papilloma (7/7), while only three animals developed papilloma in 5% treated AMF group (3/7). Animals in the normal, DMBA or croton oil alone treated groups did not produce any papilloma on their skin. At the end of 20th week, the average number of papillomas per mouse was found to be 11.28 ± 1.25 and 10.42 ± 0.97 in control and vehicle control groups and this number was significantly reduced to 4.57 ± 0.78, 3.75 ± 0.5 and 2.33 ± 0.57 by 1%, 3% and 5% AMF treatment, respectively (). A reduction of 79.32% in papilloma was observed in 5% AMF treated animals when compared to the vehicle control.
Figure 3. The effect of AMF in the formation of papilloma: (A)- control group (DMBA + croton oil alone) and (B) treated (DMBA + croton oil alone + 5% AMF).
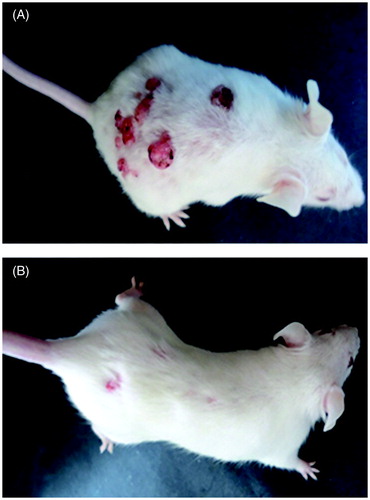
Figure 4. Effect of AMF on the number of papilloma in DMBA induced papillomas bearing mice. Control group indicates DMBA + croton oil alone. Dosage – single dose of DMBA (470 nmol/mouse) in 200 μL acetone. Croton oil (1%) in acetone (200 μL/animal) and 1%, 3% and 5% AMF in propylene glycol, twice in a week for 6 weeks. The number of papillomas per animals was recorded up to 20 weeks.
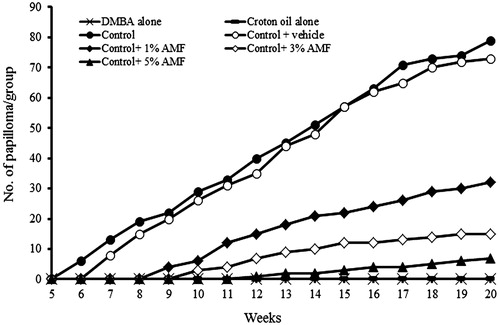
Figure 5. (A) The number of mice with papilloma/group, (B) the average number of papilloma developed/group and (C) the percentage inhibition of papilloma by AMF on DMBA induced papilloma bearing mice. Control group indicates DMBA + croton oil alone. DMBA alone and croton oil alone are not shown as they did not develop papilloma.
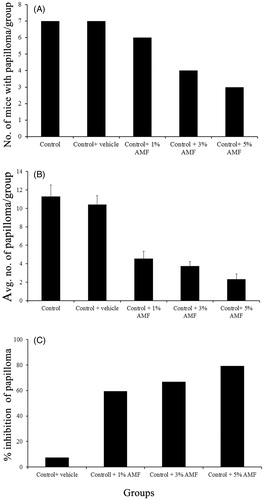
Evaluation of phytoconstituents in AMF by GC–MS analysis
GC–MS analysis revealed as many as 76 constituents in AMF as evidenced from the spectrum (). The name of these compounds with their retention time and area percentage is presented in . Out of this, the active components with known antioxidant and anti-inflammatory properties are presented in along with their compound nature and biological activity.
Table 3. The phytoconstituents identified by GC–MS analysis of AMF.
Table 4. The important phytoconstituents in GC–MS analysis of AMF.
Discussion
DMBA/croton oil induced mouse skin papilloma is a well-known experimental model to understand the anticarcinogenic potency of a test material. In this model, DMBA acts as an initiator and croton oil, which contains the most active phorbol ester, 12-O-tetradecanoyl-phorbol-13-acetate (TPA) promotes further carcinogenic processes. According to Shklar (Citation1999), DMBA and croton oil induced carcinogenic process are mediated by oxidative stress. DMBA, when metabolized to its ultimate carcinogenic form dihydrodiol-epoxide, initiates the production of excessive ROS which causes subsequent DNA damage and inflammation. Repetitive topical application of croton oil (TPA) also leads to the excessive generation of free radicals. This consecutive exposure of free radicals acts as a constant bombardment, which promotes the mutation of codon 61 of the H-ras gene (Bizub Citation1986) leading to the conversion of an initiated cell to a malignant form in mouse skin (Huachen & Krystyna Citation1991).
In the present study, DMBA/croton oil induced papilloma formation in mice was significantly inhibited by the topical application of AMF in a dose-dependent manner. Moreover, the number of mice that developed papilloma was also found to be reduced. The possible reason for this activity of AMF could be explained by the observed antioxidant and antimutagenic properties. In our earlier studies (data not shown) and reports of Masoko and Nxumalo (Citation2013), the free radical scavenging efficacy of this plant has been revealed. The improved activities of antioxidant enzymes and molecules with a concomitant reduction in the lipid peroxidation status of NaF treated mice by AMF administration suggested the strong antioxidant potential of the plant. In the initiation phase, the DMBA induced free radical stress could have been alleviated by the antioxidant action of AMF. Similarly, it is also thought that the AMF might have interfered with the overwhelmed generation of free radicals and subsequent mutagenic events often seen in the croton oil mediated progressive phase. This assumption is made from the fact that AMF inhibited the chemical mutagenicity significantly in various strains of Salmonella in the present study.
Various recent reports state that the natural antioxidants may reduce or inhibit the mutagenic potential of mutagens and carcinogens (Cardador-Martínez et al., Citation2006; El-Sayed & Hussin Citation2013). Zahin et al. (Citation2010) have reported that the anticarcinogenic and antimutagenic activities of medicinal and food plants are executed mainly by scavenging of free radicals. Flavonoids, a class of secondary metabolite, have been shown to exhibit the anticarcinogenic activity (Hirano & Oka Citation1989). In our previous studies, an appreciable amount of flavonoid content was detected in AMF (data not shown).
GC–MS analysis in the present study reveals the presence of various antioxidant and chemopreventive constituents in AMF. For instance, squalene is identified as one of the major compounds in AMF and reported to possess antioxidant (Kohno et al. Citation1995) and chemopreventive activities (Newmark Citation1997; Musakeshavarz & Abdul-Reza Citation2012). Among other identified components, dodecanoic acid (Guler Citation2012) is reported to have antioxidant and antimicrobial activities. Tetradecanoic acid and hexadecanoic acid are reported to be antioxidant, anti-inflammatory and cancer preventive effects (Bodoprost and Rosemeyer Citation2007). Apart from these, a well-known anticancer alkaloid, camptothecin, was isolated from the bark of this plant (Ramesha et al. Citation2013). In our previous studies regarding the anticancer activities of AMF, we have isolated the compound, genipin, as the most cytotoxic component (Divya et al. Citation2015). Genipin is reported to possess antioxidant (Lee et al. Citation2009) and anticancer properties (Cao et al. Citation2010).
It is thought that cancer mostly occurs by the inactivation of tumour suppressor genes resulted from a mutation triggered by ROS (Ramsey & Sharpless Citation2006; Rivlin et al. Citation2011). Most of the medicinal phytoconstituents exert their action through this pathway (Li et al. Citation2012). Thus, the role of an antimutagen and an antioxidant is inevitable in preventing carcinogenesis signifying that any anticarcinogen should essentially possess these properties.
In conclusion, the study indicates that the antioxidant and antimutagenic properties of AMF contributed by its phytoconstituents could have played a major role in the chemopreventive efficacy on skin papilloma formation either by inhibiting DMBA metabolism to its active form or by down-regulating reactive oxygen species formation. Since carcinogenesis is a multifactorial event, it is imperative to develop new drugs with multiple targeting actions. Thus, AMF, the active fraction of A. dimidiata, can serve as a new lead in the identification of a novel drug candidate for the chemoprevention.
Funding information
The authors are thankful to KSCSTE (Kerala State Council for Science Technology and Environment, Kerala, India) for the Research fellowship granted to conduct the work (No 009-26/fshp/09/cste).
Disclosure statement
The authors report no conflicts of interest.
References
- Aebi H. 1974. Catalase. In: Bergmeyer HV, editor. Methods in enzymatic analysis. Vol. 2. New York: Acadamic Press. p. 674–684.
- Bizub D. 1986. Mutagenesis of the Ha-ras oncogene in mouse skin tumors induced by polycyclic aromatic hydrocarbons. Proc Natl Acad Sci USA. 83:6048–6052.
- Bodoprost H, Rosemeyer J. 2007. Analysis of phenacylester derivatives of fatty acids from human skin surface Sebum by RP-HPLC: chromatograpic mobility as a function of physiochemical properties. Intl J Mol Sci. 8:1111–1124.
- Brackenbury TD, Appleton CC, Thurman G. 1997. Mammal toxicity assessment of the plant molluscicide, Apodytes dimidiata (Icacinaceae), in South Africa. Acta Trop. 65:155–162.
- Cao H, Feng Q, Xu W, Li X, Kang Z, Ren Y, Du L. 2010. Genipin induced apoptosis associated with activation of the c-Jun NH2-terminal kinase and p53 protein in HeLa cells. Biol Pharm Bull. 33:1343–1348.
- Cardador-Martínez A, Albores A, Bah MCalderon-Salinas V, Castano-Tostado E, Guevara-Gonzalez R, Shimada-Miyasaka A, Loarca-Pina G. 2006. Relationship among antimutagenic, antioxidant and enzymatic activities of methanolic extract from common beans (Phaseolus vulgaris L.). Plant Foods Hum Nutr. 61:61–168.
- Chaudhari, AR. 2000. Total leukocyte count. In: Chaudhari AR, editor. A text book of practical physiology. Hyderabad, India: PARAS Medical Publisher. p. 104–108.
- Clayson DB, Mehta R, Iverson F. 1994. Oxidative DNA damage – the effects of certain genotoxic and operationally non-genotoxic carcinogens. Mutat Res. 317:25–42.
- Divya MK, Salini S, Chubicka T, Raghavamenon AC, Babu TD. 2015. Evaluation of cytotoxic and anti-tumor properties of Apodytes dimidiata and characterisation of the bioactive component. Planta Med. [Epub ahead of print]. doi: http://dx.doi.org/10.1055/s-0035-1557751.
- Drabkin DL, Austin JM. 1932. Spectrometric studies; spectrometric constants for common hemoglobin derivatives in human, dog and rabbit blood. J Biol Chem. 98:719–733.
- Edenharder R, Van Petersdorf I, Rauscher V. 1993. Antimutagenic effects of flavonoids, chalcones and structurally related compounds on the activity of 2-amino-3-methylimidazol (4,5-f)quinoline (IQ) and other heterocyclic amine mutagens from cooked food. Mutat Res. 287:261–274.
- El-Sayed WM, Hussin WA. 2013. Antimutagenic and antioxidant activity of novel 4-substituted phenyl-2,2′-bichalcophenes and aza-analogs. Drug Des Dev Ther. 7:73–81.
- Foubert K, Cuyckens F, Matheeussen A, Vlietinck A, Apers S, Maes L, Pieters L. 2011. Antiprotozoal and antiangiogenic saponins from Apodytes dimidiata. Phytochemistry. 72:1414–1423.
- Garner RC, Miller EC, Miller JA. 1972. Liver microsomal metabolism of aflatoxin B1 to a reactive derivative toxic to Salmonella typhimurium TA 1530. Cancer Res. 32:2058–2066.
- Guler GO. 2012. Studies on antioxidant properties of the different solvent extracts and fatty acid composition of Hyoscyamus reticulatus. J Food Biochem. 36:532–538.
- Gupta S, Mukhtar H. 2002. Chemoprevention of skin cancer: current status and future prospects. Cancer Metastasis Rev. 21:363–380.
- Hennekens CH. 1994. Antioxidant vitamins and cancer. Am J Med. 97:2–4.
- Hirano T, Oka K. Akiba M. 1989. Antiproliferative effects of synthetic and naturally occurring flavonoids on tumor cells of the human breast carcinoma cell line, ZR-75–1. Res Commun Chem Pathol Pharmacol. 6:69–78.
- Huachen W, Krystyna F. 1991. In vivo formation of oxidized DNA bases in tumor promoter-treated mouse skin. Cancer Res. 51:4443–4449.
- Hutchings A, Scott AH, Lewis G, Cunningham AB. 1996. Zulu medicinal plants: an inventory. Pietermaritzburg: University of Natal Press.
- Kim DH, Yang BK, Jeong SC, Park JB, Cho SP, Das Surajit, Yun JW, Song CH. 2001. Production of a hypoglycemic, extracellular polysaccharide from the submerged culture of the mushroom, Phellinus linteus. Biotechnology Lett. 23:513–517.
- Kohno Y, Egawa Y, Itoh S, Nagaoka S, Takahashi M, Mukai K. 1995. Kinetic study of quenching reaction of singlet oxygen and scavenging reaction of free radical by squalene in n-butanol. Biochim Biophys Acta. 1256:52–56.
- Lee J, Lee D, Jeong C. 2009. Gardenia jasminoides Ellis ethanol extract and its constituents reduce the risks of gastritis and reverse gastric lesions in rats. Food Chem Toxicol. 47:1127–1131.
- Li F, Jiao P, Yao S, Sang H, Qin SC, Zhang W, Zhang YB, Gao LL. 2012. Paris polyphylla Smith extract induces apoptosis and activates cancer suppressor gene connexin26 expression. Asian Pac J Cancer Prev. 13:205–209.
- Masoko P, Nxumalo KM. 2013. Validation of antimycobacterial plants used by traditional healers in three districts of the Limpopo Province (South Africa). Evid Based Complement Alternat Med. 2013:586247.
- McCord JM, Fridovich I. 1969. Superoxide dismutase. An enzymic function for erythrocuprein (hemocuprein). J Biol Chem. 244:6049–6055.
- Moron DM, Ames BN. 1983. Revised methods for the Salmonella mutagenicity test. Mutat Res. 113:173–215.
- Moron MA, De Pierre JW, Manner VB. 1979. Levels of glutathione, glutathione reductase and glutathione S-transferase activities in rat lung and liver. Biochim Biophys Acta. 582:67–68.
- Musakeshavarz A, Abdul-Reza D. 2012. Compositions in the leaves extract, Avicennia marina Forssk. from Bander –e Kamair (Southern Coast of Iran). Am J Sci Res. 45:85–89.
- Newmark HL. 1997. Squalene, olive oil, and cancer risk: a review and hypothesis. Cancer Epidemiol Biomarkers Prev. 6:1101–1103.
- Okhawa H, Oshishi N, Yagi K. 1979. Assay for lipid peroxides in animal tissues by thiobarbituric acid reaction. Anal Biochem. 95:351–358.
- Ramesha BT, Suma HK, Senthilkumar U, Priti V, Ravikanth G, Vasudeva R, Kumar TR, Ganeshaiah KN, Shaanker RU. 2013. New plant sources of the anti-cancer alkaloid, camptothecine from the Icacinaceae taxa, India. Phytomedicine. 20:521–527.
- Ramsey MR, Sharpless NE. 2006. ROS as a tumour suppressor. Nat Cell Biol. 8:1213–1215.
- Reddy AL, Failkow PJ. 1983. Papillomas induced by initiation-promotion differ from those induced by carcinogen alone. Nature. 304:69–71.
- Rivlin N, Brosh R, Oren M, Rotter V. 2011. Mutations in the p53 tumor suppressor gene: important milestones at the various steps of tumorigenesis. Genes Cancer. 2:466–474.
- Ruan C. 1989. Antimutagenic effect of foods and chemoprevention of cancer. Acta Guangxi Med Coll. 1:68–71.
- Shklar G. 1999. Development of experimental oral carcinogenesis and its impact on current oral cancer research. J Dent Res. 78:1768–1772.
- Sporn MB. 1993. Chemoprevention of cancer. Lancet. 342:1211–1213.
- Watt MJ, Breyer-Brandwijk Gerdina M. 1962. The medicinal and poisonous plants of Southern and Eastern Africa. Edinburgh: E.& S. Livingstone Ltd.
- Win DT. 2005. Oleic acid – the anti-breast cancer component in olive oil. AU J Tech. 9:75–78.
- Yen GC, Duh PD, Tsai CL. 1993. Relationship between antioxidant activity and maturity of peanut hulls. J Agric Food Chem. 41:67–70.
- Zahin M, Aqil F, Ahmad I. 2010. Broad spectrum antimutagenic activity of antioxidant active fraction of Punica granatum L. peel extracts. Mutat Res. 703:99–107.