Abstract
Context Syzygium densiflorum Wall. ex Wight & Arn (Myrtaceae) has been traditionally used by local tribes of the Nilgiris, Tamil Nadu, India, for the treatment of diabetes, however, no definitive experimental studies are available.
Objective This study investigates the antidiabetic, antihyperlipidaemic and antioxidant activities of ethanol extract of S. densiflorum (EFSD) fruits in streptozotocin (STZ) and nicotinamide (NA)-induced diabetic rats.
Materials and methods Acute oral toxicity and oral glucose tolerance were assessed in normal rats. The antidiabetic, antihyperlipidaemic and antioxidant activities were investigated in STZ − NA-induced diabetic rats. Diabetic rats were orally administered with glibenclamide (10 mg/kg b.wt), EFSD (200, 400 and 800 mg/kg b.wt) for 28 d. Further, changes in the blood glucose level (BGL), biochemical parameters, antioxidants were observed and histology of pancreas was performed.
Results No toxicity and lethality were observed. Results of the following parameters are represented by treated versus disease control (STZ + NA) groups. BGL (161.33 ± 22.8 versus 476.17 ± 56.58 mg/dl), glycosylated haemoglobin (5.285 ± 0.19 versus 8.05 ± 0.55%), urea (40.32 ± 1.96 versus 75.37 ± 2.91 mg/dl), uric acid (1.2 ± 0.07 versus 2.16 ± 0.05 mg/dl), total cholesterol (89.3 ± 5.14 versus 139.7 ± 5.95 mg/dl) and triglycerides (79.65 ± 2.52 versus 108.9 ± 3.61 mg/dl) were significantly decreased, whereas haemoglobin (11.75 ± 0.73 versus 7.95 ± 0.42 g/dl), high‐density lipoprotein cholesterol (14.2 ± 1.11 versus 6.97 ± 0.84 mg/dl), total protein (45%) and liver glycogen (87%) were significantly increased in EFSD-treated diabetic group. Significant changes were observed in the enzymatic and non-enzymatic antioxidants in EFSD-treated groups (p < 0.001). Histopathological examination showed the regeneration of β-cells in Islets of Langerhans.
Conclusion This study confirms the antidiabetic, antihyperlipidaemic and antioxidant activities of S. densiflorum fruits.
Introduction
Diabetes mellitus is one of the chronic metabolic disorders with the highest prevalence and mortality rate. According to the International Diabetes Federation (IDF), 387 million people have been diagnosed with diabetes around the world and 75 million people have been diagnosed with diabetes in the Southeast Asia (IDF Citation2014). Impairment in the glucose-associated pathways causes deranged glucose homeostasis that leads to hyperglycaemia (Moller Citation2001). Under the hyperglycaemic condition, various factors such as impairment in insulin mechanism, hyperlipidaemia and oxidative stress were causing secondary complications such as diabetic retinopathy and neuropathy, cardiovascular diseases, ulcers and amputations (Ciechanowski et al. Citation2001).
Understanding and improving medication is vital for the management of diabetes and its complications (Larkin et al. Citation2015). Many of the currently available medicines for the management of diabetes have undesired side effects. Hence, interest in the scientific investigation to find alternative therapeutic strategies for the diabetes treatment has become inevitable (Huang et al. Citation2015). Natural products are considered to be an effective alternative for the treatment and management of diabetes, because of higher efficacy and less to nil side effects (Olubomehin et al. Citation2013). World Health Organization (WHO) reports that traditional medicines play a major role in health maintenance, chronic disease prevention and treatment (WHO Citation2013). The importance of plant extracts in diabetes management is widely acknowledged and a large number of medicinal plants have been documented for their effective role in the diabetes management (Petchi et al. Citation2014).
Species of Syzygium (Myrtaceae) exhibit antidiabetic, antilipidaemic and antioxidant properties (Saranya et al. Citation2012). Most of the Syzygium spp. fruits are edible and used for ethnobotanical practices for treating chronic diseases such as cancer, diabetes and cardiovascular diseases (Reynertson et al. Citation2005). Syzygium densiflorum Wall. ex Wight & Arn (Myrtaceae) is an important medicinal plant in the genus Syzygium and paucity of information are available on it therapeutic potential. Ethnobotanical studies find that S. densiflorum fruits have been used for therapeutic purposes and are consumed by local tribes of The Nilgiri Hills, South Western Ghats, India (Sasi & Rajendran Citation2012; Sathyavathi & Janardhanan Citation2014; Vijayan et al. Citation2014). Mohan Maruga Raja et al. (Citation2013) reported that leaves and ripened fruits of S. densiflorum have been used traditionally for the management of diabetes by the local tribes of The Nilgiris, Tamil Nadu, India. However, no strong experimental reports are available to support the antidiabetic properties of S. densiflorum.
In our previous study, the antioxidant and antidiabetic potential of n-hexane, ethyl acetate and ethanol extracts were determined using various in vitro antioxidant assays (2,2-diphenyl-1-picrylhydrazyl radical, superoxide radical scavenging and hydroxyl radical scavenging assays) and α-amylase inhibition assay. Ethanol extract of S. densiflorum (EFSD) fruits showed the highest inhibitory activity compared to n-hexane and ethyl acetate extracts. Besides, the preliminary phytochemical screening test confirmed the presence of high level of flavonoids, sterols, terpenoids, phenols, quinones, carbohydrates, fixed oils and fats in EFSD fruits (Gopinath & Karthikeyan Citation2015). This study assesses the antidiabetic, antilipidaemic and antioxidant properties of S. densiflorum in streptozotocin (STZ) and nicotinamide (NA)-induced diabetic rats.
Materials and methods
Chemicals
STZ and NA were purchased from Sigma Chemical Co. (St. Louis, MO), stored at 2–4 °C and away from direct sunlight. All the used chemicals and kits were of analytical grade and were purchased from standard vendors.
Animals
Healthy adult Wistar rats (male and female), weighing 160–180 g, procured from Central Animal House Facility, SASTRA University, Thanjavur, Tamil Nadu, India. They were housed in polypropylene cages lined with husk and renewed every 24 h under a 12:12 h light dark cycle at 25 ± 3 °C. The housed rats had access to standard diet pellet (Provimi Animal Nutrition India Pvt. Ltd., Bangalore, India) and reverse osmosis purified water ad libitum. The rats were acclimatised to laboratory conditions for 1 week prior to experimentation. This experimentation was approved by the Institutional Animal Ethics Committee, Central Animal House Facility, SASTRA University, Thanjavur (Institutional Animal Ethics Committee No. 277/SASTRA/IAEC/RPP) in accordance with the guidelines of the Committee for the Purpose of Control and Supervision of Experiments on Animals (CPCSEA), New Delhi, India.
Plant materials
Ripened fruits were collected from the Gudalur region, The Nilgiris, Tamil Nadu, India, during May–June, 2013. It was authenticated by Dr. GVS Murthy, Botanical Survey of India (BSI), Southern regional centre, Tamil Nadu Agricultural University campus, Coimbatore, Tamil Nadu, India (Authentication No. BSI/SRC/5/23/2013-14/Tech/60) and a voucher specimen of this plant has been deposited in the herbarium of BSI, Coimbatore (Accession Nos. MH 174300 and MH 174301). Fruits were dried at room temperature (around 25 °C). Subsequently, the dried fruits were mechanically pulverised and extracted successively with n-hexane, ethyl acetate and ethanol using a Soxhlet extractor. The obtained extracts were concentrated using a rotary evaporator under the reduced pressure at 40 °C and ethanol fruit extract was used for further investigation.
Acute toxicity study
The acute oral toxicity (AOT) was carried out as per the guidelines of the Organization for the Economic Co-operation and Development (OECD) (Section 4. Test No. 425). A test dose of 2000 mg/kg b.wt was administered orally to the rats as per the ‘Principle of the Limit Test’ of OECD (OECD Citation2001). Five female rats were fasted overnight and EFSD (2000 mg/kg b.wt, po) was fed to them. The rats were observed every 2 h throughout a day to observe its behavioural, neurological and autonomic profiles. Further, rats were observed every 24 h for 14 d for its lethality.
Oral glucose tolerance test in normal rats (OGTT)
Normal Wistar rats were used for the assessment of oral glucose tolerance. The rats were grouped into three (n = 3) and orally administered with one dose of the vehicle, EFSD (200 mg/kg b.wt) and glibenclamide (10 mg/kg b.wt), respectively. After 30 min, glucose (2000 mg/kg b.wt) was given orally to all the rats. Blood samples from the tail vein were collected at 0, 30, 60 and 120 min for detection of oral glucose tolerance.
Induction of experimental diabetes
Diabetes mellitus was induced in overnight fasted rats, weighing 160–180 g, using STZ and NA. The rats were administered intraperitoneally with STZ (65 mg/kg b.wt, dissolved in 0.1 M citrate buffer, pH 4.5) after 15 min of NA (110 mg/kg b.wt, dissolved in saline) administration (Salahuddin & Jalalpure Citation2010; Deutschländer et al. Citation2012). Hyperglycaemia was confirmed by measuring blood glucose level (BGL) at 48 h after injection of STZ-NA. Rats with BGL ≥ 250 mg/dl were considered as diabetic and they were used in the present study.
Dosage and treatment
A total of 36 diabetic rats were used in this study. The rats were divided into six groups (n = 6): Group I, normal control rats; Group II, disease control rats (STZ + NA); Group III, diabetic rats treated with glibenclamide (10 mg/kg b.wt); Group IV, diabetic rats treated with EFSD (200 mg/kg b.wt, po); Group V, diabetic rats treated with EFSD (400 mg/kg b.wt, po) and Group VI, diabetic rats treated with EFSD (800 mg/kg b.wt, po).
The standard drug glibenclamide (10 mg/kg b.wt), vehicle and EFSD (200, 400 and 800 mg/kg b.wt) were orally administered to diabetic rats for 28 d. Rats were regularly observed for feed and water intake. Urine level was measured using metabolic cages. At the end of 28th day, blood samples were collected from overnight fasted rats by a retro-orbital puncture. Blood samples were collected for plasma and serum analyses. Plasma and serum were collected by centrifugation at 2000 rpm for 10 min. All the rats were sacrificed by carbon dioxide asphyxiation for biochemical analyses. Tissue samples of pancreas, liver and kidney were collected for further investigation. The liver and kidneys were excised immediately, washed in ice-cold saline and stored at −80 °C for further assay. The liver and kidney homogenates were prepared separately by homogenising 1 g of the tissue sample in 9 ml of phosphate buffer saline (pH 7.4). The clear supernatant was obtained by centrifugation at 13 000 g for 25 min at 4 °C and used for enzymatic and non-enzymatic antioxidant assays. The pancreas was stored at 10% neutral buffered formalin solution for histopathological examination.
Biochemical assessments
BGL in the non-fasting rats was measured in blood glucose monitoring system (AP-Plus®, MEDNET GmbH, Germany). Glycosylated haemoglobin (HbA1c) was measured in the blood by an ion-exchange method using the commercial kit (LifeChem™ GHb, Hyderabad, India). Haemoglobin (Hb) was estimated by the cyanmethemoglobin method using the standard procedure as previously reported by Karthikesan et al. (Citation2010). Triglycerides (TG), total cholesterol (TC), high‐density lipoprotein cholesterol (HDL‐C), urea and creatinine levels in the serum were measured using commercial kit (Pariksha Biotech, Hyderabad, India). Uric acid was assessed by uricase/POD method using the commercial kit (Aspen Laboratories Pvt. Ltd., Delhi, India). Total protein was quantified by modified biuret end point assay using the commercial kit (Span Cogent Diagnostics, Surat, India). Liver glycogen was measured using androgen reagent using the procedure described by Van Der Vies (Citation1954).
Assessment of antioxidant activities
Lipid peroxidation (LPO) in the liver and kidney tissue was estimated according to Niehaus and Samuelsson (Citation1968) method using the standard procedure described previously by Mahendran et al. (Citation2014a). Superoxide dismutase (SOD) activity was assayed according to the Marklund and Marklund (Citation1974) method. Catalase (CAT) activity was assayed using the procedure as previously described by Naskar et al. (Citation2011). Reduced glutathione (GSH) was estimated by the method as described previously by Ellman (Citation1959). Vitamin-C (Vit-C) concentration was measured by Omaye et al. (Citation1979) method.
Histopathological study
The pancreas was dissected out, fixed in 10% formalin and embedded in paraffin. Four micrometre-thick paraffin sections were stained with haematoxylin and eosin (Stevens Citation1994). Effect of EFSD in the STZ-induced pancreatic damage was observed under a light microscope (Magnification HE. 40×).
Statistical analysis
The experimental results were represented as mean with standard error of the mean (SEM). Statistical differences between the means of the various groups were evaluated using one-way analysis of variance followed by Tukey’s multiple comparison tests. All statistical analyses were performed using GraphPad Prism software (version 5.0) (GraphPad software, San Diego, CA).
Results
Acute oral toxicity test
AOT was performed in female rats as per the OECD 425 guidelines. No significant changes in body weight, feed intake and behaviour pattern were noted in EFSD administered rats.
Oral glucose tolerance test
The BGL (mg/dl) was measured at 30-min time intervals (from 30 to 120 min) (). BGL was measured after 30 min administration of the EFSD (200 mg/kg b.wt) and glibenclamide (10 mg/kg b.wt). All the rats were orally fed with glucose (2000 mg/kg b.wt) and changes in the BGL were observed. There were no changes in the normal BGL of rats on administration of the EFSD and glibenclamide, whereas EFSD and glibenclamide controlled the BGL in glucose administered rats from 30 min. Fall in the BGL was observed from 30 to 120 min in glibenclamide and EFSD-treated groups. Statistical significance was observed from 30 to 60 min compared with disease control (p < 0.05). After 120 min, the BGL returned to the normal level in all the experimental groups. Herein, OGTT was carried with a single concentration of EFSD (200 mg/kg b.wt) to check its ability to regulate glucose metabolism in glucose administered rat with a limited number of groups. However, additional concentrations and groups are required to provide the reliable conclusion.
Table 1. Time course of blood glucose level in normal, glibenclamide and EFSD administered rats during oral glucose tolerance test.
Changes in feed and water intake, urine level and body weight
Feed and water intake of all the experimental groups were measured daily (). The results were expressed as the amount of feed and water intake of a rat per day. Disease control (STZ + NA) and EFSD (800 mg/kg b.wt) treated groups were showed statistical significance (p < 0.05) with normal control and disease control (STZ + NA), respectively. The highest feed and water intake values were observed in disease control (STZ + NA) group when compared to other groups; the initial and final body weights of all rats were compared. As a result, no significant changes in the body weight were observed. However, the body weight of the disease control (STZ + NA) was increased when compared to other groups; whereas EFSD (200, 400 and 800 mg/kg b.wt) treated groups maintained its body weight (). Urine level was measured in all the experimental groups. The individual rats were placed in metabolic cages for 24 h to collect the urine. The urine level of disease control (STZ + NA) group was found to be significantly higher than the normal control (p < 0.001). EFSD (200, 400 and 800 mg/kg b.wt) treated groups were significantly lower than the disease control (STZ + NA) and glibenclamide treated groups (p < 0.001) ().
Figure 1. Changes in the feed (g) and water (ml) intake, where plotted values represents mean ± SEM of feed (g) and water (ml) intake, in which acomparison of disease control (STZ + NA) versus normal control, bcomparison of treated group versus disease control (STZ + NA). The level of significance: *p < 0.05.
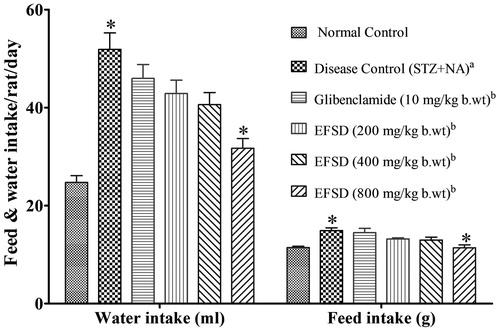
Table 2. Effect of EFSD in the body weight, urine level, HbA1c and Hb.
Effect of EFSD on blood glucose level in diabetic rats
BGL was estimated in the non-fasting experimental rats. BGL in the non-fasting STZ − NA-induced rats was found at 429 ± 12.26 mg/dl in the initial day of the treatment. During the treatment, BGL was measured once in a week for entire experimental groups. No significant changes were observed at the end of the first week (day 7). Nevertheless, a significant (p < 0.01) decrease in BGL was observed in glibenclamide and EFSD (200, 400 and 800 mg/kg b.wt) treated groups ().
Effect on biochemical parameters
The glycosylated Hb (HbA1c) level was significantly increased in the disease control (STZ + NA) when compared to normal control (p < 0.001). The HbA1c level was lowered in glibenclamide and EFSD (200, 400 and 800 mg/kg b.wt) treated groups. The HbA1c level was showed a high significance in glibenclamide and EFSD (800 mg/kg b.wt) treated groups (p < 0.001), whereas the EFSD (400 mg/kg b.wt) treated group showed a less significance compared to disease control (STZ + NA) (p < 0.01). While, Hb level was significantly decreased in disease control (STZ + NA) when compared to normal control (p < 0.001). Glibenclamide and EFSD (800 mg/kg b.wt) treated groups were showed a significant increase in Hb level than the EFSD (200, 400 mg/kg b.wt) (p < 0.001) ().
Renal function parameters such as urea, uric acid and CR levels were significantly increased in disease control (STZ + NA) when compared to normal control (p < 0.01), whereas these were significantly decreased in glibenclamide and EFSD (200, 400 and 800 mg/kg b.wt) treated groups when compared to disease control (STZ + NA) (p < 0.01) ().
Table 3. Effect of EFSD in renal function parameters: urea, uric acid and CR levels.
Effect on lipid profile
TC, HDL-C and TG were estimated (). Compared to normal control, disease control (STZ + NA) showed a significant increase in TC, TG levels and a decrease in HDL-C level (p < 0.001). The glibenclamide and EFSD (800 mg/kg b.wt) treated groups were showed a significant decrease in the TC, TG and HDL-C when compared to disease control (STZ + NA) (p < 0.01).
Table 4. Effect of EFSD in lipid profiles: TC, HDL-C and TG.
Effect on liver glycogen and total protein
Effect on liver glycogen in normal, diseased, glibenclamide and EFSD (200, 400 and 800 mg/kg b.wt) treated groups is shown in . Compared to normal control, liver glycogen level was significantly lowered in the disease control (STZ + NA) (p < 0.001), whereas it was significantly increased in the glibenclamide and EFSD (200, 400 and 800 mg/kg b.wt) treated groups when compared to disease control (STZ + NA) (p < 0.01).
Figure 3. Changes in the liver glycogen level after the treatment of EFSD in various concentrations, where plotted values are mean ± SEM of liver glycogen of normal control group, disease control (STZ + NA) group, glibenclamide treated group (10 mg/kg b.wt), EFSD (200, 400 and 800 mg/kg b.wt) treated groups, in which acomparison of disease control (STZ + NA) versus normal control, bcomparison of treated group versus disease control (STZ + NA). The level of significance: **p < 0.01; ***p < 0.001.
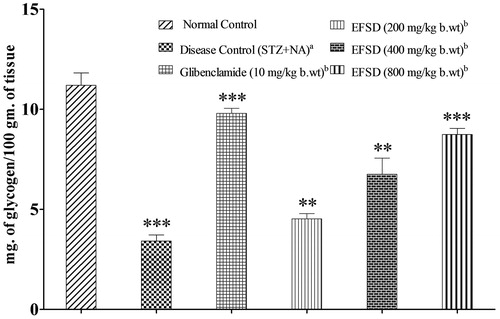
The total protein (TP) level was estimated in the liver and kidney (). Liver and kidney protein levels were significantly decreased in the disease control (STZ + NA) when compared to normal control (p < 0.05), whereas it was significantly increased in the glibenclamide and EFSD (800 mg/kg b.wt) treated groups when compared to disease control (STZ + NA) (p < 0.05).
Figure 4. Changes in the total protein after the treatment in all experimental groups, where plotted values are mean ± SEM of total protein concentration, in which acomparison of disease control (STZ + NA) versus normal control, bcomparison of treated group versus disease control (STZ + NA). The level of significance: *p < 0.05.
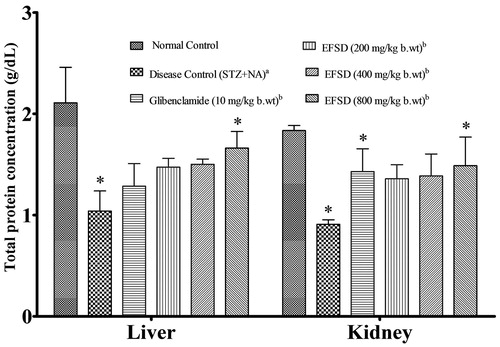
Effect on oxidative stress
Lipid peroxidation
The oxidative degradation of lipids in liver and kidney was estimated by measuring thiobarbituric acid reactive substances which were expressed in terms of malondialdehyde (MDA) content (). MDA level in liver and kidney were significantly increased in the disease control (STZ + NA) when compared to normal control (p < 0.001), whereas glibenclamide and EFSD (200, 400 and 800 mg/kg b.wt) treated groups were showed a significant reduction compared to disease control (STZ + NA) (p < 0.05).
Table 5. Effect of EFSD in the oxidative markers: LPO, SOD, CAT, GSH and Vit-C.
Effect on enzymatic antioxidants
The SOD and CAT activities of liver and kidney are shown in . Compared to normal control, a significant reduction in SOD and CAT levels were noted in the disease control (STZ + NA), whereas glibenclamide and EFSD (200, 400, and 800 mg/kg b.wt) treated groups showed a significant increase in SOD and CAT activity compared with the disease control (STZ + NA) (p < 0.05).
Effect on non-enzymatic antioxidants (GSH and Vit-C)
The activity of non-enzymatic antioxidants such as GSH and Vit-C were measured and values are shown in . The GSH and Vit-C activities in the disease control (STZ + NA) were decreased compared with normal control. A significant increase in the GSH activity was observed in the glibenclamide and EFSD (400, 800 mg/kg b.wt) treated groups when compared with disease control (STZ + NA) (p < 0.001). Similarly, Vit-C activity in the glibenclamide and EFSD (800 mg/kg b.wt) treated groups showed a significant increase when compared to disease control (STZ + NA) (p < 0.01).
Effect on pancreases histopathology
Histopathology of the pancreas from normal control, disease control (STZ + NA) and glibenclamide, EFSD (800 mg/kg b.wt) treated groups are shown in . Islets of Langerhans with granulated cytoplasm and acini tissues were normal in the pancreas of normal control rats (), whereas pancreas of diseased rat exhibited irregular necrotic pancreatic acini and cytoplasmic degeneration. Besides, irregular outlining of the Islets of Langerhans was observed in the disease control (STZ + NA) (). shows the gradual increase in the restoration in the exocrine pancreatic tissue (acinar cells) and endocrine pancreatic tissue (Islets of Langerhans) when compared to the disease control (STZ + NA).
Figure 5. Histopathology analysis of pancreas (Magnification HE. 40×), it illustrates (A) normal Islets of Langerhans with granulated cytoplasm and acini tissues in normal rat, (B) irregular necrotic pancreatic acini (marked with circle) and cytoplasmic degeneration (short arrow) also irregular outlining (long arrow) in the STZ − NA-induced disease control (STZ + NA) and (C) glibenclamide treated and (D) EFSD (800 mg/kg b.wt) treated shows restoration in the islets of Langerhans (short arrow) and regular lining of pancreatic acini around the Langerhans (marked with circle).
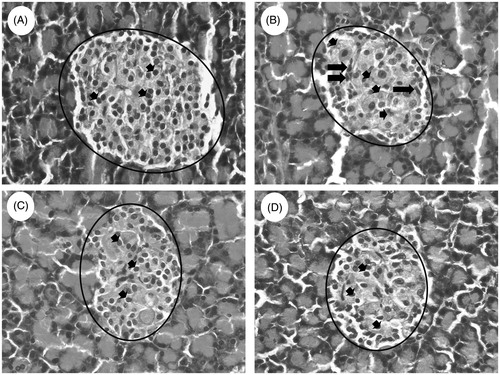
Discussion
It has been reported that the fruits of Syzygium sp. have been used to lower the BGL during hyperglycaemia three decades prior to the discovery of insulin (Helmstädter Citation2008). The fruits of Syzygium sp. also exhibit various pharmacological activities such as antidiarrhoeal, antidiuretic properties, antihyperlipidaemic, anti-inflammatory, antimicrobial, antioxidant, antiscorbutic, antiulcerogenic, astringent, cardioprotective, hepatoprotective and stomachic properties (Baliga et al. Citation2011; Bag et al. Citation2012; Ramya et al. Citation2012). The present study, in vivo experiment, was carried out with the ethanol fruit extract of S. densiflorum to evaluate the antidiabetic, antilipidaemic and antioxidant properties in STZ − NA-induced rats. Blood glucose, initial and final body weight, feed and water intake, urine level, HbA1c, Hb, liver glycogen, TP, renal function and antioxidant parameters were analysed.
In general, polyphagia, polydipsia and polyuria are associated with diabetes and considered as the main symptoms for diagnosing diabetes (Rao et al. Citation2001). All the above-said symptoms were observed in the present study, along with the increased body mass index. An increase in the body weight was associated with type 2 diabetes; it could be due to reduced insulin sensitivity and impaired glucose homeostasis (Hermansen & Mortensen Citation2007; Skovsø Citation2014). It was observed that EFSD lowered BGL after 28 d of the treatment. Effectiveness in lowering blood glucose in STZ − NA-induced rats increased as the concentration of EFSD increased, which is due to improved insulin secretion and insulin action on potentiating glucose uptake by various tissues (Pierre et al. Citation2012).
Increased HbA1c and decreased Hb levels were mainly considered for diagnosis and prognosis of diabetes complications (Sundaram et al. Citation2012). Similar observations in the present study could be due to normalisation of insulin-mediated glucose level and an anabolic effect of insulin action on protein synthesis (Ramachandran et al. Citation2013; Mahendran et al., Citation2014b).
Serum urea, uric acid and CR level demonstrate the role EFSD in renal dysfunction. An augmented level of urea, uric acid and CR cause the osmotic diuresis and depletion of extracellular fluid volume in the diabetic condition in the serum of STZ − NA-induced diabetic rats (Sheela et al. Citation2013). The EFSD (200, 400 and 800 mg/kg b.wt) treated groups showed a declined level of urea, uric acid, and CR. Thus, the positive effect of EFSD in renal dysfunction was revealed (Badole & Bodhankar Citation2010). It is well known that augmentation in TC and TG, the decline in the HDL-C are regarded as most common abnormalities in disease control (STZ + NA) group. Herein, the decreased level of TC, TG and the increased level of HDL-C in EFSD (200, 400 and 800 mg/kg b.wt) treated groups confirm the positive role of EFSD in increased insulin action on cholesterol and fatty acid biosynthesis and intestinal cholesterol absorption (Wang et al. Citation2014).
TP and liver glycogen level were estimated in the liver and kidney of normal, diseased, glibenclamide and EFSD (200, 400 and 800 mg/kg b.wt) treated groups. Insulin, cell proliferation, oxidative stresses were found to be associated with TP concentration (Pari & Srinivasan Citation2010). In this study, it was observed that TP was reduced in disease control (STZ + NA) and increased in glibenclamide and EFSD (200, 400 and 800 mg/kg b.wt) treated groups. Similar observations were noticed in STZ-induced diabetic rats (Rao et al. Citation2013). Besides, a moderate increase in liver glycogen of EFSD (200, 400, and 800 mg/kg b.wt) treated groups reveals the improved insulin action to maintain the glucose level by glycogenesis, glycogenolysis and gluconeogenesis (Palsamy & Subramanian Citation2009; Shukla et al. Citation2012).
STZ induction causes tissue damage by producing oxidative stress and hyperglycaemia by increasing free radical and ROS generation (Moradi-Afrapoli et al. Citation2012). The SOD and CAT are two major scavenging enzymes that remove free radicals. The increased SOD and CAT activities in EFSD (200, 400 and 800 mg/kg b.wt) treated groups reveal free radical scavenging property and confirm the beneficial effect of S. densiflorum fruit extract (Dewanjee et al. Citation2009). The decreased activity of endogenous antioxidant enzymes (SOD and CAT) also causes initiation and propagation of LPO. An increased oxidative stress leads to the rise in LPO level and it is associated with an elevation in BGL (Singh & Kakkar Citation2009; Kharbanda et al. Citation2014). Decreased MDA level in EFSD (200, 400 and 800 mg/kg b.wt) treated groups show the reversal of LPO level that supports the antioxidant activity of EFSD in STZ − NA-induced diabetic rats. A similar observation was observed in STZ − NA-induced diabetic rats by Shajeela et al. (Citation2012). Diabetes mediated oxidative stress also correlates with depletion in the activity of non-enzymatic antioxidants such as GSH, and Vit-C (Ramar et al. Citation2012). An elevated GSH ratio in EFSD (200, 400 and 800 mg/kg b.wt) treated groups showed that EFSD increases the GSH biosynthesis and prevents toxic effects against the increased ROS (Bagri et al. Citation2009). The increased level of Vit-C in EFSD (200, 400 and 800 mg/kg b.wt) treated groups confirms the reduced utilisation of Vit-C against the augmented ROS (Srinivasan & Pari Citation2012).
In this study, LPO, enzymatic and non-enzymatic antioxidants were used as markers to evaluate antioxidant potential of EFSD in STZ − NA-induced diabetic rats. Aforementioned observations with antioxidant markers support the antioxidant efficacy of EFSD. Thus, it is proved that the EFSD prevents the diabetic associated kidney damage and reduces the complication associated with increased oxidative stress. This could be due to the positive role of flavonoids and phenolics in free radical scavenging and antioxidant activities by activating the detoxification mechanism to prevent the oxidative stress associated cellular damage (Patel et al. Citation2014; Abdelaziz et al. Citation2015).
The fruits of Syzygium spp. have the potential to regenerate the Islets of Langerhans (Bhat & Joshi Citation2011). Histopathology examination of pancreas also supports the restoration capacity of EFSD in pancreatic cells (). Partial destruction of pancreas confirms STZ induced pancreatic tissue damage, while restoration in the exocrine acinar cells, Islets of Langerhans and increased cellularity of β-cell confirms the restoration capacity of EFSD in pancreatic cells. Overall, the present investigation supports the antidiabetic, antihyperlipidaemic and antioxidant potential of ethanol fruit extract of S. densiflorum because of the presence of flavonoids, sterols, terpenoids, phenols, carbohydrates and quinones (Reynertson et al. Citation2005; Mendonça-Filho Citation2006; Kumar et al. Citation2011; Patel et al. Citation2012; Arif et al. Citation2014; Mootoosamy & Fawzi Mahomoodally Citation2014).
Conclusion
The EFSD has a beneficial effect on hyperglycaemia, hyperlipidaemia and oxidative stress in STZ − NA-induced diabetic rats by improving insulin secretion through β-cell restoration capacity. Active phytoconstituents such as flavonoids, sterols, terpenoids, phenols, carbohydrates and quinones of EFSD are responsible for antihyperglycaemic, antihyperlipidaemic and antioxidant properties of EFSD. Furthermore, this study provides evidence for the ethnobotanical use of S. densiflorum in the treatment of diabetes mellitus. However, further studies are necessary for the isolation and purification of bioactive compounds from EFSD, this could be a limitation of the study. Identification of the novel and potent bioactive compounds with specific chemical moieties will provide a new therapeutics strategy for the treatment and management of diabetes.
Acknowledgements
The authors are grateful to Dr. Gino A. Kurian, Professor, School of Chemical & Biotechnology, SASTRA University, Thanjavur, Tamil Nadu, India, for his indeed support in biochemical analysis. We also thank Central Animal House Facility (CAF), SASTRA University and Department of Science and Technology, Govt. of India (IFA-CH-04 and #SB/FT/CS-024/2013) for supporting equipment facilities to carry out the study.
Declaration of interest
There is no conflict of interest of any author.
References
- Abdelaziz DH, Ali SA, Mostafa MM. 2015. Phoenix dactylifera seeds ameliorate early diabetic complications in streptozotocin-induced diabetic rats. Pharm Biol. 53:792–799.
- Arif T, Sharma B, Gahlaut A, Kumar V, Dabur R. 2014. Anti-diabetic agents from medicinal plants: a review. Chem Biol Lett. 1:1–13.
- Badole SL, Bodhankar SL. 2010. Antidiabetic activity of cycloart-23-ene-3beta, 25-diol (B2) isolated from Pongamia pinnata (L. Pierre) in streptozotocin-nicotinamide induced diabetic mice. Eur J Pharmacol. 632:103–109.
- Bag A, Bhattacharyya SK, Pal NK, Chattopadhyay RR. 2012. In vitro antibacterial potential of Eugenia jambolana seed extracts against multidrug-resistant human bacterial pathogens. Microbiol Res. 167:352–357.
- Bagri P, Ali M, Aeri V, Bhowmik M, Sultana S. 2009. Antidiabetic effect of Punica granatum flowers: effect on hyperlipidemia, pancreatic cells lipid peroxidation and antioxidant enzymes in experimental diabetes. Food Chem Toxicol. 47:50–54.
- Baliga MS, Bhat HP, Baliga BRV, Wilson R, Palatty PL. 2011. Phytochemistry, traditional uses and pharmacology of Eugenia jambolana Lam. (black plum): a review. Food Res Int. 44:1776–1789.
- Bhat MD, Joshi BN. 2011. Seeds of Syzygium cumini (L.) Skeels: potential for islet regeneration in experimental diabetes. Zhong Xi Yi Jie He Xue Bao. 9:1380–1387.
- Ciechanowski PS, Katon WJ, Russo JE, Walker EA. 2001. The patient-provider relationship: attachment theory and adherence to treatment in diabetes. Am J Psychiatry. 158:29–35.
- Deutschländer MS, Lall N, Van de Venter M, Dewanjee S. 2012. The hypoglycemic activity of Euclea undulata Thunb. var. myrtina (Ebenaceae) root bark evaluated in a streptozotocin-nicotinamide induced type 2 diabetes rat model. South African J Bot. 80:9–12.
- Dewanjee S, Das AK, Sahu R, Gangopadhyay M. 2009. Antidiabetic activity of Diospyros peregrina fruit: effect on hyperglycemia, hyperlipidemia and augmented oxidative stress in experimental type 2 diabetes. Food Chem Toxicol. 47:2679–2685.
- Ellman GL. 1959. Tissue sulfhydryl groups. Arch Biochem Biophys. 82:70–77.
- Gopinath K, Karthikeyan M. 2015. In vitro evaluation of antioxidant and antidiabetic activities of Syzygium densiflorum fruits. Asian Pacific J Trop Dis. 5:912–917.
- Helmstädter A. 2008. Syzygium cumini (L.) Skeels (Myrtaceae) against diabetes – 125 years of research. Pharmazie 63:91–101.
- Hermansen K, Mortensen LS. 2007. Bodyweight changes associated with antihyperglycaemic agents in type 2 diabetes mellitus. Drug Saf. 30:1127–1142.
- Huang D, Jiang Y, Chen W, Yao F, Huang G, Sun L. 2015. Evaluation of hypoglycemic effects of polyphenols and extracts from Penthorum chinense. J Ethnopharmacol. 163:256–263.
- IDF. 2014. IDF Diabetes Atlas. 6th edn. 2014 update. Belgium: International Diabetes Federation.
- Karthikesan K, Pari L, Menon V. 2010. Combined treatment of tetrahydrocurcumin and chlorogenic acid exerts potential antihyperglycemic effect on streptozotocin-nicotinamide-induced diabetic rats. Gen Physiol Biophys. 29:23–30.
- Kharbanda C, Sarwar Alam M, Hamid H, Bano S, Haider S, Nazreen S, Ali Y, Javed K. 2014. Trapa natans L. root extract suppresses hyperglycemic and hepatotoxic effects in STZ-induced diabetic rat model. J Ethnopharmacol. 151:931–936.
- Kumar R, Pate DK, Prasad SK, Sairam K, Hemalatha S. 2011. Antidiabetic activity of alcoholic leaves extract of Alangium lamarckii Thwaites on streptozotocin-nicotinamide induced type 2 diabetic rats. Asian Pac J Trop Med. 4:904–909.
- Larkin AT, Hoffman C, Stevens A, Douglas A, Bloomgarden Z. 2015. Determinants of adherence to diabetes treatment. J Diabetes. 6:864–871.
- Mahendran G, Manoj M, Murugesh E, Sathish Kumar R, Shanmughavel P, Rajendra Prasad KJ, Narmatha Bai V. 2014a. In vivo anti-diabetic, antioxidant and molecular docking studies of 1, 2, 8-trihydroxy-6-methoxy xanthone and 1, 2-dihydroxy-6-methoxyxanthone-8-O-β-d-xylopyranosyl isolated from Swertia corymbosa. Phytomedicine 21:1237–1248.
- Mahendran G, Thamotharan G, Sengottuvelu S, Bai VN. 2014b. Anti-diabetic activity of Swertia corymbosa (Griseb.) Wight ex C.B. Clarke aerial parts extract in streptozotocin induced diabetic rats. J Ethnopharmacol. 151:1175–1183.
- Marklund S, Marklund G. 1974. Involvement of the superoxide anion radical in the autoxidation of pyrogallol and a convenient assay for superoxide dismutase. Eur J Biochem. 47:469–474.
- Mendonça-Filho RR. 2006. Bioactive phytocompounds: new approaches in the phytosciences. In: Ahmad I, Aqil F, Owais M, editors. Modern phytomedicine: turning medicinal plants into drugs. Weinheim: WILEY-VCH Verlag GmbH & Co. p. 1–24.
- Mohan Maruga RM, Agilandeswari D, Dhanabal S. 2013. Pharmacognostical, antidiabetic and antioxidant studies on Syzygium densiflorum leaves. Contemp Investig Obs Pharm. 2:43–51.
- Moller DE. 2001. New drug targets for type 2 diabetes and the metabolic syndrome. Nature 414:821–827.
- Mootoosamy A, Fawzi Mahomoodally M. 2014. Ethnomedicinal application of native remedies used against diabetes and related complications in Mauritius. J Ethnopharmacol. 151:413–444.
- Moradi-Afrapoli F, Asghari B, Saeidnia S, Ajani Y, Mirjani M, Malmir M, Dalatabadi R, Hadjiakhoondi A, Salehi P, Hamburger M, et al. 2012. In vitro α-glucosidase inhibitory activity of phenolic constituents from aerial parts of Polygonum hyrcanicum. DARU 20:1–6.
- Naskar S, Mazumder UK, Pramanik G, Gupta M, Suresh Kumar RB, Bala A, Islam A. 2011. Evaluation of antihyperglycemic activity of Cocos nucifera Linn. on streptozotocin induced type 2 diabetic rats. J Ethnopharmacol. 138:769–773.
- Niehaus WG Jr, Samuelsson B. 1968. Formation of malonaldehyde from phospholipid arachidonate during microsomal lipid peroxidation. Eur J Biochem. 6:126–130.
- OECD. (2001). Guidelines for testing of chemicals, guidance. Section 4. Test Guideline 425: Acute Oral Toxicity-Up-and-Down Procedure. 1–26
- Olubomehin OO, Abo KA, Ajaiyeoba EO. 2013. Alpha-amylase inhibitory activity of two Anthocleista species and in vivo rat model anti-diabetic activities of Anthocleista djalonensis extracts and fractions. J Ethnopharmacol. 146:811–814.
- Omaye ST, Turnbull JD, Sauberlich HE. 1979. Selected methods for the determination of ascorbic acid in animal cells, tissues, and fluids. Methods Enzymol. 62:3–11.
- Palsamy P, Subramanian S. 2009. Modulatory effects of resveratrol on attenuating the key enzymes activities of carbohydrate metabolism in streptozotocin-nicotinamide-induced diabetic rats. Chem Biol Interact. 179:356–362.
- Pari L, Srinivasan S. 2010. Antihyperglycemic effect of diosmin on hepatic key enzymes of carbohydrate metabolism in streptozotocin-nicotinamide-induced diabetic rats. Biomed Pharmacother. 64:477–481.
- Patel DK, Prasad SK, Kumar R, Hemalatha S. 2012. An overview on antidiabetic medicinal plants having insulin mimetic property. Asian Pac J Trop Biomed. 2:320–330.
- Patel JJ, Acharya SR, Acharya NS. 2014. Clerodendrum serratum (L.) Moon. – a review on traditional uses, phytochemistry and pharmacological activities. J Ethnopharmacol. 154:268–285.
- Petchi RR, Vijaya C, Parasuraman S. 2014. Antidiabetic activity of polyherbal formulation in streptozotocin – nicotinamide induced diabetic Wistar rats. J Tradit Complement Med. 4:108–117.
- Pierre W, Gildas AJH, Ulrich MC, Modeste W-N, Benoît NT, Albert K. 2012. Hypoglycemic and hypolipidemic effects of Bersama engleriana leaves in nicotinamide/streptozotocin-induced type 2 diabetic rats. BMC Complement Altern Med. 12:6.
- Ramachandran S, Rajasekaran A, Adhirajan N. 2013. In vivo and in vitro antidiabetic activity of Terminalia paniculata bark: an evaluation of possible phytoconstituents and mechanisms for blood glucose control in diabetes. ISRN Pharmacol. 2013:1–10.
- Ramar M, Manikandan B, Raman T, Priyadarsini A, Palanisamy S, Velayudam M, Munusamy A, Marimuthu Prabhu N, Vaseeharan B. 2012. Protective effect of ferulic acid and resveratrol against alloxan-induced diabetes in mice. Eur J Pharmacol. 690:226–235.
- Ramya S, Neethirajan K, Jayakumararaj R. 2012. Profile of bioactive compounds in Syzygium cumini – a review. J Pharm Res. 5:4548–4553.
- Rao BK, Kesavulu MM, Apparao C. 2001. Antihyperglycemic activity of Momordica cymbalaria in alloxan diabetic rats. J Ethnopharmacol. 78:67–71.
- Rao PV, Madhavi K, Dhananjaya Naidu M, Gan SH. 2013. Rhinacanthus nasutus improves the levels of liver carbohydrate, protein, glycogen, and liver markers in streptozotocin-induced diabetic rats. Evid Based Complement Altern Med. 2013:1–7.
- Reynertson KA, Basile MJ, Kennelly EJ. 2005. Antioxidant potential of seven Myrtaceous fruits. Ethnobot Res Appl. 3:25–35.
- Salahuddin M, Jalalpure SS. 2010. Antidiabetic activity of aqueous fruit extract of Cucumis trigonus Roxb. in streptozotocin-induced-diabetic rats. J Ethnopharmacol. 127:565–567.
- Saranya J, Eganathan P, Sujanapal P, Parida A. 2012. Chemical composition of leaf essential oil of Syzygium densiflorum Wall. ex Wt & Arn.-A vulnerable tree species. J Essent Oil Bear Plants. 15:283–287.
- Sasi R, Rajendran A. 2012. Diversity of wild fruits in Nilgiri Hills of the Southern Western Ghats – ethnobotanical aspects. Int J Appl Biol Pharm Technol. 3:82–87.
- Sathyavathi R, Janardhanan K. 2014. Wild edible fruits used by Badagas of Nilgiri District, Western Ghats, Tamilnadu, India. J Med Plants Res. 8:128–132.
- Shajeela PS, Kalpanadevi V, Mohan VR. 2012. Potential antidiabetic, hypolipidaemic and antioxidant effects of Nymphaea pubescens extract in alloxan induced diabetic rats. J Appl Pharm Sci. 2:83–88.
- Sheela N, Jose MA, Sathyamurthy D, Kumar BN. 2013. Effect of silymarin on streptozotocin-nicotinamide-induced type 2 diabetic nephropathy in rats. Iran J Kidney Dis. 7:117–123.
- Shukla K, Dikshit P, Shukla R, Gambhir JK. 2012. The aqueous extract of Withania coagulans fruit partially reverses nicotinamide/streptozotocin-induced diabetes mellitus in rats. J Med Food. 15:718–725.
- Singh J, Kakkar P. 2009. Antihyperglycemic and antioxidant effect of Berberis aristata root extract and its role in regulating carbohydrate metabolism in diabetic rats. J Ethnopharmacol. 123:22–26.
- Skovsø S. 2014. Modeling type 2 diabetes in rats using high fat diet and streptozotocin. J Diabetes Investig. 5:349–358.
- Srinivasan S, Pari L. 2012. Ameliorative effect of diosmin, a citrus flavonoid against streptozotocin-nicotinamide generated oxidative stress induced diabetic rats. Chem Biol Interact. 195:43–51.
- Stevens A. 1994. Connective tissues and stains. In: Bancroft JSA, editor. Theory and practice of histological techniques. 6th ed. Philadelphia (PA): Churchill Livingstone/Elsevier. p. 129–134.
- Sundaram R, Naresh R, Shanthi P, Sachdanandam P. 2012. Efficacy of 20-OH-ecdysone on hepatic key enzymes of carbohydrate metabolism in streptozotocin induced diabetic rats. Phytomedicine 19:725–729.
- Van Der Vies J. 1954. Two methods for the determination of glycogen in liver. Biochem J. 57:410–416.
- Vijayan J, Ramachandran V, Thomas B. 2014. Role of botanic gardens in conserving edible plants. Eur J Environ Ecol. 1:26–28.
- Wang W, He Y, Lin P, Li Y, Sun R, Gu W, Yu J, Zhao R. 2014. In vitro effects of active components of Polygonum multiflorum Radix on enzymes involved in the lipid metabolism. J Ethnopharmacol. 153:763–770.
- WHO. 2013. WHO Traditional Medicine Strategy. Geneva: WHO Library Cataloguing-in-Publication Data. p. 1–87