Abstract
Context Ginseng is a widely used herbal medicine in China but its mechanism of action remains unclear.
Objective The objectives of this work were to study the protective effect of ginsenoside Rg1 on subacute murine renal damage induced by d-galactose and its mechanism.
Materials and methods C57BL/6J mice were injected with 120 mg/kg/d (sc) d-galactose for 1 week, followed by a combined treatment of Rg1 20 mg/kg/d (ip) and 120 mg/kg/d d-galactose (sc) for 5 weeks. Mice were injected with the 0.9% saline 0.2 mL/d (sc) and 120 mg/kg/d d-galactose (sc) for 6 weeks in the control group and the d-galactose group, respectively. After 6 weeks, urea, creatinine, uric acid, cystatin (Cys-C), senescence-associated β-galactosidase (SA-β-gal) staining positive kidney cells, superoxide dismutase (SOD), glutathione peroxidase (GSH-PX), malondialdehyde (MDA), glycation end products (AGEs) and 8-hydroxy-2 deoxyguanosine (8-OH-dG) were measured.
Results Treatment with Rg1 ameliorated kidney function and aging state (urea from 17.19 ± 1.09 to 15.77 ± 1.22 mmol·L − 1, creatinine from 29.40 ± 5.72 to 22.60 ± 3.97 μmol·L − 1, uric acid from 86.80 ± 5.97 to 72.80 ± 10.61 μmol·L − 1, Cys-C from 0.23 ± 0.03 to 0.18 ± 0.05 mg·L − 1, ROD of SA-β-gal from 56.32 ± 10.48 to 26.78 ± 7.34, SOD from 150.22 ± 19.07 to 190.56 ± 15.83 U·(mg·prot) −1, MDA from 9.28 ± 1.59 to 3.17 ± 0.82 nmol·(mg·prot) −1, GSH-PX from 15.68 ± 2.11 to 20.32 ± 2.96 U·(mg·prot) −1 as well as regulated glomerulus morphology (glomerulus diameter from 775.77 ± 18.41 to 695.04 ± 14.61 μm, renal capsule width from 39.56 ± 3.51 to 31.42 ± 2.70 μm, glomerulus basement membrane from 206.03 ± 16.22 to 157.27 ± 15.70 nm, podocyte slit from 55.21 ± 8.55 to 37.63 ± 6.65 nm).
Conclusions Ginsenoside Rg1 can antagonise d-galactose subacute renal damage in mice and this may occur due to alleviating oxidative stress injury.
Introduction
Kidneys, critical for fluid and electrolyte homeostasis, excrete water-soluble metabolites, restore acid–base balance and secrete bioactive substances. These functions plus the large blood supply directed to the kidney make it vulnerable to damage. Panax ginseng (Li and Li Citation1973) has been used as a tonic drug in Chinese medicine for more than 2000 years. Ginseng, a well-known herbal medicine, contains chiefly Rg1 which has been suggested to have antioxidant, anti-aging, and immune and memory improvement properties (Cheng et al. Citation2005; Chen et al. Citation2008). Our work suggests that Rg1 can prevent cognitive impairment and hippocampal senescence as well as enhance the resistance of haematopoietic stem/progenitor cells to what (Chen et al. Citation2014; Zhu et al. Citation2014). Other studies indicate that ginsenoside Rg1 can be used to treat kidney disease (Xie et al. Citation2008; Zhang et al. Citation2011; Mao et al. Citation2014). Thus, Rg1 may be able to protect the kidney against injuriousoxidation. We established a d-galactose-induced injury mouse model to investigate any protective effects of this isolate and to study its potential mechanism of action.
Materials and methods
Animals
Male C57BL/6J mice (19–21 g, 6–8 weeks-of-age) were purchased from the Medical and Laboratory Animal Center of Chongqing (qualified number is SCXK yu. 2012-0001) and housed at 20–25 °C under a 12:12 h light:dark cycle with free access to water and food. All experiments were performed according to the Regulations of Experimental Animal Administration issued by the State Committee of Science and Technology of the People’s Republic of China and were approved by the Ethics Committee of Chongqing University (CQU-2009011).
Reagents
Ginsenoside Rg1 (purity > 98%) was purchased from Hongjiu Biological Technology Co, Ltd. (Tonghua, China); d-galactose (purity ≥ 99%) was obtained from Sangon Biotech Co, Ltd. (Shanghai, China). The AGE and 8-OH-dG kits were purchased from Shanghai Yuanye Biological Technology Co, Ltd. (Shanghai, China). The GSH-PX, MDA and SA-β-gal assay systems were obtained from Beyotime Institute of Biotechnology (Shanghai, China). The SOD assay was purchased from Nanjing Jiancheng Bioengineering Institute (Nanjing, China).
Animal treatment
Thirty animals were randomly divided into three groups (N = 10) In the d-galactose group, mice were given d-galactose (120 mg/kg per day, sc) for 6 weeks (Zhu et al. Citation2014). In the Rg1 + d-galactose group, at the 8th day d-galactose injection and onward, mice were treated with Rg1 (20 mg/kg, per day, i.p.). For controls, all animals were given 0.9% saline (s.c.) 0.2 mL per day. On the 43rd day, animals were sacrificed and analyzed.
Biochemical determination
Mouse sera was centrifuged (3000 rpm, 10 min) after being obtained from the retro-orbital plexus and urea, creatinine, uric acid and Cys-C, which are proxies for renal function were analyzed by the Department of Laboratory Medicine, The Hospital Group of the First Affiliated Hospital of CQMU of Traditional Chinese Medicine (Chongqing, China).
Histological analysis
We used fresh mouse renal tissue and fixed them in a fresh solution of 4% paraformaldehyde (pH 7.4) for 24 h, dehydrated in graded (50–100%) alcohol and embedded in paraffin. Sections (5 μm) were made and these were stained with haematoxylin and eosin (H&E) and examined under light microscopy to study tissue pathology. Image-Pro Plus 6.0 (Media Cybernetics Inc., Atlanta, GA) was used to measure the glomerular diameter, the renal capsule width and normal and sclerosing glomeruli were counted.
Senescence-associated β-galactosidase cytochemical staining
Some tissue sections were deparaffinized and SA-β-gal staining was carried out according to the instructions of the manufacturer. Sections were washed in PBS after incubation and after sections were sealed, they were viewed under a bright field at 400 × magnification. To analyse images, at least three random fields were counted by blinded observers. SA-β-gal-positive cell staining intensity was evaluated with ROD values that were obtained after transformation of mean grey values into RODs using the formula: ROD = log (256/mean grey). Images were collected from at least three different sections per structure and per animal by Image Pro Plus software (Media Cybernetics Inc., Atlanta, GA).
Ultrastructural observation
Fresh tissue samples from each group were fixed with 2.5% glutaraldehyde for 4 h and then washed with PBS three times. Sections were fixed with 1% osmium tetroxide acid, gradient dehydrated in alcohol and embedded in Epon812. Ultra-thin sections were cut and stained with uranium acetate/lead nitrate electronics and visualized using H-600IV transmission electron microscopy (Bio-Rad Laboratories Inc., Berkeley, CA). To analyze images, 10 random podocyte foot process slits were counted by a blinded observer in each imagine using Image Pro Plus software (Media Cybernetics Inc., Atlanta, GA).
Measurement of oxidation-associated biomarkers
Kidneys were promptly dissected and perfused with 50 mM (pH 7.4) in an ice bath with PBS were homogenized in PBS containing a protease inhibitor, creating 10% kidney tissue homogenates. Supernatant was collected after centrifugation (12 000 rpm, 4 °C, 30 min). GSH-PX and SOD activities and MDA content were measured with colorimetric analysis according to the manufacturer’s instructions. GSH-PX, SOD activity and MDA content were at 340, 450 and 532 nm, respectively.
Measurements of AGEs and 8-OH-dG in kidney tissues by ELISA
Supernatant was collected as described above, and AGEs and 8-OH-dG were measured in the kidney by ELISA according to the manufacturer’s instructions.
Statistical analysis
Data are presented as means ± SD. Significant differences between groups was confirmed with ANOVA. p < 0.05 was considered statistically significant. All results were analyzed using SPSS 17.0 statistical software (SPSS Inc., Chicago, IL).
Results
The effect of Rg1 on renal function in d-galactose-induced mice
Urea, creatinine, uric acid and Cys-C were used to monitor renal damage and these were measured after experimental treatments. Urea, creatinine, uric acid and Cys-C were significantly increased in the d-galactose group; however, Rg1 reduced renal injury induced by d-galactose ().
Table 1. Effect of Rg1 on the kidney function of d-galactose model mice (x ± s, n = 10).
Rg1 and histological changes in d-galactose-induced renal injury
Histology was used to investigate the effect of Rg1 on d-galactose-induced injury. and , and show that d-galactose treatment damaged kidneys compared with the control group. Glomeruli decreased and sclerosing glomeruli increased as did their diameter. Renal capsules expanded, too. Rg1 was protective against d-galactose-induced kidney injury, as evidenced by more normal glomeruli fewer damaged and dilated glomeruli and less renal capsule expansion.
Figure 1. Effect of Rg1 on the kidney structure of d-galactose model mice (HE stain, ×100). ▴: glomerulus.
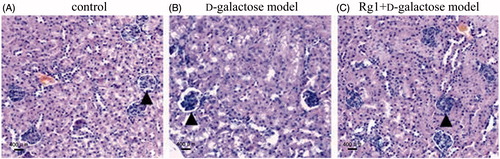
Figure 2. Effect of Rg1 on the structure of kidney glomerulus of d-galactose model mice (HE stain, ×400).
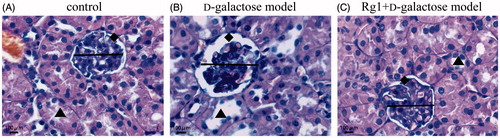
Table 2. Effect of Rg1 on the structure of glomerulus of d-galactose model mice (x ± s, n = 10).
Rg1 and SA-β-gal in d-galactose-induced renal injury
SA-β-gal is a common biomarker for aging cells for which the cytoplasm stains blue. SA-β-gal-positive cells were not observed in glomerular areas but were seen in tubular areas (Ding et al. Citation2001). shows that SA-β-gal-positive cells staining intensity as evaluated by RODs were greater compared to controls. However, in the Rg1 + d-galactose model group, RODs were significantly reduced (and ). Thus, Rg1 can protect the kidney against senescence.
Figure 3. Effect of Rg1 on the kidney cells senescence of d-galactose model mice (SA-β-Gal stain × 400).
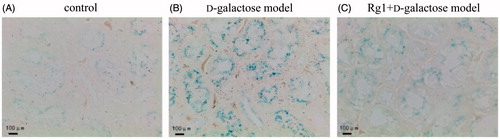
Table 3. Effect of Rg1 on the SA-β-gal staining of d-galactose model mice (x ± s, n = 10).
Rg1 on the glomerular ultrastructure of d-galactose induced renal damage
To investigate glomerular ultrastructure, we used transmission electron microscopy to observe morphological changes induced by d-galactose. In controls, glomerular structures were normal. In the d-galactose group, glomerular basement membranes were thick and uneven, podocyte slits were wider, and some podocyte cell processes were fused, and ribosomes and rough endoplasmic reticuli in the podocyte were decreased compared with the normal group. After Rg1 treatment for 35 d, glomerular ultrastructural damages were reversed ( and ).
Table 4. Effect of Rg1 on the thickness of glomerulus basement membrane and slit of podocyte of d-galactose model mice (x ± s, n = 10).
Antioxidant effect of Rg1 on renal damage induced by d-galactose
GSH-PX and SOD are responsible for ROS degeneration and subsequent senescence. MDA, a product of ROS, can serve as an oxidative stress biomarker. Thus, by measuring the GSH-PX, SOD activity and MDA, we estimated antioxidant ability and oxidative damage in d-galactose-treated groups. Compared with controls, GSH-PX and SOD activities were less and MDA was greater in the d-galactose group. Meanwhile, Rg1 partially rescued reduced GSH-PX, SOD activity and MDA decreased in the Rg1+ d-galactose group ().
Table 5. Effect of Rg1 on the level of SOD, MDA and GSH-PX in the kidney of d-galactose model mice (x ± s, n = 10).
Rg1 and 8-OH-dG and AGEs in d-galactose-induced renal damage
8-OH-dG is an oxidative DNA lesion formed by the oxidation of the C-8 position of 20-deoxyguanosine, and it is a commonly used biomarker of oxidative DNA damage (Singh et al. Citation2007). Advanced glycation end products (AGEs) have major roles in kidney disease (Noordzij et al. Citation2008). AGEs are glycated moieties that bind to proteins as well as lipids and nuclear acids. AGEs form during glycation and oxidative stress (Baynes Citation1991). AGEs and 8-OH-dG in the d-galactose group were increased compared with controls. Interestingly, Rg1 reduced AGEs and 8-OH-dG ().
Discussion
Ageing is characterized by a decline in renal functional reserve, so the elderly are more susceptible to renal failure (Esposito and Dal Canton Citation2010). Furthermore, aldosterone (Mao et al. Citation2014), alcohol (Rodrigo et al. Citation2002), lipopolysaccharide (Ueki et al. Citation2007) and other drugs that induce renal injury may be difficult to repair or treat, so a renal therapeutic would have enormous clinical value. Ginseng, a well-known herbal, may be useful for treating kidney diseases in China (Yan et al. Citation2009) but how it works is uncertain. Here, we suggest that ginsenoside Rg1 treatment may protect the kidney against abnormalities in a well-characterized aging rat model using d-galactose to induce renal damage.
d-Galactose-induced mouse kidney aging is related to the accumulation of ROS, or the stimulation of free radical production indirectly via AGEs formation in vivo, and this can mimic normal aging in rats (Ho et al. Citation2003; Zhang et al. Citation2005). ROS accumulation and free radicals can damage kidney function during normal aging processes and mimicking this aging with d-galactose offers a reasonable model for study (Aydın et al. Citation2012). Thus, we used d-galactose to damage mouse kidneys and treated this damage with Rg1. Experimental data from transmission electron microscopy indicated that SA-β-gal staining was increased, and these data agree with other studies showing that positively stained cells were significantly increased after d-galactose administration (Chen et al. Citation2014; Zhu et al. Citation2014). Also, SOD and GSH-PX activities increased and MDA decreased as did AGEs, 8-OH-dG which are markers of renal damage.
Urea, creatinine, uric acid and Cys-C (Lyman Citation1986; Jovanović et al. Citation2003) are common renal function markers that increase with damage. After inducing renal damage and treating mice with Rg1 for 35 d, all renal damage markers were reduced, indicating that Rg1 could reverse renal injury (Zhang et al. Citation2011; Mao et al. Citation2014). Furthermore, daily injection of Rg1 to the d-galactose-induced aging mice showed that SA-β-gal staining was significantly decreased. SA-β-gal, which reflects lysosomal function, accumulates in aging cells as lysosomes begin to malfunction, so it is used to evaluate senescence. These data agreed with previous reports that Rg1 could slow or prevent senescence in a rat model (Chen et al. Citation2014; Zhu et al. Citation2014). Administration of Rg1 to d-galactose-damaged mice kidneys attenuated damage as evidenced by histopathology and ultrastructural data. Renal cell senescence or injury can inevitably affect glomerular filtration rate and endothelial function, resulting in renal failure. Our data agreed with published findings that renal cell senescence or injury can inevitably affect glomerular filtration rate and endothelial function, resulting in renal failure (Bonegio and Lieberthal Citation2002). Among the three intrinsic glomerular cells, the podocyte provides an important component of the filtration barrier. We presume that incremental production of d-galactose in the podocyte may contribute to ongoing oxidative stress, and Rg1 treatment may decrease it.
ROS oxidation is the main cause of senescence, but senescence can be postponed by ROS scavengers (Lopez-Torres and Barja Citation2008). ROS include superoxide anion radical (O2−) and hydroxyl radical (·OH−), as well as non-radical oxidants, such as hydrogen peroxide (H2O2) and singlet oxygen (1O2) (Zorov et al. Citation2014). The ability of D-galactose to induce aging may be via ROS and subsequent damage. Indeed, we observed that SOD and GSH-PX activities decreased likely as they managed ROS damage. Also, MDA, which is formed from ROS, increased in response to d-galactose-induced senescence and decreased after injection of Rg1. Thus, Rg1 may partially rescue SOD and GSH-PX function and decrease MDA. These effects may offer preliminary data about the mechanism by which Rg1 is renally protective. AGEs formed by free amines of amino acids in proteins and peptides in vivo can cause accumulation of ROS (Fan et al. Citation2009; Liu et al. Citation2010). That AGEs decreased after Rg1 treatment may suggest that Rg1 could protect the mouse kidney by abating oxidative stress this way. ROS may also damage DNA, proteins and lipids within cells and cause tissue injury (Wu et al. Citation2008). Renal 8-OH-dG, a biomarker for DNA damage, was also decreased in mice treated with Rg1 compared with those injured with d-galactose. Thus, Rg1 may protect against DNA damage by attenuating oxidative stress and this agrees with previous data (Kim et al. Citation2014).
In conclusion, ginsenoside Rg1 treatment may delay renal dysfunction or reverse damage as evidenced in a mouse model of d-galactose-induced aging. Further studies are required to confirm these data, but they hold enormous promise for the future of kidney toxicology and pharmacology.
Declaration of interest
The authors report that they have no conflicts of interest.
References
- Aydin S, Yanar K, Atukeren P, Dalo E, Sitar ME, Uslu E, Caf N, Çakatay U. 2012. Comparison of oxidative stress biomarkers in renal tissues of D-galactose induced, naturally aged and young rats. Biogerontology 13:251–260.
- Baynes JW. 1991. Role of oxidative stress in development of complications in diabetes. Diabetes. 40:405–412.
- Bonegio R, Lieberthal W. 2002. Role of apoptosis in the pathogenesis of acute renal failure. Curr Opin Nephrol Hypertens. 11:301–308.
- Chen C, Mu XY, Zhou Y, Shun K, Geng S, Liu J, Wang JW, Chen J, Li TY, Wang YP. 2014. Ginsenoside Rg1 enhances the resistance of hematopoietic stem/progenitor cells to radiation-induced aging in mice. Acta Pharmacol Sin. 35:14–3150.
- Chen C, Mu XY, Zhou Y, Shun K, Geng S, Liu J, Wang JW, Chen J, Li TY, Wang YP. 2008. Ginsenoside Rg1 delays tertbutyl hydroperoxide-induced premature senescence in human WI-38 diploid fibroblast cells. J Gerontol A Biol Sci Med Sci. 63:253–264.
- Cheng Y, Shen LH, Zhang JT. 2005. Anti-amnestic and anti-aging effects of ginsenoside Rg1 and Rb1 and its mechanism of action. Acta Pharmacol Sin. 26:143–149.
- Ding G, Franki N, Kapasi AA, Reddy K, Gibbons N, Singhal PC. 2001. Tubular cell senescence and expression of TGF-beta1 and p21(WAF1/CIP1) in tubulointerstitial fibrosis of aging rats. Exp Mol Pathol. 70:43–53.
- Esposito C, Dal Canton A. 2010. Functional changes in the aging kidney. J Nephrol. 15:S41–S45.
- Fan SH, Zhang ZF, Zheng YL, Lu J, Wu DM, Shan Q, Hu B, Wang YY. 2009. Troxerutin protects the mouse kidney from D-galactose-caused injury through anti-inflammation and anti-oxidation. Int Immunopharmacol. 9:91–96.
- Ho SC, Liu JH, Wu RY. 2003. Establishment of the mimetic aging effect in mice caused by D-galactose. Biogerontology 4:15–18.
- Jovanović D, Krstivojević P, Obradović I, Durdević V,Dukanović L. 2003. Serum cystatin C and beta2-microglobulin as markers of glomerular filtration rate. Ren Fail. 25:123–133.
- Kim MH, Seo JY, Liu KH, Kim JS. 2014. Protective effect of Artemisia annua L. extract against galactose-induced oxidative stress in mice. PLoS One. 9:e101486.
- Li CP, Li RC. 1973. An introductory note to ginseng. Am J Chin Med (Gard City N Y). 1:249–261.
- Lyman JL. 1986. Blood urea nitrogen and creatinine. Emerg Med Clin North Am. 4:223–233.
- Lopez-Torres M, Barja G. 2008. Calorie restriction, oxidative stress and longevity. Rev Esp Geriatr Gerontol. 43:252–260.
- Liu CM, Ma JQ, Lou Y. 2010. Chronic administration of troxerutin protects mouse kidney against D-galactose-induced oxidative DNA damage. Food Chem Toxicol. 48:2809–2817.
- Mao N, Cheng Y, Shi XL, Wang L, Wen J, Zhang Q, Hu QD, Fan JM. 2014. Ginsenoside Rg1 protects mouse podocytes from aldosterone-induced injury in vitro. Acta Pharmacol Sin. 35:513–522.
- Noordzij MJ, Lefrandt JD, Smit AJ. 2008. Advanced glycation end products in renal failure: an overview. J Ren Care. 34:207–212.
- Rodrigo R, Trujillo S, Bosco C, Orellana M, Thielemann L, Araya J. 2002. Changes in (Na + K)-adenosine triphosphatase activity and ultrastructure of lung and kidney associated with oxidative stress induced by acute ethanol intoxication. Chest. 121:589–596.
- Singh R, Sram RJ, Binkova B, Kalina I, Popov TA, Georgieva T, Garte S, Taiolif E, Farmer PB. 2007. The relationship between biomarkers of oxidative DNA damage, polycyclic aromatic hydrocarbon DNA adducts antioxidant status and genetic susceptibility following exposure. Mutat Res. 620:83–92.
- Ueki M, Taie S, Chujo K, Asaga T, Iwanaga Y, Ono J, Maekawa N. 2007. Urinary trypsin inhibitor reduces inflammatory response in kidney induced by lipopolysaccharide. J Biosci Bioeng. 104:315–320.
- Wu DM, Lu J, Zheng YL, Zhou Z, Shan Q, Ma DF. 2008. Purple sweet potato color repairs D-galactose-induced spatial learning and memory impairment by regulating the expression of synaptic proteins. Neurobiol Learn Mem. 90:19–27.
- Xie XS, Liu HC, Zuo C, Deng Y, Fan JM. 2008. The effect of ginsenoside-Rg1 on the renal interstitial fibrosis of UUO rat. Sichuan Da Xue Xue Bao Yi Xue Ban. 39:218–222.
- Yan X, Li YL, Zhao RS, Wu GD, Wang R, Li B, Wang Y, Zeng MJ, Wu C, Xu K, et al. 2009. Renal protective effect of ginsenoside against acute renal failure and nitronergic mechanism in PCT. J Dalian Med Univ. 31:279–282.
- Zhang MH, Fan JM, Xie XS, Deng YY, Chen YP, Zhen R, Li J, Cheng Y, Wen J. 2011. Ginsenoside-Rg1 protects podocytes from complement mediated injury. J Ethnopharmacol. 137:99–107.
- Zhang Q, Li X, Cui X, Zuo P. 2005. D-Galactose injured neurogenesis in the hippocampus of adult mice. Neurol Res. 27:552–556.
- Zhu JH, Mu XY, Zeng J, Xu CY, Liu J, Zhnag M, Li C, Chen J, Li T, Wang Y. 2014. Ginsenoside Rg1 prevents cognitive impairment and hippocampus senescence in a rat model of D-galactose-induced aging. PLoS One. 9:e101291.
- Zorov DB, Juhaszova M, Sollott SJ. 2014. Mitochondrial reactive oxygen species (ROS) and ROS-induced ROS release. Physiol Rev. 94:909–950.