Abstract
Context Oxidative stress induces apoptosis within Islets of Langerhans in diabetes mellitus (DM). Enicostemma littorale blume, herb of the Gentianaceae family is used as an anti-diabetic agent across rural India.
Objective This report demonstrates potent anti-apoptotic and cyto-protective activity of Enicostemma littorale MeOH extract (EL MeOH ext.) against 50 μM H2O2 in isolated rat Islets.
Materials and methods In this study, the whole plant methanolic extract of EL with doses 0.25–4 mg/mL each for the preincubation duration of 0.5–4 h against 50 μM H2O2 were tested for optimum protective dose and time by Trypan blue dye exclusion assay. Islet intracellular reactive oxygen species (ROS) was quantified by DCFDA staining and cell death using PS/PI & FDA/PI staining. Further, comet assay, biochemical assessment of caspase-3 and antioxidant enzyme activities along with immunoblotting of PARP-1, caspase-3, TNF-α activation and p-P38 MapK (stress kinase) induction was performed.
Results The optimized dose of EL MeOH ext. 2 mg/mL for 2 h was used throughout the study, which significantly decreased total Intracellular ROS and cell death. Further, caspase-3 activity, PARP-1 cleavage, p-P38 MapK (stress kinase) activation and TNF-α levels, which had been significantly elevated, were normalized. Antioxidant enzymes like catalase, superoxide dismutase, reduced glutathione and glutathione peroxidase, along with Comet assay, demonstrated that pretreatment with EL MeOH ext. can augment antioxidant enzyme activities and protect from DNA damage.
Discussion and conclusions Significant anti-apoptotic and cyto-protective effects were mediated by EL with Islets of Langerhans subjected to oxidative stress-induced cell death.
Introduction
Reactive oxygen species (ROS) are constantly generated under normal metabolic conditions, as a consequence of aerobic metabolism. They are particularly transient species due to their high chemical reactivity and can react with DNA, proteins, carbohydrates and lipids in a destructive manner which leads to various pathophysiological diseases, including diabetes, Parkinson's, Alzheimer, and retinal degeneration (Bonnefont-Rousselot Citation2002; Valko et al. Citation2007).
Glucose toxicity, leading to chronic hyperglycaemia in diabetes, involves oxidative stress as a major regulatory mechanism for inducing progressive β-cell loss and various metabolic complications such as insulin resistance. Pancreatic islets are very sensitive to oxidative stress since they express very low amount of anti-oxidant enzymes, like superoxide dismutases (SOD-1, SOD-2), catalase and glutathione peroxidise (GPx) (Grankvist et al. Citation1981; Tiedge et al. Citation1997). GSH is the major intrinsic antioxidant in cells. It has been reported, that long-term exposure to high glucose concentration decreases GSH level (Catherwood et al. Citation2002). Many clinical studies have documented chronic oxidative stress in type 2 diabetes generating excessive ROS causing islet cell death (Sakuraba et al. Citation2002; Padgett et al. Citation2013).
In type-1 diabetes, β-cell mass is strictly reduced by 70–80% at an early stage, unlike type-2 diabetes where, the same occurs at relatively slower and gradual pace. However, because of the variable degree of insults and absence of detectable β-cell necrosis, it is suggested that β-cell loss occurs slowly over years (Kloppel et al. Citation1985). These histopathological findings are in line with the progressive decline in first-phase insulin secretion in antibody-positive individuals, long before the development of overt diabetes (Srikanta et al. Citation1983). Further, it has also been later shown that βcell apoptosis causes a gradual β-cell depletion in rodent models of type-2 diabetes. Activated macrophages secrete cytokines which are responsible for β-cell apoptosis, probably mediated by three main pathways – namely JNK, ER stress, and liberation of pro-apoptotic proteins from the mitochondria (Kutlu et al. Citation2003).
In type 2 diabetic subjects, initial histopathologic studies suggested β-cell loss by 25–50% (Kloppel et al. Citation1985; Clark et al. Citation1988), but this was debated by others (Guiot et al. Citation2001). A few earlier studies which match diabetic patients and control subjects for BMI, showed a significant reduction in β-cell mass and around a three-fold increase in β-cell apoptosis (Sakuraba et al. Citation2002; Butler et al. Citation2003). These observations suggest that β-cell mass is decreased in type 2 diabetes, secondary to increased rates of β-cell apoptosis, but it remains unclear whether this explains the observed functional loss (Kahn Citation2003). β-Cell apoptosis may thus be a common feature of both type 1and type 2 diabetes.
Natural flavonoids are emerging as potent therapeutic drugs for free radical-mediated diseases. Enicostemma littorale (EL), a herb of the Gentianaceae family, contains diversified phytochemicals: some of the important constituents of the plant include betulin, a triterpenoid sapogenin, swertiamarin, a secoiridoid glycoside, monoterpene alkaloids like enicoflavine, swertisin, a flavonoid and gentiocrucine (Saranya et al. Citation2013). Also, as described by Ambikapathy et al. (Citation2011), some of the major components of the EL MeOH ext. are laminaribiitol (79.93%), 12-hydroxy-9-octadecenoic acid (9.546%), myricetin (4.7519), 3.3-methylenebis (4-hydroxycoumarin) (2.811). Cabechin (2.002) and the total phenolic content have been found to be approximately 5–6 mg gallic acid equivalents (GAE)/g DW (dry weight) (Abirami & Gomathinayagam Citation2013). Extract of the plant and its major phytochemical swertiamarin have been reported as antioxidant candidates (Vaijanathappa & Badami Citation2009). EL is known to have good antioxidant activity in diabetic rats (Maroo et al. Citation2003) as well as in newly diagnosed NIDDM patients (Vasu et al. Citation2003).
As mentioned above, our previous lab studies demonstrated a series of beneficial effects of EL on diabetic patients as well as in diabetic rat model. Hence, in this current study we wanted to further explore the cyto-protective potential of EL on oxidative stress-induced DNA damage and apoptosis in Islets of Langerhans.
Materials and methods
Animals
Adult virgin male rats of Charles-Foster strain weighing 250–300 g aged 6–8 weeks were kept under controlled conditions of light and temperature. They had access to food and water ad libitum. The experimental studies were performed after the approval from Committee for the Purpose of Control and Supervision of Experiments on Animals (CPCSEA) of Department of biochemistry, The M. S. University of Baroda and all applicable guidelines for the care and use of animals were followed.
Plant material and preparation of methanolic extract
Whole dried plant was procured from Saurashtra district in Gujarat state, India, in August 2013 after authentication from taxonomist Prof. S. D. Sabnis with voucher specimen number Oza 51, 51 (a) present in the Herbarium, Department of Botany, The M.S University of Baroda, Vadodara, Gujarat, India and all the applicable guidelines for the plant studies were followed. Whole dried plant was used for extract preparation. Plant was cut into small pieces and extracted with petroleum ether for 12 h in a Soxhlet apparatus. Residues were again extracted with methanol for 24 h. After the extraction, methanol was recovered by distillation and remaining traces of methanol were completely removed by keeping methanol extract at 60 °C for 4 days (Maroo et al. Citation2003; Vasu et al. Citation2003).
Chemicals
All chemicals and culture media used in this study were purchased from Sigma Aldrich, Bangalore, India. The details of the antibodies used are given in . Molecular biology reagents and cDNA and PCR kits were procured from Fermentas/Thermo Fisher Scientific, Bangalore, India.
Table 1. List of antibodies along with other details.
Islet isolation and treatment
Islets were isolated from healthy non-diabetic rats by the method of Xia and Laychock (Citation1993), and then subjected to purification. Here, maintaining sterile conditions, islets were first excised out and thoroughly minced. The minced tissue was collected and given a wash with cold phosphate buffer saline (PBS). The tissue was introduced into a 5 ml Hank’s balanced salt solution (HBSS) containing 5 mg collagenase type V. Digestion was carried out for 15 min in a shaking water bath at 37 °C. The digestate was diluted with cold PBS. The digested tissue was allowed to settle and washed thrice. Islets in the digestate were handpicked using Pasteur pipette under dissecting microscope (approx. 1000 islets/group). Purified islets were incubated with cumene H2O2 at a dose of 50 μM for 30 min then centrifuged at 400g for 10 min, washed twice with PBS and used for measurement of different parameters. Another group of islets was pre-incubated with 2 mg/ml EL MeOH ext. for 2 h, washed with PBS and then subjected to 50 μM H2O2 exposure. This treatment of EL MeOH ext. and H2O2 was uniformly maintained throughout the study. In vitro dose (five doses) and time (four time points) dependent study was carried out with extract. The viability of the islets was checked by trypan blue dye exclusion test using 0.4% (W/V) trypan blue (Shewade et al. Citation2001). Blue stained islets were scored as non-viable and the unstained were scored as viable islets.
Assessment of cell death by PS/PI dual staining
One hundred islets of Langerhans were pelleted and washed twice with PBS. To differentiate between apoptotic and necrotic cell death, dual staining with PS (Annexin V-FITC)/PI (propidium iodide) was performed as described by Rieger et al. (Citation2011). Fluorescence was monitored at 40× magnification using Confocal microscope (LSM, 710 Zeiss, Bangalore, India). The relative islet fluorescence intensity was measured by Image J software (NIH, Bethesda, MD) and then plotted.
Assessment of intracellular oxidative stress using DCFDA (2′,7′–dichlorofluoresceindiacetate) dye
One hundred islets were handpicked and pelleted by centrifuging at 400g for 3 min in a 1.5 mL micro centrifuge tube. Media was aspirated and these islets were washed with PBS and re-suspended in the 990 μL PBS along with 10 μL of 10 μg/mL DCFDA dye for 20 min at room temperature. To this suspension 1 μL of 1 μg/mL DAPI stain was added and incubated further for 10 min. Islets were spun down at 400g for 3 min and washed twice with PBS. Finally, islets were re-suspended in 50 μL of PBS and mounted and a glass slide and observed under a phase contrast fluorescent microscope. The relative islet fluorescence intensity was measured by Image J software and then plotted as previously reported by Burgess et al. (Citation2010).
Assessment of islet viability using FDA (fluorescein diacetate)/PI staining
One hundred islets were stained with FDA/PI similarly as described in protocol for DCFDA staining. FDA and PI were used at a final concentration of 0.5 and 2 μg/mL respectively.
Comet assay
DNA single strand breaks were measured using the alkaline comet assay. Glass microscope slides were frosted with 1% normal melting point Agarose (type I-A) prepared in deionized water. Islet of Langerhans were re-suspended in 400 μL of 0.8% low melting point Agarose (type VII A) in PBS at 37 °C and pipetted onto a frosted microscope slide pre-coated with 100 μL of 1% normal melting point Agarose. Slides with layers of cells in agarose were incubated at 4 °C for 10 min and then immersed in lysis solution (2.5 mol/L NaCl, 100 mmol/L Na2EDTA, 10 mmol/L Tris, 1% Triton X-100, pH 10) for 1 h to remove cellular membranes. After lysis, slides were placed in a horizontal electrophoresis tank containing electrophoretic solution (1 mmol/L Na2EDTA, 300 mmol/L NaOH, pH 13) at 40 °C for 40 min. (DNA unwinding). Electrophoresis was performed in the same solution at 25 V, 300 mA, 40 °C for 30 min. The slides were washed three-times with neutralizing buffer (0.4 mmol/L Tris, pH 7.5) for 5 min at 40 °C before staining with 4, 6-diamidine-2-phenylinole dihydrochloride-DAPI (1 μg/mL).
Biochemical analysis
Reduced glutathione level was determined using 5,5′-dithio-bis (2-nitrobenzoic) acid (DTNB) which form a highly yellow colored compound developed spectrophotometrically at 412 nm (Beutler & Gelbart Citation1985). Lipid peroxidation was estimated as described by Ohkawa, where malondialdehyde (MDA), which reacts with thiobarbituric acid (TBA) and gives thiobarbituric reactive substance (TBARS) (Ohkawa et al. Citation1979). TBARS gives a characteristic pink color which can be measured colorimetrically at 532 nm. The superoxide dismutase activity was measured by inhibiting pyrogallol auto-oxidation under standard assay conditions, the reaction was measured at 420 nm spectrophotometrically (Marklund & Marklund Citation1974). Catalase decomposes H2O2, which was measured at 240 nm (Aebi Citation1984). Glutathione peroxidase (GPx) was measured as discussed by Hafeman et al. (Citation1974). Estimation of caspase-3 activity was using the instruction given in the kit (Sigma, Bangalore, India, # CASP-3-C).
Immunoblotting
Islets were collected by centrifugation and suspended in lysis buffer (1 mM EDTA, 50 mM Tris-HCl pH 7.5, 70 mM NaCl, 1% Triton, 50 mM NaF) containing 1× proteinase inhibitors (Sigma, Bangalore, India), incubated on ice for 30 min. After centrifugation at 16 000g for 15 min at 4 °C, the supernatant was collected and kept at −80 °C for future use. Total protein content was quantified using Bradford assay (Bio-Rad Bradford Solution, Haryana, India). The protein (20 μg) was loaded on a 10% polyacrylamide gel and then electrophoretically transferred onto a Hybond Nitrocellulose membrane (GE Healthcare, Bangalore, India). The membrane was then incubated for 1 h at room temperature in blocking buffer (TBS-T containing 5% skimmed milk) and further incubated overnight with the primary antibody at 4 °C (). Membrane was then washed four times 15 min each with TBST and incubated with HRP-conjugated secondary antibody for 1 h (). Finally, membrane was developed and visualized with Enhanced Chemiluminescence Western Blotting detection system (Millipore Inc., Bangalore, India).
Results
Islet isolation and viability
Rat islets were isolated using collagenase V digestion and handpicked for uniform size architecture. Islets were stained with dithizone stain to confirm the purity, and before each experiment, isolated islets were more than 99% viable, which was confirmed using Trypan blue staining, ensuring live healthy islets to study apoptosis and DNA damage ().
Figure 1. Trypan blue dye exclusion assay of isolated rat islets: (a) Isolated islets and DTZ staining. (b) Trypan blue dye exclusion assay to identify the pretreatment dose and time of incubation for EL MeOH ext. in a time dependent and dose dependent study assessing its maximum protective effect against 50 μM H2O2 induced cell death (n = 3).
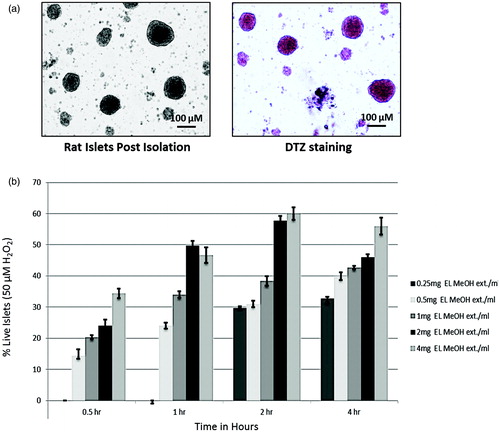
Standardization of effective dose and time of EL MeOH extract for cytoprotective activity
Dose and time-dependent experiment were performed in order to standardize effective dose and time of EL MeOH extract for protection against H2O2 induced loss of islets viability. In order to assess the cytoprotective effect of EL on Islet of Langerhans against the oxidative stress, Trypan blue dye exclusion test was performed. Islets were pre-incubated with EL MeOH ext. at 0.25, 0.5, 1, 2 and 4 mg/mL of assay volume for 0.5, 1.0, 2.0 and 4.0 h each. Results demonstrated partial protection against H2O2 induced toxicity in a dose and time dependent manner. We observed that pre-incubation before H2O2 treatment of 0.25 mg/mL EL MeOH ext. till 1 h had no protective effect. However, 2 mg/mL EL MeOH ext. for 2 h gave optimum cell viability of 58 ± 1.8% when compared to H2O2 treated islets which do not show any cell viability ().
FDA/PI staining for viability assessment
We performed islet viability both using FDA/PI staining. FDA stains live cells with green fluorescence, while PI stains dead cells giving red fluorescence. Freshly isolated rodent islets when stained for FDA/PI showed maximum viability by green fluorescent FDA staining while PI stained dead cells (). Further, when these islets were exposed to 50 μM H2O2, they showed enormous islet death as demonstrated by significant increase in the PI red fluorescence and decrease FDA green fluorescence intensities. While in other case, islets when pre-incubated with EL MeOH extract, we found enhanced protection to cytotoxicity in islets by 50 μM H2O2 and significantly fewer number of PI stained dead cells (). These results suggested that EL MeOH extract pretreatment provides enhanced protection against oxidative stress induced cell death.
Figure 2. FDA/PI and DCFDA Staining: (a) Qualitative assessment of islet viability when exposed to 50 μM H2O2, when pre-incubated with EL extract compared to the control group. (b) Comparative fluorescent intensity plot for FDA/PI staining (***p ≤ 0.001 versus Control FDA; @@p ≤ 0.01 versus Control PI; $p ≤ 0.05 versus H2O2 treated FDA; #p ≤ 0.05 versus H2O2-treated PI). DCFDA staining representing the amount of ROS generation. (c) Comparative qualitative assessment of the DCFDA staining between the control, 50 μM H2O2 and the EL extract-treated groups. Here, the DCFDA gives green fluorescence in the presence of ROS in direct proportion. Hence, this figure indicates the presence of ROS, which is higher in the H2O2-treated group compared to EL treated and (d) Quantitative graphical representation for the same (***p ≤ 0.001 versus Control group; $$$p ≤ 0.001 versus H2O2-treated group) (n = 3).
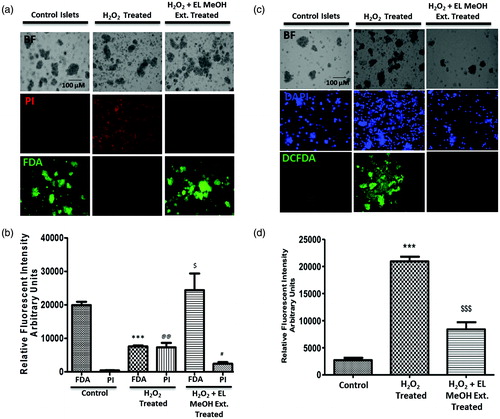
DCFDA staining for intra-cellular ROS generation
DCFDA is a measure for estimating intra-cellular ROS generation. Mild exposure of islets to H2O2 show increased level of intra-cellular oxidative stress (), when quantified was found to be approximately 4-fold higher compared to control islets (). Also, islets first pre-incubated with EL MeOH extract and later exposed to H2O2 showed highly reduced ROS generation within cells with low green fluorescent intensity, evidently suggesting normalized intracellular ROS stress. Thus, ROS estimation clearly indicates preventive potential of EL MeOH extract to islets rendering cytoprotection by lowering ROS generation similar to control islets.
Phosphotidylserine and propidium iodide staining in islets for live and dead cells
We further looked for apoptotic and necrotic cells both qualitatively and quantitatively by PS/PI dual staining. Freshly isolated islets after isolation did not show PS staining with fairly low apoptotic nuclei. On the other hand, Islet when exposed to H2O2 alone, showed significant increase in the apoptotic nuclei (red) on exposure with 50 μM H2O2 for 0.5 h with Annexin-V binding to phosphotidylserine (green). However, pre-incubating islets with 2 mg/mL of EL MeOH extract significantly inhibited H2O2-induced apoptosis (). It was observed that both Annexin-V-FITC and PI fluorescent intensities when quantified demonstrated significant reduction after EL MeOH ext. pretreatment ().
Figure 3. Cell death determined by Annexin V-FITC (PS)/PI staining and DNA damage by comet assay: (a) H2O2-treated group demonstrated significant increase in staining for PS and PI from the control group indicating cell death. However, there was no difference in staining of PS and PI in the EL pre-treated group and the control group but a significant reduction in stain uptake between the H2O2-treated and EL pre-treated group, which suggested that pre-incubation with EL protects the islets from undergoing oxidative stress induced cell death, which was quantified and depicted (b) (**p ≤ 0.01 versus Control Annexin V; @@p ≤ 0.01 versus Control PI; $$p ≤ 0.01 versus H2O2 treated Annexin V; ##p ≤ 0.01 versus H2O2-treated PI). (c) Comet Assay where the tailing is more pronounced in the case of H2O2 treated group suggesting DNA fragmentation, whereas the tailing is not seen in the case of EL-treated group giving a direct evidence of effective protection against DNA damage (n = 3).
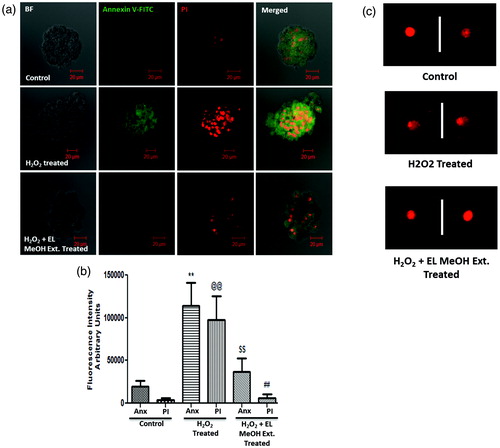
Comet assay for oxidative stress induced DNA damage
Damage to cellular DNA integrity, induced by H2O2 exposure was detected using an alkaline comet assay experiment. Acute exposure of islet cells to H2O2 facilitated increase in DNA damage which was observed by comet tail length. Control islets did not have stress and showed no tailing. Islets treated with 50 μM H2O2 demonstrate comet tailing suggested evident DNA damage in response to oxidative stress. However, islets pre-incubated with EL MeOH extract and then exposed to 50 μM H2O2 showed reduced comet tail length, indicating a protective effect over H2O2 induced DNA damage, hence cytoprotection ().
Immunoblotting and quantification of cell death markers due to oxidative stress
Oxidative stress results in DNA damage and activates DNA repair enzymes. This increase in DNA damage leads to PARP-1 activation causing depletion of cellular energy supply which drives the cell into apoptosis via activating caspases, an apoptotic executor. caspase-3 cleaves PARP-1 and abolishes its enzymatic activity that abates further energy depletion thus preventing cells from going into necrosis (Luo & Kraus Citation2012). Also, Oxidative stress increases the expression of inflammatory cytokines such as TNF-α and stress kinases like Phospho-p38 MAP kinases (Cnop et al. Citation2005). Herein, we attempted to analyze various cell death and stress markers by immunoblotting. Islets treated with and without hydrogen peroxide and pre-incubated with islets were assessed on gels and observed for markers like PARP-1, caspase3, TNF-α and p-38 MapK. In our experiments, we observed a relative increase in procaspase-3 and PARP-1 levels upon oxidative stress induction along with activated caspase-3 and cleaved PARP-1 levels in 50 μM H2O2 treated group. However, islets pre-incubated with EL MeOH extract demonstrated basal levels of PARP-1 and pro-caspase-3 (). Also, densitometric quantification showed significant reduction in both PARP-1 cleavage and caspase3 activation (). This pretreatment rescued the cells from DNA damage and death by oxidative stress. We further looked for MafA, a specific marker for the β cells, which did not change within the groups. This indicated uniform presence of β cells within the experimental islet groups (). We then looked for p-38 MapK phosphorylation which is a stress kinase and expressed in response to external or internal oxidative and other stress factors. Control islets did not show any rise in p-38 phosphorylation, whereas islets exposed to H2O2 demonstrated high p-38 phosphorylation due to ROS generation demonstrating ER stress in islet cells and thus cytotoxicity. Moreover, when islets were pre-incubated with EL MeOH extract, we found complete rescue of cells from this cytotoxicity by reverting p-38 phosphorylation back to basal levels as present in control islets (). TNF-α is a cytokine that plays a central role in islet inflammatory response pathway. Cytokines induce cell death similar to oxidative stress induced mechanism. Hence, it becomes evident to look for marker of cytokines mediated cell death induction. Therefore, in our case, we observed TNF-α activation in response to H2O2 induced cytotoxicity. As expected, control islets did show low levels of TNF-α precursor but no activation. Islets exposed to H2O2 showed a steep rise in TNF-α activation and precursor both, suggesting cytotoxicity via cytokine mediated cell death. However, islets pre-incubated with EL MeOH extract demonstrated rescue of cell from cytokine mediated cell death. EL treatment significantly drops TNF-α precursor level and its activation, demonstrating its cytoprotective effect on β-cells ().
Figure 4. Immunoblotting assay: (a) Western blot analysis for the following proteins to evaluate the protective effect of EL extract against 50 μM H2O2 induced apoptosis, Caspase-3, PARP-1, TNF-α, MafA, P-P38 MAPK and β-actin as endogenous control. Further, the protein expression profile is analyzed densitometrically. (b, c, e and f) Densitometry plots of activated caspase-3, cleaved PARP-1, phospho p-38 MAPK and TNF-α respectively where EL pretreatment significantly decrease there expression level after H2O2 treatment back to that of the control expression levels. (d) Densitometry plot for MafA which remain unchanged as discussed above (* indicates versus Control and # indicates versus H2O2-treated group; */#p ≤ 0.05; **/##p ≤ 0.01 and ***/###p ≤ 0.001) (n = 3).
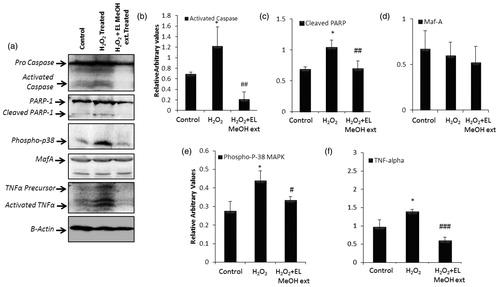
Antioxidant enzymes activity in H2O2 exposed and EL MeOH treated islets
Activated caspase-3 is a gold standard marker for cell death, hence caspase-3 enzyme activity was investigated in control, cumene H2O2 exposed and pre-incubated islets. We observed that pre-incubating islets with EL MeOH extract itself is sufficient to significantly reduce caspase-3 activity by 42% (). While looking on other antioxidant enzymes, 50 μM H2O2 exposed islets showed significantly increased lipid peroxidation levels (101%) but decreased GSH (75%), SOD (43%), catalase (53%) and GPx (44%) activities. However, pre-incubation with EL MeOH extract for 2 hours showed decreased lipid peroxidation (58%) whereas increased GSH content (68%), SOD (55%), catalase (51%) and GPx activities (44%) were observed respectively ().
Figure 5. Effect of EL on caspase-3 activity and the antioxidant enzyme activities viz. reduced glutathione, lipid peroxidation, glutathione peroxidase, catalase and superoxide dismutase in isolated islets on 50 μM H2O2 exposure and EL MeOH ext. pretreatment: (a) Caspase-3 activity which significantly increases on exposure to H2O2 and reduces significantly when pretreated with EL. (b) Similar results with LPO is shown. (c, d, e and f) Representation of antioxidant enzyme activities of GSH, Gpx, catalase and SOD respectively. Their activities significantly decrease in response to H2O2 induced oxidative stress but gets rescued with pretreatment with EL back to control levels (a indicates versus Control and b indicates versus H2O2 treated group; a/bp ≤ 0.05; aa/bbp ≤ 0.01 and aaa/bbbp ≤ 0.001) n = 3).
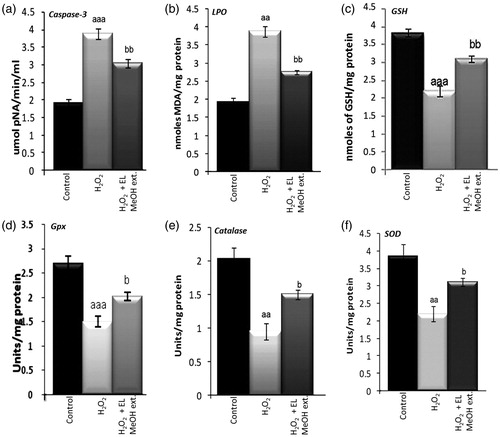
Discussion
Diabetes mellitus is characterized by hyperglycaemia due to excessive ROS generation within beta cells (Bonnefont-Rousselot Citation2002). Islets are known to have intrinsically very-low levels of antioxidant enzymes expression and activities (Lenzen et al. Citation1996; Tiedge et al. Citation1997). Hence, islets are at higher risk for ROS induced oxidative damage compared to other tissues. Many reports have shown that transient exposure of H2O2 is sufficient to cause significant damage to beta cells (Tang & Zhang Citation2000). In pancreatic islets, antioxidant enzymes CAT, SOD and GPx are expressed at fairly low levels (Robertson et al. Citation2003). The high expression levels of catalase and SOD in insulin secreting cells through genetic engineering provide protection against the toxicity of reactive oxygen species (Tiedge et al. Citation1998; Bottino et al. Citation2002). Herbs such as Curcuma longa have been shown to abate oxidative stress (Quiles et al. Citation2002) while its compound curcumin has been used to reduce ROS generation and increase insulin secretion from islets (Amoli et al. Citation2006; Kanitkar & Bhonde Citation2008); Puerarin, the main isoflavone glycoside found in the Chinese herb, Puerariae Lobatae Radix (Pueraria lobata (Willd) Ohwi), has been shown to have antioxidant activities such as increasing CAT and SOD activity in rat islets and to protect them from toxic effects of H2O2 (Xiong et al. Citation2006). Green tea (Camellia sinensis) extract when supplemented to streptozotocin induced diabetic rats also decreased serum glucose and increased serum and hepatic total antioxidant capacity (Haidari et al. Citation2013). Similarly, studies with other herbal extracts in streptozotocin-treated diabetic rat models have demonstrated their protective potential against oxidative stress induced damage by elevating antioxidant activity (Sarkhail et al. Citation2007; Stephen Irudayaraj et al. Citation2012; Nain et al. Citation2012). In another such study, it was demonstrated that S. lavandulifolia extract possessed marked anti-oxidative stress activity and it can be useful as a supplement in the management of diseases related to oxidative stress in humans (Rahzani et al. Citation2013). Also, hypoxia which occurs during the islet transplantation procedure initiates a cascade of biochemical reactions which results in the production of ROS causing oxidative stress leading to necrosis and apoptosis via intracellular pathways. Hence, incorporation of effective antioxidant supplementation in such procedures can enhance the chances of islet graft survival (Ramkumar et al. Citation2013).
Our present study coincides with many other earlier reports. Enicostemma littorale, a perennial herb, has been used for therapy of diabetes mellitus in India as a folk medicine (Saranya et al. Citation2013). Very recently we also demonstrated that EL MeOH extract possess potential islet neogenic activity from tissue specific stem/progenitor cells, which was later confirmed by identifying the active ingredient swertisin for this islet neogenic potential in a another recent report from us (Dadheech et al. Citation2010, Citation2013). There are also other reports where decrease in insulin resistance, normalization of fat and carbohydrate metabolism by EL were observed in both experimental cellular and rodent models (Maroo et al. Citation2002; Vasu et al. Citation2003; Patel et al Citation2013). Further, decreased oxidative stress by amelioration of antioxidant status in diabetic animals has also been observed (Maroo et al. Citation2003). EL has also been shown to possess hypolipidemic, hepato-protective, anti-edematogenic and free radical scavenging properties (Vasu et al. Citation2005; Vaijanathappa & Badami Citation2009). In the present study, we highlight novel cytoprotective and anti-apoptotic activity of EL just with pre-incubation of islets with 2 mg/mL EL MeOH extract for 2 h. This report imparts concrete evidence for significant improvement of GSH content, SOD, CAT and GPx activities in just 30 min on pre-incubation of islets before cytotoxicity. These anti-oxidant enzyme status and improved functionality suggests that the EL alone can strengthen the ability to combat oxidative stress and impart cytoprotection to cells against oxidative stress induced damage.
Further, caspase-3, the apoptotic executor can be activated by H2O2 (Turner et al. Citation1998). Also, PARP-1, DNA repair enzyme is activated in response to DNA damage caused by oxidative stress. Mild exposure to oxidative stress causes DNA damage and so, activation of PARP-1, leading to excessive energy consumption hence, apoptosis. Excessive depletion of energy further leads to caspase-3 activation and PARP cleavage, inhibiting PARP over activation. This allows the cells to enter apoptosis, thus preventing necrotic onset (Luo & Kraus Citation2012). In this study, we could show that H2O2-treated islets showed increased activity and expression of activated caspase-3 and PARP-1 cleavage indicating more apoptosis, which gets rescued very efficiently by just pre-incubating islets with EL MeOH extract. This was confirmed by significant reduction in the levels of activated caspase-3 and cleaved PARP-1. It has been reported that hydrogen-peroxide-associated ROS-mediated DNA damage takes place via direct attack on chromosome or mitochondrial pathways, leading to necrosis or apoptosis (Valko et al. Citation2007). Oxidative stress-induced cellular death can be prevented by blocking DNA damage. Our results from this study suggest that the EL MeOH extract protects H2O2 induced cells from apoptosis by efficiently blocking DNA damage.
Many reports have very well documented p38MapK, a stress kinase, affecting variety of intracellular responses, with well recognized roles in inflammation and cell death (Turner et al. Citation1998). Also, p-38 phosphorylation is found to be a standard measure to analyze ER stress in beta cells, leading to beta cell death. In this present study, phosphorylation of p38MapK was observed in the H2O2 treated islets, which reverts to normal basal levels with just pre-incubating islets with EL MeOH extract. Similar phenomenon occurs in the case of diabetes, where glucolipotoxicity causes ROS generation. Hence, increased p38MapK signaling has been described in both forms of diabetes, and is associated with late complications, such as ROS-mediated neuropathy and nephropathy (Coulthard et al. Citation2009). There is a direct correlation between inflammatory cytokines and pathogenesis of diabetes mellitus (Cnop et al. Citation2005). TNF-α is a known as potent modulator of glucose intolerance and insulin resistance in the peripheral tissue. Also, it is believed that macrophages and adipocytes are the primary sites of TNF-α production. Many groups have demonstrated that purified pancreatic islets serve as a potent source of TNF-α activation upon ROS exposure (Leeper-Woodford and Tobin Citation1997; Tobin et al. Citation2001). Hence, these reports explain the sharp increase in the TNF-α levels observed in the islets exposed to H2O2 in the case as well. Inflammatory cytokines and stress kinases have a profound inter-dependence that modulate and regulate beta cell function (Wellen & Hotamisligil Citation2005).
Henceforth, in present study, we demonstrated that p38MapK and TNF-α levels in the EL MeOH extract pre-incubated islets show normalized levels similar to control islets. This strongly suggests that EL has the capacity to counteract both inflammatory cytokines and oxidative stress mediated cytotoxicity. The significance of this study lies in the fact that this study is multifactorial right from isolation and purified islets, and usage of EL alone or in combination can provide efficient cytoprotection to islets while isolation procedure, storage and transplantation against oxidative stress mediated DNA damage and death ().
Conclusion
Our experimental observation and data suggest that EL protects islets or beta cells from oxidative stress (H2O2)-induced apoptosis by blocking ROS-mediated DNA damage, increasing antioxidants activity, suppressing expression of intra-islet stress kinases and proinflammatory cytokines. Therefore, this study strongly highlights a novel therapeutic agent that imparts protection to the Islets of Langerhans on various levels against oxidative stress.
Funding information
We would like to acknowledge Department of Science and Technology, Department of Biotechnology, and University Grant Commission, Ministry of Science and technology Govt. of India for providing fellowships to the researchers associated with this manuscript.
Acknowledgements
We acknowledge the Department of Biotechnology for establishing Instrumentation facility under DBT-MSUB-ILSPARE program, which allowed us to have access to Microscopy facility and other instruments.
Disclosure statement
We wish to confirm that there are no known conflicts of interest associated with this publication.
References
- Abirami P, Gomathinayagam M. 2013. Biochemical composition of Enicostemma littorale blume extracts. Bangladesh J Bot. 42:367–369.
- Aebi H. 1984. Catalase in vitro. Meth Enzymol. 105:121–126.
- Ambikapathy V, Mahalingam R, Panneerselvam A. 2011. GC-MS determination of bioactive compounds of Enicostemma littorale (Blume). Asian J Plant Sci Res. 1:56–60.
- Amoli MM, Mousavizadeh R, Sorouri R, Rahmani M, Larijani B. 2006. Curcumin inhibits in vitro MCP-1 release from mouse pancreatic islets. Transplant Proc. 38:3035–3038.
- Beutler E, Gelbart T. 1985. Plasma glutathione in health and in patients with malignant disease. J Lab Clin Med. 105:581–584.
- Bonnefont-Rousselot D. 2002. Glucose and reactive oxygen species. Curr Opin Clin Nutr Metab Care. 5:561–568.
- Bottino R, Balamurugan AN, Bertera S, Pietropaolo M, Trucco M, Piganelli JD. 2002. Preservation of human islet cell functional mass by anti-oxidative action of a novel SOD mimic compound. Diabetes. 51:2561–2567.
- Burgess A, Vigneron S, Brioudes E, Labbé JC, Lorca T, Castro A. 2010. Loss of human greatwall results in G2 arrest and multiple mitotic defects due to deregulation of the cyclin B-Cdc2/PP2A balance. Proc Natl Acad Sci USA. 107:12564–12569.
- Butler AE, Janson J, Bonner-Weir S, Ritzel R, Rizza RA, Butler PC. 2003. Beta-cell deficit and increased beta-cell apoptosis in humans with type 2 diabetes. Diabetes. 52:102–110.
- Catherwood MA, Powell LA, Anderson P, McMaster D, Sharpe PC, Trimble ER. 2002. Glucose-induced oxidative stress in mesangial cells. Kidney Int. 61:599–608.
- Clark A, Wells CA, Buley ID, Cruickshank JK, Vanhegan RI, Matthews DR, Cooper GJ, Holman RR, Turner RC. 1988. Islet amyloid, increased A-cells, reduced B-cells and exocrine fibrosis: quantitative changes in the pancreas in type 2 diabetes. Diabetes Res. 9:151–159.
- Cnop M, Welsh N, Jonas JC, Jörns A, Lenzen S, Eizirik DL. 2005. Mechanisms of pancreatic beta-cell death in type 1 and type 2 diabetes: many differences, few similarities. Diabetes. 54 Suppl 2:S97–107.
- Coulthard LR, White DE, Jones DL, McDermott MF, Burchill SA. 2009. p38(MAPK): stress responses from molecular mechanisms to therapeutics. Trends Mol Med. 15:369–379.
- Dadheech N, Singh A, Soni S, Gupta S, Bhonde RR. 2010. Enicostemma littorale: a new therapeutic target for islet neogenesis. Int J Integr Biol. 9:49–53.
- Dadheech N, Sanket S, Srivastava A, Dadheech S, Gupta S, Gopurappilly R, Bhonde RR, Gupta S. 2013. A small molecule swertisin from Enicostemma littorale differentiates NIH3T3 cells into islet-like clusters and restores normoglycemia upon transplantation in diabetic Balb/c mice. J Evid Based Complementary Altern Med. 2013: Article ID 280392, 20 pages.
- Grankvist K, Marklund SL, Taljedal IB. 1981. CuZn-superoxide dismutase, Mn-superoxide dismutase, catalase and glutathione peroxidase in pancreatic islets and other tissues in the mouse. Biochem J. 199:393–398.
- Guiot Y, Sempoux C, Moulin P, Rahier J. 2001. No decrease of the beta-cell mass in type 2 diabetic patients. Diabetes. 50 (Suppl 1):S188
- Hafeman DG, Sunde RA, Hoekstra WG. 1974. Effect of dietary selenium on erythrocyte and liver glutathione peroxidase in the rat. J Nutr. 104:580–587.
- Haidari F, Omidian K, Rafiei H, Zarei M, Mohamad Shahi M. 2013. Green tea (Camellia sinensis) supplementation to diabetic rats improves serum and hepatic oxidative stress markers. Iran J Pharm Res. 12:109–114.
- Kahn SE. 2003. The relative contributions of insulin resistance and beta-cell dysfunction to the pathophysiology of Type 2 diabetes. Diabetologia. 46:3–19.
- Kanitkar M, Bhonde RR. 2008. Curcumin treatment enhances islet recovery by induction of heat shock response proteins, Hsp70 and heme oxygenase-1, during cryopreservation. Life Sci. 82:182–189.
- Kloppel G, Lohr M, Habich K, Oberholzer M, Heitz PU. 1985. Islet pathology and the pathogenesis of type 1 and type 2 diabetes mellitus revisited. Surv Synth Pathol Res. 4:110–125.
- Kutlu B, Cardozo AK, Darville MI, Kruhøffer M, Magnusson N, Ørntoft T, Eizirik DL. 2003. Discovery of gene networks regulating cytokine-induced dysfunction and apoptosis in insulin-producing INS-1 cells. Diabetes. 52:2701–2719.
- Leeper-Woodford SK, Tobin BW. 1997. Tumor necrosis factor activity of pancreatic islets. Am J Physiol. 273:E433–437.
- Lenzen S, Drinkgern J, Tiedge M. 1996. Low antioxidant enzyme gene expression in pancreatic islets compared with various other mouse tissues. Free Radic Biol Med. 20:463–436.
- Luo X, Kraus WL. 2012. On PAR with PARP: cellular stress signaling through poly(ADP-ribose) and PARP-1. Genes Dev. 26:417–432.
- Marklund S, Marklund G. 1974. Involvement of the superoxide anion radical in the autoxidation of pyrogallol and a convenient assay for superoxide dismutase. Eur J Biochem. 47:469–474.
- Maroo J, Vasu VT, Aalinkeel R, Gupta S. 2002. Glucose lowering effect of aqueous extract of Enicostemma littorale Blume in diabetes: a possible mechanism of action. J Ethnopharmacol. 81:317–320.
- Maroo J, Ghosh A, Mathur R, Vasu VT, Gupta S. 2003. Antidiabetic efficacy of Enicostemma littorale methanolic extract in alloxan-induced diabetic rats. Pharm Biol. 41:388–391.
- Nain P, Saini V, Sharma S, Nain J. 2012. Antidiabetic and antioxidant potential of Emblica officinalis Gaertn. leaves extract in streptozotocin-induced type-2 diabetes mellitus (T2DM) rats. J Ethnopharmacol. 142:65–71.
- Ohkawa H, Ohishi N, Yagi K. 1979. Assay for lipid peroxides in animal tissues by thiobarbituric acid reaction. Anal Biochem. 95:351–358.
- Padgett LE, Broniowska KA, Hansen PA, Corbett JA, Tse HM. 2013. The role of reactive oxygen species and proinflammatory cytokines in type 1 diabetes pathogenesis. Ann N Y Acad Sci. 1281:16–35.
- Patel TP, Sanket S, Parikh P, Gosai J, Chruvattil R, Gupta S. 2013. Swertiamarin: an active lead from enicostemma littorale regulates hepatic and adipose tissue gene expression by targeting ppar-γ and improves insulin sensitivity in experimental NIDDM rat model. J Evid Based Complementary Altern Med. 2013:358673.
- Quiles JL, Mesa MD, Ramirez-Tortosa CL, Aguilera CM, Battino M, Gil A, Ramírez-Tortosa MC 2002. Curcuma longa extract supplementation reduces oxidative stress and attenuates aortic fatty streak development in rabbits. Arterioscler Thromb Vasc Biol. 22:1225–1231.
- Rahzani K, Malekirad AA, Zeraatpishe A, Hosseini N, Seify SM, Abdollahi M. 2013. Anti-oxidative stress activity of Stachys lavandulifolia aqueous extract in human. Cell J. 14:314–317.
- Ramkumar KM, Sekar TV, Bhakkiyalakshmi E, Foygel K, Rajaguru P, Berger F, Paulmurugan R. 2013. The impact of oxidative stress on islet transplantation and monitoring the graft survival by non-invasive imaging. Curr Med Chem. 20:1127–1146.
- Rieger AM, Nelson KL, Konowalchuk JD, Barreda DR. 2011. Modified annexin V/propidium iodide apoptosis assay for accurate assessment of cell death. J Vis Exp. 50:e2597.
- Robertson RP, Harmon J, Tran PO, Tanaka Y, Takahashi H. 2003. Glucose toxicity in beta-cells: type 2 diabetes, good radicals gone bad, and the glutathione connection. Diabetes. 52:581–587.
- Sakuraba H, Mizukami H, Yagihashi N, Wada R, Hanyu C, Yagihashi S. 2002. Reduced beta-cell mass and expression of oxidative stress-related DNA damage in the islet of Japanese Type II diabetic patients. Diabetologia. 45:85–96.
- Saranya R, Thirumalai T, Hemalatha M, Balaji R, David E. 2013. Pharmacognosy of Enicostemma littorale: a review. Asian Pac J Trop Biomed. 3:79–84.
- Sarkhail P, Rahmanipour S, Fadyevatan S, Mohammadirad A, Dehghan G, Amin G, Shafiee A, Abdollahi M.2007. Antidiabetic effect of Phlomis anisodonta: effects on hepatic cells lipid peroxidation and antioxidant enzymes in experimental diabetes. Pharmacol Res. 56:261–266.
- Shewade Y, Tirth S, Bhonde RR. 2001. Pancreatic islet-cell viability, functionality and oxidative status remain unaffected at pharmacological concentrations of commonly used antibiotics in vitro. J Biosci. 26:349–355.
- Srikanta S, Ganda OP, Jackson RA, Gleason RE, Kaldany A, Garovoy MR, Milford EL, Carpenter CB, Soeldner JS, Eisenbarth GS. 1983. Type I diabetes mellitus in monozygotic twins: chronic progressive beta cell dysfunction. Ann Intern Med. 99:320–326.
- Stephen Irudayaraj S, Sunil C, Duraipandiyan V, Ignacimuthu S. 2012. Antidiabetic and antioxidant activities of Toddalia asiatica (L.) Lam. leaves in streptozotocin induced diabetic rats. J Ethnopharmacol. 143:515–523.
- Tang J, Zhang JH. 2000. Mechanisms of [Ca2+]i elevation by H2O2 in islets of rats. Life Sci. 68:475–481.
- Tiedge M, Lortz S, Drinkgern J, Lenzen S. 1997. Relation between antioxidant enzyme gene expression and antioxidative defense status of insulin-producing cells. Diabetes. 46:1733–1742.
- Tiedge M, Lortz S, Munday R, Lenzen S. 1998. Complementary action of antioxidant enzymes in the protection of bioengineered insulin-producing RINm5F cells against the toxicity of reactive oxygen species. Diabetes. 47:1578–1585.
- Tobin BW, Leeper-Woodford SK, Hashemi BB, Smith SM, Sams CF. 2001. Altered TNF-alpha, glucose, insulin, and amino acids in islets of Langerhans cultured in a microgravity model system. Am J Physiol Endocrinol Metab. 280:E92–102.
- Turner NA, Xia F, Azhar G, Zhang X, Liu L, Wei JY. 1998. Oxidative stress induces DNA fragmentation and caspase activation via the c-Jun NH2-terminal kinase pathway in H9c2 cardiac muscle cells. J Mol Cell Cardiol. 30:1789–1801.
- Vaijanathappa J, Badami S. 2009. Antiedematogenic and free radical scavenging activity of swertiamarin isolated from Enicostemma axillare. Planta Med. 75:12–17.
- Valko M, Leibfritz D, Moncol J, Cronin MT, Mazur M, Telser J. 2007. Free radicals and antioxidants in normal physiological functions and human disease. Int J Biochem Cell Biol. 39:44–84.
- Vasu VT, Ashwinikumar C, Maroo J, Gupta S, Gupta S. 2003. Antidiabetic effect of Enicostemma littorale Blume aqueous extract in newly diagnosed non-insulin-dependent diabetes mellitus patients (NIDDM): a preliminary investigation. Orient Pharm Exp Med. 3:84–89.
- Vasu VT, Modi H, Thaikoottathil JV, Gupta S. 2005. Hypolipidaemic and antioxidant effect of Enicostemma littorale Blume aqueous extract in cholesterol fed rats. J Ethnopharmacol. 101:277–282.
- Wellen KE, Hotamisligil GS. 2005. Inflammation, stress, and diabetes. J Clin Invest. 115:1111–1119.
- Xia M, Laychock SG. 1993. Insulin secretion, myo-inositol transport, and Na(+)-K(+)-ATPase in glucose-desensitized rat islets. Diabetes. 42:1392–1400.
- Xiong FL, Sun XH, Gan L, Yang XL, Xu HB. 2006. Puerarin protects rat pancreatic islets from damage by hydrogen peroxide. Eur J Pharmacol. 529:1–7.