Abstract
Context Melissa officinalis subsp. inodora Bornm. (Lamiaceae) has been used since ancient times in folk medicine against various diseases, but it has not been investigated against protozoa.
Objective To evaluate the activities of M. officinalis against Leishmania braziliensis, Leishmania infantum and Trypanosoma cruzi as well as its cytotoxicity in fibroblast cell line.
Materials and methods The fresh leaves were chopped into 1 cm2 pieces, washed and macerated with 99.9% of ethanol for 72 h at room temperature. Antiparasitic activity of M. officinalis was accessed by direct counting of cells after serial dilution, while the cytotoxicity of M. officinalis was evaluated in fibroblast cell line (NCTC929) by measuring the reduction of resazurin. The test duration was 24 h. High-performance liquid chromatography (HPLC) was used to characterise the extract.
Results The extract at concentrations of 250 and 125 μg/mL inhibited 80.39 and 54.27% of promastigote (LC50 value = 105.78 μg/mL) form of L. infantum, 80.59 and 68.61% of L. brasiliensis (LC50 value = 110.69 μg/mL) and against epimastigote (LC50 value = 245.23 μg/mL) forms of T. cruzi with an inhibition of 54.45 and 22.26%, respectively, was observed. The maximum toxicity was noted at 500 μg/mL with 95.41% (LC50 value = 141.01 μg/mL). The HPLC analysis identified caffeic acid and rutin as the major compounds.
Discussion The inhibition of the parasites is considered clinically relevant (< 500 μg/mL). Rutin and caffeic acids may be responsible for the antiprotozoal effect of the extract.
Conclusion The ethanol extract of M. officinalis can be considered a potential alternative source of natural products with antileishmania and antitrypanosoma activities.
Introduction
Leishmaniasis is a set of complex and multifaceted syndromes caused by several species belonging to the genus Leishmania (Trypanosomatidae), transmitted by insect vectors. This disease affects human beings as well as domestic and wild animals, has wide geographic distribution and is present in all continents except Antarctica (WHO Citation1990; Desjeux Citation1992). In humans, there are three main forms of leishmaniasis: cutaneous, mucocutaneous and visceral. These forms were classified based on clinical criteria and are caused by 15 different species of parasites (Desjeux Citation2004).
A number of substances are being tested as anti-Leishmania chemotherapic drugs, in which the most important consist of inhibiting vital metabolic pathways specific to the parasite. Pentavalent antimonials (Sb5+) have traditionally been the first-line drugs to treat leishmaniasis (Bezerra et al. Citation2004). Other drug groups currently in use against leishmaniasis included amidines, polyenics, aminoglycosides and hexadecylphosphocholine (miltefosine), some of which are known to have toxicity on patients associated with side effects (Bezerra et al. Citation2004). In addition, these compounds are costly and may induce parasite resistance (Croft & Coombs Citation2003; Rath et al. Citation2003).
Another quite common parasitic disease worldwide is the Chagas disease. It is caused by parasitic protozoan Trypanosoma cruzi and represents one of the most serious endemics diseases in Brazil. Of particular public health concern, it affects 16–18 million individuals in the Americas, with 100 million under risk of contamination (Ambrozin et al. Citation2008). The parasite can be transmitted to humans through the faces and urine of vectors (triatomines), infection with T. cruzi through the transfusion of blood products and organ transplants from the infected donors. It can also be transmitted when the infected mother transmits T. cruzi to the foetus during pregnancy or childbirth (congenital or vertical), and when contaminated material comes in contact with open skin or mucosa during manipulation in laboratory settings (accidentally).
In the early 1970s, two compounds namely, nifurtimox (Lampit®) (3-methyl-4-(5-nitro-furfuryladenine) tetrahydro-4H-1,4-thiazine-1,1-dioxide)) and benznidazole (Rochagan®) (N-benzyl-2-nitroimidazol acetamide) were developed for their acute-phase efficiency and tolerance in order to offer new perspectives in the treatment of Chagas disease. Until now, they are still in use, but have limitation in terms of low specificity of action on defined biochemical pathways of the parasite, associated with side effects during treatment (Dias & Dessoy Citation2009).
In general, the treatment of both diseases (Leishmaniasis and Chagas disease) is difficult, particularly due to the resistance of the parasite to available drugs and the toxicity or side effects of the drugs (Ouellette et al. Citation2004; Castro et al. Citation2006). The latter issue is justified mainly by the fact that because they are eukaryotes (i.e., the parasites), they share several characteristics with mammalian cells. Thus, the action of antiparasitic agents occurs in pathways or targets common to the parasite and host (Murray et al. Citation2000). Given the difficulty in finding therapeutic drugs that are effective and non-toxic to human beings, it becomes extremely important to search for new chemotherapy agents with no or reduced toxicity (Calixto Citation2000; Rocha et al. Citation2005).
In this context, a very promising alternative is the use of plant extracts, which have been used since the dawn of civilisation in the cure process for countless diseases (Calixto Citation2000; Rocha et al. Citation2005). Several studies have been carried out to evaluate the effect of the use of plant extracts or their metabolites in the prevention and/or treatment of protozoan infection (Rodríguez et al. Citation2008; Rojas et al. Citation2010; Morais-Braga et al. Citation2013; Figueredo et al. Citation2014; Tasdemir et al. Citation2006). For instance, secondary metabolites such as quinolones, alkaloids, monoterpenes, sesquiterpenes, diterpenes, triterpenes, saponins, chalcones and flavonoids were reported to exhibit anti-Leishmania activity (Chan-Bacab et al. Citation2001). Similarly, diterpenes and flavonoids were reported to possess anti-Trypanosoma activity (Tasdemir et al. Citation2006; Ambrosio et al. Citation2008).
Melissa officinalis subsp. inodora Bornm. (Lamiaceae), commonly known as “lemon balm”, is a perennial herb with multiple uses in the folk medicine. In traditional medicine, it is used in the treatment of headache, migraine, toothache, earache, flatulence, indigestion, cramping, nausea, anxiety anaemia, vertigo, syncope, asthma, bronchitis, amenorrhea, heart failure, arrhythmias, insomnia, epilepsy, depression, psychosis, hysteria, stomach disorders, ulcers, wounds, rheumatisms and torticollis (Carnat et al. Citation1998; Herodez et al. Citation2003; Salah & Jäger Citation2005; Dastmalchi et al. Citation2007). Pharmacological reports indicate that M. officinalis exhibit a variety of actions including antioxidant (Kamdem et al. Citation2013) and anticholinesterase activities (Pereira et al. Citation2009).
Based on the aforementioned considerations, the present study was undertaken to evaluate for the first time the anti-Leishmania and anti-Trypanosoma activities of ethanol extract from the leaves of M. officinalis on promastigote forms of parasites Leishmania braziliensis, Leishmania infantum and epimastigote forms of T. cruzi, which are responsible for clinical manifestation of leishmaniasis and trypanosomiasis, respectively. In addition, the cytotoxicity of the plant extract was investigated in fibroblasts.
Materials and methods
Plant material
Melissa officinalis leaves were collected from the Crato Seedling Production Bank, located at 07°14′S and 39°24′W in May 2014. A specimen was deposited at the Dárdano de Andrade Lima Herbarium of the Regional University of Cariri URCA and identified by Dr. Maria Arlene Pessoa da Silva.
Preparation of the extract
The fresh leaves were chopped into 1 cm2 pieces, washed and macerated with 99.9% of ethanol for 72 h at room temperature. Next, the extract was filtered and concentrated using a rotary evaporator (model Q-344B, Quimis, Diadema, Brazil) to evaporate the ethanol. Dehydration took place in water bath (model Q214M2, Quimis, Diadema, Brazil). Once concentrated, the extract was frozen at −20 °C, freeze-dried, placed in an amber glass jar and stored in a freezer and lyophilised (Matos Citation2009).
Chemicals, apparatus and general procedures
All chemicals were of analytical grade. Ethanol, formic acid, gallic acid, caffeic acid, chlorogenic acid, rosmarinic acid and ellagic acid were purchased from Merck (Darmstadt, Germany). Catechin, epicatechin, isoquercitrin, quercetin, quercitrin, kaempferol and rutin were acquired from Sigma Chemical Co. (St. Louis, MO). High-performance liquid chromatography (HPLC-DAD) was performed with a Shimadzu Prominence Auto Sampler (SIL-20A) HPLC system (Shimadzu, Kyoto, Japan), equipped with Shimadzu LC-20AT reciprocating pumps connected to a DGU 20A5 degasser with a CBM 20A integrator, SPD-M20A diode array detector and LC solution 1.22 SP1 software.
Quantification of compounds by HPLC-DAD
Reverse phase chromatographic analyses were carried out under gradient conditions using C18 column (4.6 mm × 150 mm) packed with 5 μm diameter particles; the mobile phase was water containing 1% formic acid (A) and ethanol (B), and the composition gradient was 13% of B until 10 min and changed to obtain 20, 30, 50, 60, 70, 20 and 10 B at 20, 30, 40, 50, 60, 70 and 80 min, respectively, following the method described by Abbas et al. (Citation2014) with slight modifications. Melissa officinalis extract and mobile phase were filtered through 0.45 μm membrane filter (millipore) and then degassed by ultrasonic bath prior to use. Melissa officinalis extract was analysed at a concentration of 5 mg/mL. The flow rate was 0.7 mL/min, injection volume 50 μL and the wavelength were 254 nm for gallic acid, 280 nm for catechin and epicatechin; 325 nm for chlorogenic, caffeic, rosmarinic and ellagic acids, and 365 nm for quercetin, isoquercitrin, quercitrin, kaempferol and rutin. Stock solutions of standards references were prepared in the HPLC mobile phase at a concentration range of 0.030–0.250 mg/mL for quercetin, isoquercitrin, quercitrin, rutin, kaempferol, epicatechin and catechin; and 0.050–0.400 mg/mL for ellagic, caffeic, gallic, rosmarinic and chlorogenic acids. Chromatography peaks were confirmed by comparing its retention time with those of reference standards and by DAD spectra (200–600 nm). All chromatography operations were carried out at ambient temperature and in triplicate. The limit of detection (LOD) and the limit of quantification (LOQ) were calculated based on the standard deviation of the responses and the slope using three independent analytical curves. LOD and LOQ were calculated as 3.3 and 10 σ/S, respectively, where σ is the standard deviation of the response and S is the slope of the calibration curve (Boligon et al. Citation2013).
Antiparasitic activity
Cell lines used
For in vitro studies with T. cruzi, the clone CL-B5 was used according to the method described by Le Senne et al. (Citation2002). Parasites were stably transfected with the Escherichia coli β-galactosidase gene (lacZ). Epimastigotes were grown at 28 °C in liver infusion tryptose broth (Difco, Detroit, MI) with 10% foetal bovine serum (FBS) (Gibco, Carlsbad, CA), penicillin (Ern, S.A., Barcelona, Spain) and streptomycin (Reig Jofré S.A., Barcelona, Spain), and harvested during the exponential growth phase (Roldós et al. Citation2008). Test duration was of 24 h. Culture of L. brasiliensis and L. infantum were obtained from the Instituto de Investigaciones en Ciencias de la Salud, Asunción, Paraguay – IICS. The maintenance of strain, the form of cultivation and the isolation of shape promastigote were made according to the procedure of Roldós et al. (Citation2008). The inhibition assays of promastigotes were performed using the strain of L. braziliensis (MHOM/BR/75/M2903), grown at 22 °C in Schneider’s Drosophila melanogaster medium supplemented with 20% FBS. For the cytotoxic assays, the fibroblast cell line NCTC929, grown in Minimal Essential Medium (Sigma, St. Louis, MO), was used. The culture medium was supplemented with heat-inactivated FBS (10%), penicillin G (100 U/mL) and streptomycin (100 μg/mL). Cultures were maintained at 37 °C in humid atmosphere with 5% CO2. The viability of these strains was assessed according to Roldós et al. (Citation2008), through the use of resazurin as a colorimetric method.
Reagents
Resazurin sodium salt was obtained from Sigma-Aldrich (St. Louis, MO) and stored at 4 °C protected from light. A solution of resazurin was prepared in 1% phosphate buffer, pH 7, filtered and sterilised prior to use. Chlorophenol red-β-d-galactopyranoside (CPRG; Roche, Indianapolis, IN) was dissolved in 0.9% Triton X-100 (pH 7.4). Penicillin G (Ern, S.A., Barcelona, Spain), streptomycin (Reig Jofré S.A., Barcelona, Spain) and dimethylsulphate were also used. Liver infusion tryptose broth (Difco, Detroit, MI) was used.
In vitro epimastigote susceptibility assay
The screening assay was performed in 96-well microplates with cultures that had not reached the stationary phase (Vega et al. Citation2005). Briefly, epimastigotes were seeded at 105 mL − 1 in 200 μL of liver tryptose broth medium. The plates were then incubated with the drugs (0.1–50 μg/mL) at 28 °C for 72 h, and 50 μL of CPRG solution was added to give a final concentration of 200 μM. The plates were incubated at 37 °C for an additional 6 h and were then read at 595 nm. Each experiment was performed three-times and independently, each concentration was tested in triplicate in each experiment. The efficacy of each compound was estimated by calculating the anti-epimastigotes and the result was expressed in percent inhibition.
In vitro leishmanicidal assay
Cultures of promastigotes of L. brasiliensis and L. infantum were grown to a concentration of 106 cells/mL and then transferred to the test. The compounds were dissolved in DMSO to the concentrations to be tested and were transferred to microplates. The activity of compounds was evaluated after 72 h by direct counting of cells after serial dilutions and compared with an untreated control.
Cytotoxic assays
NCTC929 fibroblasts were placed in 96-well microplates at a final concentration of 104 cells/well. The cells were grown at 37 °C in an atmosphere of 5% CO2. After that, the culture medium was removed and the compounds were added to 200 μL, and performed a new culture for 24 h. After this incubation, 20 μL of a 2 mM solution of resazurin was added to each well. The plates were incubated for 3 h and the reduction of resazurin was measured at 490 and 595 nm against blank control.
Statistical analysis
The statistical analysis of LC50 values are expressed as mean ± SD, using software Probit TSK version 1.5 (SPSS Inc., Chicago, IL).
Results and discussion
Melissa officinalis at concentrations of 250 and 125 μg/mL inhibited, respectively, 80.39 and 54.27% of promastigote form of L. infantum. Similar inhibition was observed at the same concentrations, against L. brasiliensis. However, 250 and 125 μg/mL of M. officinalis caused lower inhibition against epimastigote forms of T. cruzi, with 54.45 and 27.26%, respectively (). As depicted in , M. officinalis showed increased toxicity with increasing concentration. However, the maximum toxicity was noted at 500 μg/mL with 95.41%.
Table 1. Leishmanicide and trypanocide activities of Melissa officinalis.
Table 2. Cytotoxic activity of Melissa officinalis.
HPLC fingerprinting of M. officinalis ethanol extract revealed the presence of the gallic acid (tR = 1.37 min; peak 1), catechin (tR = 14.96 min; peak 2), chlorogenic acid (tR = 21.09 min; peak 3), caffeic acid (tR = 24.73 min; peak 4), rosmarinic acid (tR = 27.18; peak 5), ellagic acid (tR = 32.51 min; peak 6), epicatechin (tR = 38.26 min; peak 7), rutin (tR = 41.62 min; peak 8), isoquercitrin (tR = 45.03 min; peak 9), quercitrin (tR = 47.38 min; peak 10), quercetin (tR = 51.84 min; peak 11) and kaempferol (tR = 55.04 min; peak 12) ( and ). Calibration curve for gallic acid: Y = 130 845× + 1267.3 (r = 0.9997); chlorogenic acid: Y = 12 761× + 1198.5 (r = 0.9999); caffeic acid: Y = 12 621× + 1367.1 (r = 0.9995); ellagic acid: Y = 13 146× + 1264.1 (r = 0.9994); catechin: Y = 11 984× + 1257.5 (r = 0.9993); epicatechin Y = 12 743× + 1186.9 (r = 0.9996); rosmarinic acid: Y = 11 978× + 1347.2 (r = 0.9995); isoquercitrin: Y = 18 874× + 1350.6 (r = 0.9999); quercitrin: Y = 12 836× + 1205.8 (r = 0.9999); rutin: Y = 13 058× + 1173.9 (r = 0.9998); quercetin: Y = 13 627× + 1292.5 (r = 0.9996) and kaempferol: Y = 13 548× + 1246.5 (r = 0.9995).
Figure 1. Representative high-performance liquid chromatography profile of Melissa officinalis. Gallic acid (peak 1), catechin (peak 2), chlorogenic acid (peak 3), caffeic acid (peak 4), rosmarinic acid (peak 5), ellagic acid (peak 6), epicatechin (peak 7), rutin (peak 8), isoquercitrin (peak 9), quercitrin (peak 10), quercetin (peak 11) and kaempferol (12). TR, retention time.
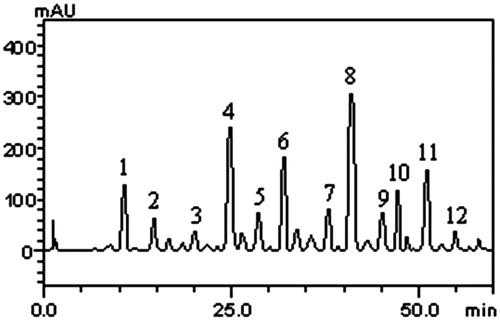
Table 3. Phenolics and flavonoids composition of Melissa officinalis leaves extract.
Based on our results, it appears that rutin was the most prevalent flavonoid with 9.26%, followed by quercetin (4.13%), quercitrin (3.59%) and isoquercitrin (2.35%). With regard to phenolic acids, the most prevalent was caffeic acid with 6.42%, followed by ellagic acid (4.85%) and gallic acid (3.76%). In HPLC analysis of study performed by Dastmalchi et al. (Citation2007), caffeic acid, eriodictyol-7-O-glucoside, m-coumaric acid, naringin, hesperidin, rosmarinic acid, naringenin and hesperetin were identified. From the compounds identified, rosmarinic and caffeic acids have already been reported to be present in the plant by other researchers (Carnat et al. Citation1998; Ziaková & Brandšteterova Citation2002, Citation2003; Zgórka & Glowniak Citation2006).
The scientific evaluation of medicinal plants used in the preparation of folk remedies has provided modern medicine with effective pharmaceuticals for the treatment of diseases caused by protozoan parasite (Phillipson & Wright Citation1991; Chan-Bacab et al. Citation2001). Some of the metabolites obtained from plants used in the treatment of diseases caused by protozoan parasites include quinones, phenolics, flavonoids, alkaloids and terpenes (Chan-Bacab et al. Citation2001).
Most of the studies directed towards the detection of plant secondary metabolites with leishmanicidal activity have been done using the promastigote form of the parasite because it is easier to maintain under in vitro conditions (Chan-Bacab et al. Citation2001; Lewin et al. Citation2011; Figueredo et al. Citation2014). However, since the promastigote is not the infective form of the parasite in vertebrate hosts, evaluations done with promastigotes have only an indicative value of the possible leishmanicidal activity of the metabolite tested (Chan-Bacab et al. Citation2001). As a result of this, a preliminary evaluation using promastigotes must be complemented with an evaluation using intracellular amastigotes in macrophages (Chan-Bacab et al. Citation2001; Sobral-Souza et al. Citation2014).
As for the study with T. cruzi, several reports indicate that activity on epimastigote forms of T. cruzi can also be a indicative pathway for an effective choice of anti-Trypanosoma substances, considering that in vitro studies can reduce the number of animals used in experiments with trypomastigote and amastigote forms (Leite et al. Citation2013; Morais-Braga et al. Citation2013).
This study detected that the anti-promastigote activity of L. infantum and L. brasiliensis offered ≥ 54.27% inhibition at concentrations of 125 and 250 μg/mL, respectively. This can be considered significant, given that inhibition at concentrations ≤ 500 μg/mL, is regarded as clinically relevant. Anti-protozoan activity against the epimastigote form of T. cruzi was identified as moderate (Rosas et al. Citation2007).
This is the first study on anti-Leishmania activity of the extracts from this species, but there have been studies on the activity against this protozoan by the essential oil of M. officinalis (Mikus et al. Citation2000). Nevertheless, there are studies demonstrating the anti-Leishmania activity of flavonoids and isolated phenolic compounds; among them include those found in the leaves extract of M. officinalis (Lewin et al. Citation2011). With regard to flavonoids, one study shows the activity of Rutin with IC50 value > 30 μg/mL against the amastigote form of L. donovani; this substance was the major secondary metabolites/component from the group of flavonoid identified in the plant extract. In the same line, caffeic acid which was the major component of the phenolic acids showed an IC50 value of 5.6 μg/mL against the amastigote form of L. donovani (Tasdemir et al. Citation2006).
With regard to anti-Trypanosoma activity, the search for new alternative medications for the treatment of Chagas disease has brought plant products to the forefront, as they proved to be a significant pathway against hemoflagellate parasites (Otoguro et al. Citation2011). This is the first study on the anti-Trypanosoma activity of M. officinalis. Nevertheless, studies exist on the anti-Trypanosoma activity of flavonoids and isolated phenolic compounds in this species, which relate this activity to the majority components of the extract from the leaves of M. officinalis (Tasdemir et al. Citation2006). With regard to flavonoids, the same study shows activity of Rutin IC50 value >30 μg/mL, against trypomastigote forms of T. cruzi, while another study shows activity of the caffeic acid phenolic compound of IC50 value >30 μg/mL (Tasdemir et al. Citation2006).
There is one study demonstrating the activity of kaempferol, quercitrin, quercetin and gallic acid against L. donovani and T. cruzi (Tasdemir et al. Citation2006). The results obtained in this study corroborate with those of Tasdemir et al. (Citation2006), suggesting that the anti-Leishmania and anti-Trypanosoma activities of M. officinalis may be partly attributed to the presence of caffeic acid and rutin.
One of the possible modes of action of antiprotozoal flavonoids is the effect on the generation of reactive oxygen species (Ribeiro et al. Citation1997). Other biochemical mechanisms include the inhibition of the p-glycoprotein-like transporter (Perez-Victoria et al. Citation1999) or modulation of protein phosphorylation on the SPK89 protein kinase in trypanosomes (Gale et al. Citation1994). Corroborating with what was previously mentioned; a more recent study showed that quercetin has a pro-oxidant effect, as it acted to produce reactive oxygen species, against the amastigote forms of Leishmania amazonensis, leading to dysfunction of its mitochondrial membrane (Fonseca-Silva et al. Citation2013).
The in vitro evaluation of the toxicity of active substances is one of the first steps for the use of these compounds in animal models (Dias & Dessoy Citation2009). In most cases, the results are not extrapolated directly to the animals, because if a product causes damage to cells in vitro, it may also affect animals exposed (Carvalho Citation1996). Different products have been tested to evaluate the cytotoxic potential, allowing the possibility of discovering new drug candidates, whose side effects are lower. In this study, toxicity was low at the concentration of 125 μg/mL but was high at the other concentrations and LC50 value of 141.01 μg/mL considered high, however, the fractionation of their compounds can reduce the toxicity (Santos et al. Citation2012). Only one study exists on the toxicity of this species, demonstrating low genotoxicity and cytotoxicity of the ethanol extract of M. officinalis in human leukocytes. The authors demonstrated that M. officinalis was neither genotoxic nor cytotoxic to human leukocytes (Kamdem et al. Citation2013).
Conclusion
The ethanol extract from the leaves of M. officinalis can be regarded as a potential source of natural product featuring anti-Leishmania and anti-Trypanosoma activity. However, due to its high toxicity, new trials as well as in vivo tests are necessary to evaluate the anti-protozoan potential of the isolated compounds, in order to identify their efficacy and toxicity.
Acknowledgements
The authors are particularly grateful to Dr. F. Buckner from the Instituto Conmemorativo Gorgas (Panama) for providing the parasites. Dr. Kamdem thanks CAPES, TWAS, and CNPq for financial support.
Disclosure statement
The authors report no conflicts of interest. The authors alone are responsible for the content and writing of this article.
References
- Abbas SR, Sabir SM, Ahmad SD, Boligon AA, Athayde ML. 2014. Phenolic profile, antioxidant potential and DNA damage protecting activity of sugarcane (Saccharum officinarum). Food Chem. 147:10–16.
- Ambrosio SR, Arakawa NS, Esperandim VR, de Albuquerque S, Da Costa FB. 2008. Trypanocidal activity of pimarane diterpenes from Viguiera arenaria (Asteraceae). Phytother Res. 22:1413–1415.
- Ambrozin ARP, Vieira PC, Fernandes JB, Graças MF, Silva F. 2008. Piranoflavonas inéditas e atividades tripanocidas das substâncias isoladas de Conchocarpus heterophyllus. Quim Nova. 31:740–743.
- Bezerra RJS, Leon L, Genestra M. 2004. Recentes avanços da quimioterapia das leishmanioses: moléculas intracelulares como alvo de fármacos. Rev Bras Ciênc Farm. 40:141–148.
- Boligon AA, Kubiça TF, Mario DN, Brum TF, Piana M, Weiblen R, Lovato L, Alves SH, Santos RCV, Alves CFS, et al. 2013. Antimicrobial and antiviral activity-guided fractionation from Scutia buxifolia Reissek extracts. Acta Physiol Plant. 35:2229–2239.
- Calixto JB. 2000. Efficacy, safety, quality control, marketing and regulatory guidelines for herbal medicines (phytotherapeutic agents). Braz J Med Biol Res. 33:179–189.
- Carnat AP, Carnat A, Fraisse D, Lamaison JL. 1998. The aromatic and polyphenolic composition of lemon balm (Melissa officinalis L. subsp. officinalis) tea. Pharm Acta Helv. 72:301–305.
- Carvalho TU. 1996. Culturade Células Animais. In: Benchimol M, editor. Métodos de estudo da célula. Rio de Janeiro: FENORTE/UENF.
- Castro JA, Mecca MM, Bartel LC. 2006. Toxic side effects of drugs used to treat Chagas' disease (American trypanosomiasis). Hum Exp Toxicol. 25:471–479.
- Chan-Bacab MJ, Peña-Rodríguez LM. 2001. Plant natural products with leishmanicidal activity. Nat Prod Rep. 18:674–688.
- Croft S, Coombs G. 2003. Leishmaniasis – current chemotherapy and recent advances in the search for novel drugs. Trends Parasitol. 19:502–508.
- Dastmalchi K, Dorman HJD, Oinonen PP, Darwis Y, Laakso I, Hiltunen R. 2007. Chemical composition and in vitro antioxidative activity of a lemon balm (Melissa officinalis L.) extract. LWT – Food Sci Technol. 41:391–400.
- Desjeux P. 1992. Human leishmaniases: epidemiology and public health aspects. World Health Stat Q. 45:267–275.
- Desjeux P. 2004. Leishmaniasis: current situation and new perspectives. Comp Immunol Microbiol Infect Dis. 27:305–318.
- Dias LC, Dessoy MA. 2009. Chemotherapy of Chagas’ disease: state of the art and perspectives for the development of new drugs [in Portuguese]. Quim Nova 32:2444–2457.
- Figueredo FG, Tintino SR, de Brito DIV, Braga MFBM, Leite NF, Lucena BFF, Sobral-Souza CE, Gomez MCV, Coutinho HDM. 2014. Avaliação das potenciais atividades tripanocida e antileishmania do extrato de folhas de Piper arboreum (Piperaceae) e de suas frações. Rev Ciênc Farm Básica Apl. 35:149–154.
- Fonseca-Silva F, Inacio JD, Canto-Cavalheiro MM, Almeida-Amaral EE. 2013. Reactive oxygen species production by quercetin causes the death of Leishmania amazonensis intracellular amastigotes. J Nat Prod. 76:1505–1508.
- Gale M JrJr, Carter V, Parsons M. 1994. Cell cycle-specific induction of an 89 kDa serine/threonine protein kinase activity in Trypanosoma brucei. J Cell Sci. 107:1825–1832.
- Herodez SS, Hadolin M, Skerget M, Knez Z. 2003. Solvent extraction study of antioxidants from balm (Melissa officinalis L.) leaves. Food Chem. 80:275–282.
- Kamdem JP, Adekunle A, Boligon AA, Klimaczewski CV, Elekofehinti OO, Hassan W, Ibrahim M, Waczuk EP, Meinerz DF, Athayde ML. 2013. Antioxidant, genotoxity and cytotoxicity evaluation of lemon balm (Melissa officinalis L.) ethanol extract: its potential role in neuroprotection. Ind Crop Prod. 52:26–34.
- Le Senne A, Muelas-Serrano S, Fernandez-Portillo C, Escario JA, Gómez-Barrio A. 2002. Biological characterization of a beta-galactosidase expressing clone of Trypanosoma cruzi CL strain. Mem Inst Oswaldo Cruz. 97:1101–1105.
- Leite NF, Sobral-Souza CE, Albuquerque RS, Brito DI, Lavor AK, Alencar LB, Coutinho HDM. 2013. Actividad antiparasitaria in vitro citotóxica de cariofileno y eugenol contra Trypanosoma cruzi y Leishmania brasiliensis. Rev Cubana Plantas Med. 18:522–528.
- Lewin G, Cojean S, Gupta S, Verma A, Puri SK, Loiseau PM. 2011. In vitro antileishmanial properties of new flavonoids against Leishmania donovani. Biomed Prev Nutr. 1:168–171.
- Morais-Braga MFB, Souza TM, Santos KKA, Guedes GMM, Andrade JC, Vega C, Rolón M, Costa JG, Saraiva AA, Coutinho HD. 2013. Phenol composition, cytotoxic and anti-kinetoplastidae activities of Lygodium venustum SW. (Lygodiaceae). Exp Parasitol. 134:178–182.
- Matos FJA. 2009. Introdução a fitoquímica experimental. Fortaleza: UFC.
- Mikus J, Harkenthal M, Steverding D, Reichling J. 2000. In vitro effect of essential oils and isolated mono-and sesquiterpenes on Leishmania major and Trypanosoma brucei. Planta Med. 66:366–368.
- Murray PR, Rosenthal KS, Kobayashi GS, Pfaller MA. 2000. Microbiologia médica. Rio de Janeiro: Guanabara Koogan.
- Otoguro K, Iwatsuki M, Ishiyama A, Namatame M, Nishihara-Tukashima A, Kiyohara H, Hashimoto T, Asakawa Y, Omura S, Yamada H. 2011. In vitro anti-trypanosomal activity of plant terpenes against Trypanosoma brucei. Phytochemistry 70:2024–2030.
- Ouellette M, Drummelsmith J, Papadopoulou B. 2004. Leishmaniasis: drugs in the clinic, resistance and new developments. Drug Resist Updat. 7:257–266.
- Pereira KS, Schimidt FL, Guaraldo AMA, Franco RMB, Dias VL, Passos LAC. 2009. Chagas disease as a foodborne illness. J Food Protect. 72:441–446.
- Perez-Victoria JM, Chiquero MJ, Conseil G, Dayan G, Di Pietro A, Barron D, Castanys S, Gamarro F. 1999. Correlation between the affinity of flavonoids binding to the cytosolic site of Leishmania tropica multidrug transporter and their efficiency to revert parasite resistance to daunomycin. Biochemistry 38:1736–1743.
- Phillipson JD, Wright CW. 1991. Antiprotozoal agents from plant sources. Planta Med. 57:53–59.
- Rath S, Trivelin LA, Imbrunito TR, Tomazela DM, de Jesus MN, Marzal PC, Andrade Jr HF, Tempone AG. 2003. Antimoniais empregados no tratamento da leishmaniose: estado da arte. Quim Nova 26:550–553.
- Ribeiro A, Piló-Veloso D, Romanha AJ, Zani CL. 1997. Trypanocidal flavonoids from Trixisvauthieri. J Nat Prod. 60:836–838.
- Rocha LG, Almeida JRGS, Macêdo RO, Barbosa-Filho JM. 2005. A review of natural products with antileishmanial activity. Phytomedicine 12:514–535.
- Rodríguez AM, Camargo JR, García FJB. 2008. Actividad in vitro de la mezcla de alcaloides de Ervatamiacoronaria (Jacq) Staff. Apocynaceae sobre amastigotes de Leishmaniabraziliensis. Rev Bras Farmacogn. 18:350–355.
- Rojas J, Solís H, Palacios O. 2010. Evaluación in vitro de la actividad anti Trypanosoma cruzi de aceites esenciales de diez plantas medicinales. Anales Facul Med. 71:161–165.
- Roldós V, Nakayama H, Rolón M, Montero-Torres A, Trucco F, Torres S, Vega C, Marrero-Ponce Y, Heguaburu V, Yaluff G, et al. 2008. Activity of a hydroxybibenzyl bryophyte constituent against Leishmania spp. and Trypanosoma cruzi: in silico, in vitro, and in vivo activity studies. Eur J Med Chem. 43:1797–1807.
- Rosas LV, Cordeiro MS, Campos FR, Nascimento SK, Januário AH, França SC, Nomizo A, Toldo MP, Albuquerque S, Pereira PS. 2007. In vitro evaluation of the cytotoxic and trypanocidal activities of Ampelozizyphus amazonicus (Rhamnaceae). Braz J Med Biol Res. 40:663–670.
- Santos KK, Matias EF, Tintino SR, Souza CE, Braga MF, Guedes GM, Rolón M, Vega C, de Arias AR, Costa JG, et al. 2012. Anti-Trypanosoma cruzi and cytotoxic activities of Eugenia uniflora L. Exp Parasitol. 131:130–132.
- Salah SM, Jäger AK. 2005. Screening of traditionally used Lebanese herbs for neurological activities. J Ethnopharmacol. 97:145–149.
- Sobral-Souza CE, Leite NF, Brito DIV, Lavor AK, Alencar LB, Albuquerque RS, Coutinho HD. 2014. Avaliação da atividade citotóxica e potencial antiparasitário in vitro do α-pineno e carvacrol. Acta Toxicol Arg. 22:76–80.
- Tasdemir D, Kaiser M, Brun R, Yardley V, Schmidt TJ, Tosun F, Rüedi P. 2006. Antitrypanosomal and antileishmanial activities of flavonoids and their analogues: in vitro, in vivo, structure-activity relationship, and quantitative structure-activity relationship studies. Antimicrob Agents Chemother. 50:1352–1364.
- Vega C, Rolón M, Martínez-Fernández AR, Escario JA, Gómez-Barrio A. 2005. A new pharmacological screening assay with Trypanosomacruzi epimastigotes expressing β-galactosidase. Parasitol Res. 95:296–298.
- Zgórka G, Glowniak K. 2006. Variation of free phenolic acids in medicinal plants belonging to the Lamiaceae family. J Pharm Biomed Anal. 26:79–87.
- Ziaková A, Brandšteterova E. 2002. Application of different preparation techniques for extraction of phenolic antioxidants from lemon balm (Melissa officinalis) before HPLC analysis. J Liq Chromatog Relat Technol. 25:3017–3032.
- Ziaková A, Brandšteterova E. 2003. Validation of HPLC determination of phenolic acids present in some Lamiaceae family plants. J Liquid Chromatog Relat Technol. 26:443–453.
- World Health Organization (WHO). 1990. Control of the Leishmaniases. WHO Technical Report Series 793. Geneva: World Health Organization. p. 158.