Abstract
Context In a previous study, the total extract of Melaleuca styphelioides Sm. (Myrtaceae) showed a significant hepatoprotective effect in a CCl4-induced toxicity model in mice. However, the active components responsible for the activity of the extract were not identified.
Objective To determine the in vitro hepatoprotective activity of the isolated pure compounds from M. styphelioides leaves using the CCl4-challenged HepG2 cell model.
Materials and methods The hepatoprotective activity of the compounds (at concentrations of 100, 50 and 25 μm), the total extract and silymarin (Sil) (100, 50 and 25 μg/ml) was determined by measuring the activities of alanine aminotransferase (ALT) and aspartate aminotransferase (AST) after pretreatment with the tested samples for one hour. Glutathione (GSH) and superoxide dismutase activity (SOD) were estimated to determine the mechanisms of the hepatoprotective activity.
Results Some compounds showed marked hepatoprotection, including tellimagrandin I, which produced 42, 36 and 31% decrease in ALT and 47, 43 and 37% decrease in AST, at the tested concentrations, respectively, pedunculagin (32, 32 and 30% decrease for ALT and 48, 48 and 45% for AST), tellimagrandin II (38, 32 and 26% decrease for ALT and 45, 40 and 34% for AST) and pentagalloyl glucose (30, 28 and 26% decrease for ALT and 45, 38 and 36% for AST). Tellimagrandin I and II showed the highest increase in GSH (113, 105 and 81% and 110, 103 and 79%, respectively), which was comparable to Sil. Pedunculagin produced the highest increase in SOD (497, 350 and 258%).
Conclusion This study highlights promising natural hepatoprotective candidates derived from M. styphelioides.
Introduction
The liver has an important role in the metabolism and detoxification of xenobiotics. Chronic exposure to drugs and pollutants overwhelm the detoxification power of the liver and lead to liver injury (Ramachandra Setty et al. Citation2007). Liver damage is associated with enzyme leakage, oxidative stress and lipid peroxidation (Ramachandra Setty et al. Citation2007). There is now general agreement among pharmacologists that the number of effective drugs currently available for the treatment of liver diseases is not sufficient (Muriel & Rivera-Espinoza Citation2008). Corticosteroids and colchicine have been used for the treatment of several liver disorders; however, they are of limited therapeutic benefits and produce serious adverse effects (Muriel & Rivera-Espinoza Citation2008; Srivastava & Shivanandappa Citation2010). Interferons have been used in the treatment of patients having cirrhotic or fibrotic diseases; however, interferons are expensive and have serious adverse effects (Muriel & Rivera-Espinoza Citation2008). Silymarin (Sil) is a popular herbal extract used as a hepatoprotective agent; however, some clinical trials have shown that Sil was ineffective in the treatment of many patients having liver disease (Jacobs et al. Citation2002; Muriel & Rivera-Espinoza Citation2008). In addition, serious adverse effects, including gastroenteritis have been reported in patients using Sil. Development of relapses is also common after using Sil, which makes its efficacy arguable (Muriel & Rivera-Espinoza Citation2008). In view of the limited drugs available for the treatment of liver diseases, there is an urgent need for the development of safe, effective new candidates for the treatment of liver diseases.
Natural products represent a new therapeutic strategy in the search for new and effective hepatoprotective agents (Fahmy et al. Citation2016). They offer great potential as hepatoprotective agents because of their reduced side effects when compared to synthetic drugs (Srivastava & Shivanandappa Citation2010). Experimental evidence indicates that ellagitannins have potent radical scavenging activity and inhibitory effect on lipid peroxidation (Al-Sayed & El-Naga Citation2015; Shimoda et al. Citation2008). Ellagitannins can prevent the liver injury induced by free radicals through different mechanisms, including radical scavenging, metal chelation and activation of antioxidant defence mechanisms, including superoxide dismutase (SOD) and glutathione (GSH) (Al-Sayed et al. Citation2010; Okuda Citation2005). Previous studies have reported that various Melaleuca species are rich sources of ellagitannins (Al-Sayed et al. Citation2014; Yoshida et al. Citation2008). In a previous study, we demonstrated that the total extract of Melaleuca styphelioides Sm. (Myrtaceae) possessed a potent hepatoprotective effect against the liver toxicity induced by CCl4 in mice (Al-Sayed et al. Citation2014). However, the main active components responsible for the activity of the extract were not identified. This prompted us to determine the in vitro hepatoprotective activity of the individual pure compounds isolated from M. styphelioides in HepG2 cells challenged with CCl4, with the aim to discover new effective hepatoprotective candidates from cheap natural sources. Hepatoprotection was determined by assaying the activities of alanine aminotransferase (ALT) and aspartate aminotransferase (AST) in the culture medium of HepG2 cells treated with CCl4. The antioxidant parameters SOD and reduced GSH were estimated in the cell lysates to determine the possible mechanisms of the hepatoprotective activity. The isolated compounds were identified by spectral data.
Materials and methods
General experimental procedures
The NMR spectra were measured by a Bruker Avance 500 spectrometer (Bruker BioSpin Inc., Fällanden, Switzerland). CD3OD was used as a solvent. The LC-HRESIMS analysis was performed on a Bruker micrOTOF-Q Daltonics (API) Time-of-Flight Mass Spectrometer (Bremen, Germany) coupled to 1200 series HPLC system (Agilent Technologies, Waldbronn, Germany). Chromatographic separation was carried out on a Superspher 100 RP-18 (75 × 4 mm i.d.; 4 μm) column (Merck, Darmstadt, Germany). The ionisation technique was an electrospray. The mass spectrometer was operated in the negative mode using the following settings: capillary voltage, 4000 V; end plate offset, −500 V; drying gas (N2) flow rate, 10 l/min and temperature, 200 °C. For MS/MS measurements, argon was used as a collision gas and the voltage over the collision cell varied from 20 to 70 eV. Sephadex LH-20 was purchased from Amersham Biosciences (Uppsala, Sweden). RP-C18 was obtained from Sigma-Aldrich GmbH, Germany and precoated silica gel TLC GF254 was purchased from Riedel-De Häen-AG, Seelze, Germany.
Chemicals
Bovine serum albumin, Dulbecco’s modified Eagle’s medium (DMEM), fetal bovine serum (FBS), penicillin/streptomycin solution and other cell culture reagents were purchased from Lonza (Basel, Switzerland). Reduced GSH and 5,5′-dithiobis-2-nitrobenzoic acid (Ellman’s reagent) were purchased from Sigma-Aldrich Chemical Company (St. Louis, MO). Sil 99% (Indena S.P.A, Milano, Italy) was kindly supplied by (Medical Union Pharmaceuticals Company, Cairo, Egypt). All other chemicals were of analytical grade and of the highest purity.
Plant material, extraction and isolation
The leaves of M. styphelioides were collected in July 2009 from Anshas Botanical Garden, El-sharkya, Egypt. The plant was botanically identified by Therese Labib, the taxonomy specialist at the herbarium of El-Orman Botanical Garden, Giza, Egypt. A voucher specimen of M. styphelioides was deposited at the herbarium of the Department of Pharmacognosy, Faculty of Pharmacy, Ain Shams University, Cairo, Egypt (ASU MSM2009). The isolation of ellagitannins and galloyl esters was carried out as described before (Al-Sayed et al. Citation2014). Briefly, air-dried powdered leaves of M. styphelioides (500 g) were extracted three times with 80% MeOH. The extract was concentrated and freeze-dried to obtain a dry powder, which was dissolved in absolute MeOH. The methanol-soluble part was concentrated and freeze-dried to obtain a dry powder 80 g (MSE). Column fractionation was performed with a portion of MSE (30 g) using a Sephadex LH-20 column (5 × 50 cm) by eluting with H2O followed by H2O–MeOH mixtures to yield seven major fractions (I–VII). Pedunculagin (220 mg) and pterocarinin A (107 mg) were purified by repeated CC of Fr III (eluted with 30% MeOH) over different Sephadex LH-20 columns using mixtures of H2O and MeOH. Fr IV (eluted with 40% MeOH) was repeatedly chromatographed over Sephadex LH-20 columns to obtain tellimagrandin I (103 mg). Fr V (eluted with 50% MeOH) was re-chromatographed over a Sephadex LH-20 column (5 × 50 cm) to yield casuarinin (1 g). Tellimagrandin II (5.6 mg) was obtained by repeated CC of Fr VI (eluted with 60% MeOH) over Sephadex LH-20. Fr VII (eluted with MeOH) was fractionated by Sephadex LH-20 CC, followed by purification over RP-18 CC to yield tetragalloylglucose (1.5 mg) and pentagalloyl glucose (4.5 mg).
Cell culture
HepG2 cell line was purchased from VACSERA (Giza, Egypt), and then maintained in the tissue culture facility (Faculty of Pharmacy, Ain Shams University, Cairo, Egypt). Cells were grown in DMEM supplemented with heat-inactivated FBS (10%), penicillin G (100 IU/ml) and streptomycin (100 μg/ml). Cells were maintained at 37 °C in a 5% CO2 atmosphere with 95% humidity.
In vitro assay for hepatoprotective activity
The hepatoprotective activity of the isolated compounds was tested in vitro at three concentrations (25, 50 and 100 μm). MSE and the standard hepatoprotective agent Sil were assayed at the concentrations (25, 50 and 100 μg/ml). A HepG2 monolayer culture after attachment was pretreated with the tested material for 1 h. An aliquot of 40 mm CCl4 in 0.05% dimethyl sulphoxide was added and incubation was continued for another 2 h. The supernatant medium and cell lysates were then collected and stored at −20 °C until analysis. The positive control was a set of cells maintained in culture medium and treated only with CCl4 (40 mm); while the negative control was a set of cells maintained in phosphate-buffered saline without CCl4. The levels of ALT, AST were assessed in the supernatant. The concentration of GSH and the activity of SOD were evaluated in the cell lysates using commercially available kits according to the manufacturer instructions (Biodiagnostics, Cairo, Egypt). Generally, the cells were collected and homogenised in PBS buffer (phosphate-buffered saline, pH 7.4) using a Dounce homogeniser (Fischer Scientific Co., Pittsburgh, PA), centrifuged at 4000 rpm for 15 min. Aliquots were then stored at −80 °C for the estimation of GSH content and SOD activity.
Biochemical analyses
ALT and AST activities were measured as reported before (Reitman & Frankel Citation1957). GSH was estimated by monitoring the reduction of Ellman’s reagent. The reduced chromogen is measured at 405 nm and is directly proportional to GSH concentration (Beutler et al. Citation1963). The SOD activity was determined according to the method of Nishikimi et al. (Citation1972). The assay relied on the ability of the enzyme to inhibit the phenazine methosulphate-mediated reduction of nitroblue tetrazolium dye.
Statistical analysis
Data are presented as the mean ± SEM. Comparisons were carried out using one-way analysis of variance followed by Tukey–Kramer’s test for post hoc analysis. Statistical significance was acceptable to a level of p < 0.05 while p < 0.01 was considered highly significant, and p <0.001 were considered extremely significant. All statistical analyses were performed using GraphPad InStat software, version 3.05 (GraphPad Software, La Jolla, CA). Graphs were plotted using GraphPad Prism software, version 5.00 (GraphPad Software).
Results
Fractionation of the methanol-soluble portion (MSE) obtained from the 80% methanol extract of M. styphelioides yielded compounds 1–7 (). All the compounds were previously identified (Al-Sayed et al. Citation2014) based on their UV, HRESI-MS/MS and NMR (1H, 13C, 1D-TOCSY, DQF-COSY, HSQC, HMBC) data as well as by comparing these data with those previously reported in the literature (Supplementary data).
Hepatoprotective effect
A significant increase in the activity of the serum enzymes ALT and AST (p < 0.001) was observed in the CCl4-treated group compared to the normal control groups ( and ). Most of the isolated compounds conferred a significant (p < 0.001) hepatoprotective effect and reduced the activity of ALT and AST at all the tested concentrations, compared to the CCl4-treated groups. Pedunculagin significantly ameliorated the CCl4-induced elevation in ALT and AST. The percentage decrease in the liver marker enzymes at the tested concentrations (100, 50 and 25 μm) of pedunculagin was 32, 32 and 30% for ALT and 48, 48 and 45% for AST, respectively, relative to the CCl4-treated groups ( and ). The levels of ALT and AST in all the groups treated with pedunculagin were non-significant from the normal control group as well as the Sil groups. Pterocarinin A produced 25, 17 and 13% decrease in ALT and 35, 23 and 17% decrease in AST, at the tested concentrations (100, 50 and 25 μm), respectively, when compared to the CCl4-treated group. The groups treated with 100, 50 and 25 μm of tellimagrandin I showed 42, 36 and 31% decrease in ALT and 47, 43 and 37% decrease in AST, relative to the CCl4-treated groups. Notably, all the tested concentrations of tellimagrandin I were more effective in reducing the ALT levels compared to Sil (). The levels of the hepatic enzymes in the groups treated with tellimagrandin I (100 and 50 μm) were not significantly different from the normal control and the Sil groups. The percentage decrease in the liver marker enzymes in the groups treated with tellimagrandin II was 38, 32 and 26% for ALT and 45, 40 and 34% for AST. The levels of AST in the groups treated with 100 and 50 μm of tellimagrandin II were comparable and non-significant to the normal control group. In addition, the levels AST and ALT enzymes at all the tested concentrations of tellimagrandin II were comparable to those in the Sil groups. Pretreatment of the cells with casuarinin produced 30, 26 and 22% decrease in ALT and 42, 38 and 32% decrease in AST. The level of AST in the group treated with 100 μm of casuarinin was non-significant to the normal control group. Similarly, tetragalloylglucose decreased the ALT levels by 21, 20 and 19% and the AST levels by 45, 38 and 36%, relative to the CCl4-treated groups. Pretreatment of HepG2 cells with pentagalloyl glucose significantly protected against CCl4-induced damage as evidenced from the reduced leakage of ALT in the medium by 30, 28 and 26%, while it reduced the leakage of AST by 45, 38 and 36%. The groups treated with the total extract MSE exhibited 21, 13 and 7% decrease in ALT and 13, 7 and 1% decrease in AST. Sil produced 37, 34 and 29% decrease in ALT leakage and 51, 48 and 41% decrease in AST leakage, at the tested concentrations, respectively.
Figure 2. Effect of pretreatment with isolated compounds, extract (MSE) and silymarin (Sil) on the ALT leakage from HepG2 cells challenged with CCl4. Data are expressed as the mean ± SEM, (n = 3). The experiment was done in triplicates. MSE and silymarin were tested in three concentrations (100, 50, 25 μg/ml). The isolated compounds were tested in three concentrations (100, 50, 25 μm). **p < 0.01 and ***p < 0.001; significantly different compared to the normal control group. ###p < 0.001; significantly different compared to the CCl4-treated group. $p < 0.05, $$p < 0.01 and $$$p < 0.001; significantly different compared to the silymarin-treated group. (Pedun, pedunculagin; Ptero, pterocarinin A; Tellim I, tellimagrandin I; Casuar, casuarinin; Tellim II, tellimagrandin II; TGG, tetragalloylglucose; PGG, pentagalloyl glucose).
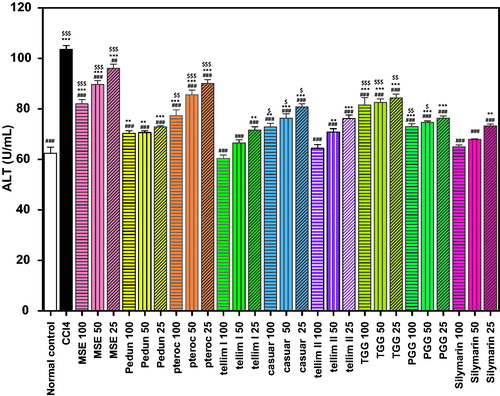
Figure 3. Effect of pretreatment with isolated compounds, extract (MSE) and silymarin (Sil) on the AST leakage from HepG2 cells challenged with CCl4. Data are expressed as the mean ± SEM, (n = 3). The experiment was done in triplicates. MSE and silymarin were tested in three concentrations (100, 50, 25 μg/ml). The isolated compounds were tested in three concentrations (100, 50, 25 μm). *p < 0.05, **p < 0.01 and ***p < 0.001; significantly different compared to the normal control group. ###p < 0.001; significantly different compared to the CCl4-treated group. $p < 0.05 and $$$p < 0.001; significantly different compared to the silymarin-treated group. (Pedun, pedunculagin; Ptero, pterocarinin A; Tellim I, tellimagrandin I; Casuar, casuarinin; Tellim II, tellimagrandin II; TGG, tetragalloylglucose; PGG, pentagalloyl glucose).
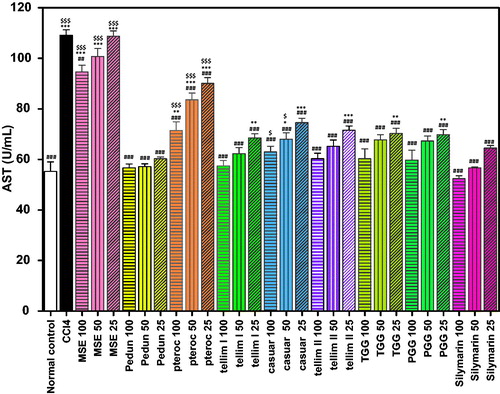
Antioxidant activity
The CCl4 group exhibited a significant decrease in the activity of GSH and SOD (p < 0.001) when compared to the normal control groups ( and ). Pedunculagin produced a significant antioxidant (p < 0.001) effect as evidenced from the increased levels of GSH and SOD at all the tested concentrations. The percentage increase in GSH and SOD levels at the tested concentrations (100, 50 and 25 μm) was 76, 66 and 59% for GSH and 497, 350 and 258% for SOD, respectively, compared to the CCl4-treated groups ( and ). The levels of SOD in the groups treated with 100 and 25 μm of pedunculagin were non-significant from the corresponding Sil groups. Pterocarinin A produced 49, 31 and 21% increase in GSH and 189, 64 and 43% in SOD, at all the tested concentrations, respectively. Interestingly, tellimagrandin I showed the highest antioxidant effect (p < 0.001) and significantly enhanced the GSH levels by 113, 105 and 81% and induced the SOD activity by 442, 348 and 119%, relative to the CCl4-treated groups ( and ). Additionally, pretreatment with tellimagrandin II induced 110, 103 and 79% increase in GSH and 411, 304 and 41% in SOD activity with respect to the CCl4-treated groups. The levels of the GSH in the groups treated with (100 and 50 μm) of tellimagrandin I and tellimagrandin II were not significantly different from the normal control. All the tested concentrations of tellimagrandin I and tellimagrandin II were non-significant to the corresponding Sil groups. Casuarinin produced 71, 65 and 55% increase in GSH and 489, 336 and 239% increase in SOD. The level of SOD in the group treated with 100 μm of casuarinin was non-significant to the corresponding Sil group. Tetragalloylglucose induced 30, 16 and 5% increase in GSH and 235, 73 and 40% increase in SOD. Pentagalloyl glucose induced a significant increase in both GSH and SOD. The increase in GSH and SOD levels at the tested concentrations of pentagalloyl glucose was 61, 57 and 54% for GSH and 465, 287 and 176% for SOD. The level of SOD in the group treated with 100 μm of pentagalloyl glucose was non-significant to that of the corresponding Sil group. Pretreatment of the cells with MSE showed 55, 36 and 26% increase in GSH and 186, 60 and 38% increase in SOD. Sil produced 120, 116 and 97% increase in GSH and 516, 450 and 324% increase in SOD, at the tested concentrations, respectively ( and ).
Figure 4. Effect of pretreatment with isolated compounds, extract (MSE) and silymarin (Sil) on GSH level in HepG2 cell lysate. Data are expressed as the mean ± SEM, (n = 3). The experiment was done in triplicates. MSE and silymarin were tested in three concentrations (100, 50, 25 μg/ml). The isolated compounds were tested in three concentrations (100, 50, 25 μm). **p < 0.01 and ***p < 0.001; significantly different compared to the normal control group. #p < 0.05, ##p < 0.01 and ###p < 0.001; significantly different compared to the CCl4-treated group. $$$p < 0.001; significantly different compared to the silymarin-treated group. (Pedun, pedunculagin; Ptero, pterocarinin A; Tellim I, tellimagrandin I; Casuar, casuarinin; Tellim II, tellimagrandin II; TGG, tetragalloylglucose; PGG, pentagalloyl glucose).
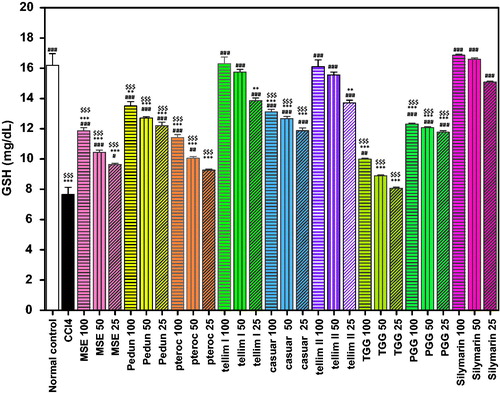
Figure 5. Effect of pretreatment with isolated compounds, extract (MSE) and silymarin (Sil) on SOD activity in HepG2 cell lysate. Data are expressed as the mean ± SEM, (n = 3). The experiment was done in triplicates. MSE and silymarin were tested in three concentrations (100, 50, 25 μg/ml). The isolated compounds were tested in three concentrations (100, 50, 25 μm). ***p < 0.001; significantly different compared to the normal control group. ###p < 0.001; significantly different compared to the CCl4-treated group. $p < 0.05, $$p < 0.01 and $$$p < 0.001; significantly different compared to the silymarin-treated group. (Pedun, pedunculagin; Ptero, pterocarinin A; Tellim I, tellimagrandin I; Casuar, casuarinin; Tellim II, tellimagrandin II; TGG, tetragalloylglucose; PGG, pentagalloyl glucose).
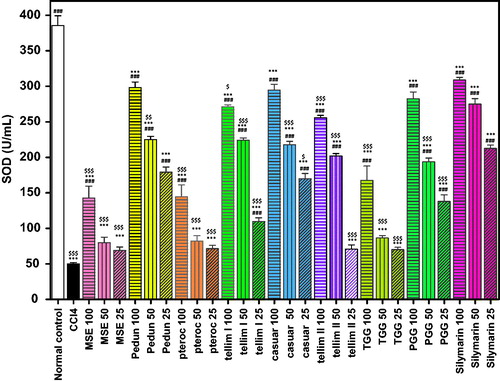
Discussion
The human hepatocellular carcinoma cell line (HepG2) is a suitable model to screen the hepatoprotective activity of drugs because it retains most of the functional characteristics of normal human hepatocytes (Knasmüller et al. Citation1998). CCl4 is a highly hepatotoxic chemical that is widely used in experimental models to induce liver damage that mimics human hepatotoxicity (Al-Sayed & Abdel-Daim Citation2014). The highly reactive trichloromethyl radicals (CCl3 and CCl3O2) are formed from CCl4 by cytochrome P-450. These radicals initiate lipid peroxidation and necrosis of the liver. The trichloromethyl radicals also devastate the cellular antioxidant defence system by deactivating GSH and antioxidant enzymes (Lee & Lim Citation2008; Al-Sayed et al. Citation2015). In this study, CCl4 treatment significantly increased the leakage of AST and ALT from the HepG2 cells into the culture medium as compared to the normal control. CCl4 treatment also induced severe oxidative stress as evidenced by the marked reduction in SOD and GSH levels. All the isolated compounds induced a significant (p < 0.001) hepatoprotective effect and reduced the elevated ALT and AST levels at a concentration-dependent manner, compared to the CCl4-treated groups. Tellimagrandin I exhibited the highest hepatoprotective effect and ameliorated the CCl4-induced elevation of ALT and AST, followed by pedunculagin, tellimagrandin II and pentagalloyl glucose, followed by tetragalloylglucose and casuarinin, while MSE and pterocarinin A showed the least hepatoprotective effects. All the tested concentrations of tellimagrandin I was more effective in reducing the ALT levels than Sil. Furthermore, tellimagrandin I and II were the most potent components in enhancing the GSH levels, followed by pedunculagin, casuarinin and pentagalloyl glucose. The GSH levels in the groups treated with tellimagrandin I and II were not significantly different from the normal control and Sil groups. Pedunculagin induced the highest SOD activity, followed by casuarinin and pentagalloyl glucose. While tellimagrandin I, tellimagrandin II and tetragalloylglucose moderately induced the SOD activity. Pterocarinin A and MSE showed the least antioxidant activity.
The results of this study indicated that tellimagrandin I significantly protected against CCl4-induced cellular damage, which is in accordance with previous studies reported by Shimoda et al. (Citation2008). In vitro studies in primary cultured rat hepatocytes revealed that ellagitannins having both hexahydroxydiphenoyl and galloyl groups, such as tellimagrandin I and II were more effective in protecting the hepatocytes from CCl4-induced damage (as evidenced by the reduced AST and ALT leakage) than pedunculagin, pentagalloyl glucose and casuarinin (Shimoda et al. Citation2008). However, in our study, pedunculagin and pentagalloyl glucose also showed a marked hepatoprotective effect, this may be due to the different experimental conditions between the two studies. Other studies showed that pentagalloyl glucose exhibited a cytoprotective effect and a strong inhibitory effect against lipid peroxidation (Okuda Citation2005; Piao et al. Citation2009), which supported our findings. Pentagalloyl glucose also showed strong radical scavenging activities and enhanced the levels of the antioxidant defences (Piao et al. Citation2009), which was also evident from the present study. Based on the findings of this study, the presence of a glucopyranose core, such as in tellimagrandin I and pedunculagin is essential for the hepatoprotective effect, pterocarinin A which has a ring-opened glucose unit along with a lyxose sugar exhibited a weak hepatoprotective activity, which was in accordance with previously reported studies (Shimoda et al. Citation2008). Casuarinin, which also has a ring-opened glucose unit, markedly induced the SOD activity but moderately reduced the leakage of ALT and AST, which suggested that the cytoprotective effect of these compounds involves other mechanisms than their antioxidant effects.
In conclusion, the present findings demonstrated that tellimagrandin I, II, pedunculagin and pentagalloyl glucose are the most active components in suppressing CCl4-induced cellular damage in HepG2. The pronounced hepatoprotective effect of these compounds might be partially mediated through their potent antioxidant effects. The ability of tellimagrandin I and II to markedly increase the GSH levels clearly contributed to their strong hepatoprotective effect. The ability of pedunculagin to induce the SOD activity might explain its hepatoprotective effect. Besides the antioxidant effects, the ability to reduce the leakage of the hepatic enzymes through stabilising the cellular membranes might also contribute to the strong hepatoprotective effect of these components. The present study warrants further detailed studies using different animal models and controlled clinical trials on the hepatoprotective potential of these components.
IPHB_1125933_Supplementary.docx
Download MS Word (15.3 KB)Supplementary_data.docx
Download MS Word (15.3 KB)Declaration of interest
The authors declare that there are no conflicts of interest.
References
- Al-Sayed E, Abdel-Daim MM. 2014. Protective role of Cupressuflavone from Cupressus macrocarpa against carbon tetrachloride-induced hepato- and nephrotoxicity in mice. Planta Med. 80:1665–1671.
- Al-Sayed E, El-Lakkany NM, Seif El-Din SH, Sabra AN, Hammam OA. 2014. Hepatoprotective and antioxidant activity of Melaleuca styphelioides on carbon tetrachloride-induced hepatotoxicity in mice. Pharm Biol. 52:1581–1590.
- Al-Sayed E, El-Naga RN. 2015. Protective role of ellagitannins from Eucalyptus citriodora against ethanol-induced gastric ulcer in rats: impact on oxidative stress, inflammation and calcitonin-gene related peptide. Phytomedicine 22:5–15.
- Al-Sayed E, Martiskainen O, Bobrowska-Hägerstrand M, Sinkkonen J, Törnquist K, Pihlaja K, Ayoub N, Singab A-N. 2010. Phenolic compounds from Eucalyptus gomphocephala with potential cytotoxic and antioxidant activities. Nat Prod Commun. 5:1639–1642.
- Al-Sayed E, Martiskainen O, Seif el-Din S, Sabra A-N, Hammam O, El-Lakkany N. (2015). Protective effect of Pelargonium graveolens against carbon tetrachloride-induced hepatotoxicity in mice and characterization of its bioactive constituents by HPLC–PDA–ESI–MS/MS analysis. Med Chem Res. 24:1438–1448.
- Beutler E, Duron O, Kelly BM. 1963. Improved method for the determination of blood glutathione. J Lab Clin Med. 61:882–888.
- Fahmy NM, Al-Sayed E, Abdel-Daim MM, Karonen M, Singab AN. 2016. Protective effect of Terminalia muelleri against carbon tetrachloride-induced hepato and nephro-toxicity in mice and characterization of its bioactive constituents. Pharm Biol. 54:303–313.
- Jacobs BP, Dennehy C, Ramirez G, Sapp J, Lawrence VA. 2002. Milk thistle for the treatment of liver disease: a systematic review and meta-analysis. Am J Med. 113:506–515.
- Knasmüller S, Parzefall W, Sanyal R, Ecker S, Schwab C, Uhl M, Mersch-Sundermann V, Williamson G, Hietsch G, Langer T, et al. 1998. Use of metabolically competent human hepatoma cells for the detection of mutagens and antimutagens. Mutat Res. 402:185–202.
- Lee SJ, Lim KT. 2008. Glycoprotein of Zanthoxylum piperitum DC has a hepatoprotective effect via anti-oxidative character in vivo and in vitro. Toxicol In Vitro. 22:376–385.
- Muriel P, Rivera-Espinoza Y. 2008. Beneficial drugs for liver diseases. J Appl Toxicol. 28:93–103.
- Nishikimi M, Appaji N, Yagi K. 1972. The occurrence of superoxide anion in the reaction of reduced phenazine methosulfate and molecular oxygen. Biochem Biophys Res Commun. 46:849–854.
- Okuda T. 2005. Systematics and health effects of chemically distinct tannins in medicinal plants. Phytochemistry 66:2012–2031.
- Piao MJ, Kang KA, Zhang R, Ko DO, Wang ZH, Lee KH, Chang WY, Chae S, Jee Y, Shin T, et al. 2009. Antioxidant properties of 1,2,3,4,6-penta-O-galloyl-β-d-glucose from Elaeocarpus sylvestris var. ellipticus. Food Chem. 115:412–418.
- Ramachandra Setty S, Quereshi AA, Viswanath Swamy AH, Patil T, Prakash T, Prabhu K, Veeran Gouda A. 2007. Hepatoprotective activity of Calotropis procera flowers against paracetamol-induced hepatic injury in rats. Fitoterapia 78:451–454.
- Reitman S, Frankel S. 1957. A colorimetric method for the determination of serum glutamic oxalacetic and glutamic pyruvic transaminases. Am J Clin Pathol. 28:56–63.
- Shimoda H, Tanaka J, Kikuchi M, Fukuda T, Ito H, Hatano T, Yoshida T. 2008. Walnut polyphenols prevent liver damage induced by carbon tetrachloride and d-galactosamine: hepatoprotective hydrolyzable tannins in the kernel pellicles of walnut. J Agric Food Chem. 56:4444–4449.
- Srivastava A, Shivanandappa T. 2010. Hepatoprotective effect of the root extract of Decalepis hamiltonii against carbon tetrachloride-induced oxidative stress in rats. Food Chem. 118:411–417.
- Yoshida T, Ito H, Yoshimura M, Miyashita K, Hatano T. 2008. C-Glucosidic ellagitannin oligomers from Melaleuca squarrosa Donn ex Sm., Myrtaceae. Phytochemistry 69:3070–3079.