Abstract
Context Gelidiella acerosa (Forsskål) Feldmann & G. Hamel (Rhodophyta-Gelidiales) is a marine red macroalga. Our previous work found that a benzene extract of G. acerosa possesses noticeable neuroprotective activity, when evaluated through in vitro and in vivo systems.
Objective Bioactive-guided fractionation and identification of active compounds by column chromatography using solvents of varying polarity.
Materials and methods Fractionation was done by column chromatography, antioxidant and anticholinesterase activity was assessed by DPPH and cholinesterase inhibition assays (50–200 μg/ml), compound identification was done by LC-MS analysis, the mode of interaction of active compound was analyzed through docking studies and quantification was done by high-performance thin-layer chromatography (HPTLC) analysis.
Results The results suggest that fractions F9–F13 exhibited significant (p < 0.05) antioxidant and anticholinesterase activities. Hence, these fractions were pooled together and verified for neuroprotective activity. The pooled fraction was subjected to LC-MS analysis and among all the compounds, phytol was previously reported to possess excellent neuroprotective potential. Hence, the neuroprotective potential of phytol was assessed. The results suggest that phytol showed significant (p < 0.05) antioxidant activities (25–125 μg/ml) with an IC50 value of 95.27 ± 1.65 μg/ml and cholinesterase inhibitory potential (5–25 μg/ml) with IC50 values of 2.704 ± 0.07 and 5.798 ± 0.72 μg/ml for AChE and BuChE, respectively. Molecular docking studies suggest that phytol interacts with cholinesterase through the arginine residue of the enzyme. HPTLC quantification showed that about 6.266 μg of phytol was present per mg of pooled fraction.
Conclusion The study suggests that phytol might act as the key compound in contributing to the neuroprotective potential of G. acerosa.
Introduction
The marine red alga Gelidiella acerosa (Forsskål) Feldmann & G. Hamel (Rhodophyta-Gelidiales) has been employed as the principle agarophyte for the production of superior quality of agar. In recent years, evaluation of pharmacological properties of the secondary metabolites present in G. acerosa has been greatly increased (Elsie et al. Citation2011). The study led by Duraikannu et al. (Citation2014) reported that G. acerosa possess potential anticancer activity, when verified in Dalton’s ascitic lymphoma cells. Moreover, the antioxidant capability of G. acerosa has also been demonstrated through in vitro antioxidant systems (Megha & Anjali Citation2013). The seaweed was reported to have excellent antimicrobial activities against a wide range of bacterial and fungal species (Elsie & Dhanarajan Citation2010; Vivek et al. Citation2011). Apart from the above-mentioned therapeutic potential, our group has demonstrated that G. acerosa possess potential antioxidant (Suganthy et al. Citation2013), antiChE (Syad et al. Citation2012) and anti-amyloidogenic activities (Syad et al. Citation2013), when evaluated through various in vitro systems. In addition, G. acerosa benzene extract was also found to possess excellent neuroprotective potential against Aβ 25–35 induced toxicities, when evaluated under in vitro (PC 12 cell line) and in vivo systems (mice) (unpublished data). Since the identification of the active compound which is responsible for the above-mentioned neuroprotective activity is crucial for the development of novel drug entities against AD, in the present study, G. acerosa benzene extract was subjected to bioactive-guided fractionation and the active compounds present in the fractions were identified. The active fractions were further analyzed to identify the active compounds responsible for the neuroprotective activity.
Materials and methods
Chemicals
Phytol, ATCI and AChE were purchased from Sigma-Aldrich, Inc., (St Louis, MO), and BTCI was purchased from Hi Media Laboratories Pvt. Ltd., Mumbai, India. All the other chemicals and reagents used were of analytical grade with the highest purity.
Seaweed collection and solvent extraction
The red alga, G. acerosa was collected (in the month of July) from south Indian coastal area and the species was identified (by Dr. M. Ganesan, Scientist, CSMCRI, Mandapam Camp, Tamil Nadu) according to Oza and Zaidi (Citation2003) and Krishnamurthy and Joshi (Citation1970). The voucher specimen was deposited at Department of Biotechnology, Alagappa University, under the accession number AUDBTGA20100101. The collected seaweeds were washed thoroughly with distilled water, dried in shade, powdered and used for extraction. The powdered seaweed sample was defatted using petroleum ether and successively extracted with benzene. The extract was then subjected to complete evaporation and stored in an air-tight container.
Fractionation of G. acerosa benzene extract using column chromatography
Silica gel column (60–120 mesh) was used for separation. The column bed (evenly set) was prepared using hexane. G. acerosa benzene extract (7 g) was impregnated with silica gel and loaded onto the silica gel column (60–120 mesh). The solvents of varying ratio were introduced to the column and the fractions of double the bed volume were collected sequentially. The scheme of usage of solvent ratios is described in .
Evaluation of antioxidant potential by DPPH radical scavenging activity
DPPH assay is based on the reduction of DPPH, a stable free radical (which is purple in colour) (Shimada et al. Citation1992). To 333 μl of 0.1 mM of DPPH reagent (prepared in methanol), 1 ml of different doses of G. acerosa extracts (100–500 μg/ml) in water was added, mixed well and incubated at room temperature for 30 min. The absorbance was read at 517 nm in a UV-visible spectrophotometer (U-2800 model, Hitachi, Tokyo, Japan). BHT (100–500 μg/ml) was used as positive control.
Determination of cholinesterase inhibitory activity
AChE and BuChE inhibitory activity was evaluated by Ellman et al. (Citation1961) and Ingkaninan et al. (Citation2008). Different concentrations (100–500 μg/ml) of all the solvent extracts of G. acerosa were incubated with 10 μl of AChE or BuChE for 45 min at room temperature. To the reaction mixtures, 125 μl of 3 mM DTNB was added and the total volume was made up to 300 μl with Tris-HCl buffer (pH 8.0). Subsequently, 50 μl of 15 mM ATCI or BTCI was added to the reaction mixtures in order to initiate the enzyme activity. The formation of 5-thio-2-nitrobenzoate anion was detected by yellow colouration and the absorbance was detected at the wavelength of 405 nm using UV-visible spectrophotometer (U-2800). The experiments were done in triplicates. Donepezil (currently employed cholinesterase inhibitory drug) was used as the standard.
LC-MS analysis
The active fraction was subjected to LC-MS analysis using reverse phase C-18 column Shimadzu, Tokyo, Japan LC 10 ATVP with integrated library Metwin version 2.0. Electron spray ionization mode was employed (both positive and negative mode). Ten microlitres of the sample was injected for analysis and the column temperature was maintained at 25 °C. The m/z ratio range used was 50–1000 for the detection of compounds. The mobile phase used was acetonitrile and methanol (50:50) and the flow rate maintained was 1.5 ml/min. The chemical components of the fraction were identified by comparing with the library Metwin version 2.0.
Assessment of anti-Alzheimer potential of the ligand through molecular docking
The bioactive compound, which was identified in the active column fraction (through LC-MS analysis), was subjected to molecular docking. Since donepezil is the currently used drug candidate for AD, it was used as positive control. Three-dimensional (3D) structure of the active compounds was retrieved from PubChem database (http://pubchem.ncbi.nlm.nih.gov). The 3D structure of AChE enzyme (receptor protein) was obtained from Protein Data Bank (PDB code: 1B41). The binding pocket of the AChE protein was identified using ‘Define and edit binding site’ protocol availed from Discovery Studio (Shafreen & Pandian Citation2013). The presence of water molecules in AChE was removed and was further subjected to docking analysis for the evaluation of the AChE inhibitory potential of the active compound. The active compound was docked into the rigid binding pocket of AChE. The molecular docking studies were performed using Ligand Fit module, which is available in Discovery Studio package, version 2.5 (Accelrys, Inc., San Diego, CA).
High-performance thin-layer chromatography (HPTLC)
HPTLC analysis was performed to determine the amount of bioactive compound present in the pooled column fraction. The pooled column fraction was dissolved in methanol and the sample was loaded in 10 × 10 cm Silica gel 60F254 TLC plate. The plate was kept in TLC twin trough developing chamber (after saturation) with the mobile phase of benzene-ethyl acetate (1:1). The developed plate was dried and scanned in CAMAG (Wilmington, DE) TLC Scanner 3 at the wavelength of 254 nm. The data recorded were analyzed using winCATS Planar Chromatography Manager (Wilmington, DE).
Statistical analysis
Statistical analysis was performed using SPSS version 17.0 software package (SPSS Inc., Chicago, IL). The results of all the experiments were represented as mean ± S.D. Statistical analysis between the groups was performed by one-way ANOVA followed by post hoc multiple comparisons. p Values <0.05 were regarded as significant. IC50 values were calculated by probit analysis.
Results
Bioactive-guided fractionation of G. acerosa benzene extract
The bioactive-guided fractionation and separation of the extract was performed using a wide range of solvent ratios in the order of increasing polarity. The fractions eluted were evaluated for antioxidant and anticholinesterase activities using in vitro assays. The results of DPPH radical scavenging assay suggests that among all the fractions, F8–F14 exhibited significant (p < 0.05) antioxidant activity with the IC50 values of 36.13 ± 1.36 (F8), 38.08 ± 0.97 (F9), 40.55 ± 2.85 (F10), 40.09 ± 2.08 (F11), 41.66 ± 2.90 (F12), 41.99 ± 1.51 (F13), 39.20 ± 2.38 μg/ml (F14), respectively (. BHT, the standard antioxidant was used as positive control. The results of cholinesterase inhibitory assay suggest that among all the fractions evaluated, fractions F9–F13 exhibited higher AChE activities at the concentration of 200 μg/ml (). In the case of BuChE, fractions F9–F13 exhibited a significant (p < 0.05) inhibition of BuChE activity with the percentage of inhibition of 70.21 ± 5.86, 72.39 ± 10.05, 55.37 ± 0.45, 63.88 ± 3.81, 53.09 ± 2.31% for fractions F9, F10, F11, F12 and F13, respectively (). The IC50 values of the fractions were found to be 48.36 ± 1.38 (F9), 44.80 ± 2.02 (F10), 48.82 ± 6.91 (F11), 66.25 ± 12.89 (F12), 153.12 ± 16.79 μg/ml (F13), respectively for BuChE. Donepezil, the standard anticholinesterase drug was used as positive control.
Figure 2. Evaluation of DPPH radical scavenging activity of column fractions. The values are expressed as mean ± SD. *p < 0.05 compared with control.
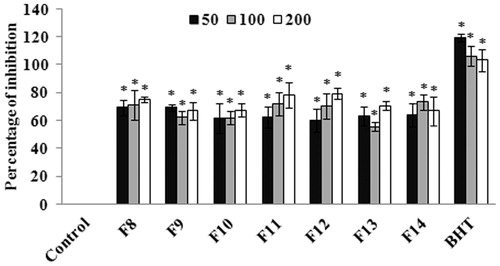
Figure 3. (a) Evaluation of AChE inhibitory activity of column fractions. The values are expressed as mean ± SD. *p < 0.05 compared with control. (b) Evaluation of BuChE inhibitory activity of column fractions. The values are expressed as mean ± SD. *p < 0.05 compared with control.
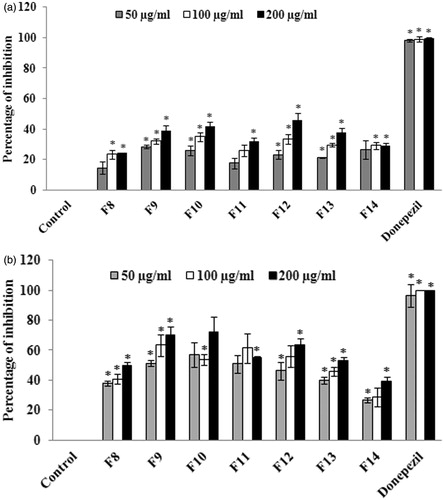
Since the fractions F9–F13 showed potent antioxidant and anticholinesterase activities, these fractions were pooled together and again verified for the antioxidant and anticholinesterase activities. The results of the assays demonstrated that the pooled fraction showed significant (p < 0.05) radical scavenging activity at the concentrations of 50, 100 and 200 μg/ml with the IC50 value of 79.29 ± 18.65 μg/ml (. The cholinesterase inhibitory assay suggests that at the concentration of 200 μg/ml, the pooled fraction also exhibited significant (p < 0.05) AChE (60.38 ± 2.55%) and BuChE (54.15 ± 5.84%) inhibitory activities ().
Figure 4. Determination of DPPH radical scavenging activity of the pooled column fractions (F9–F13). The values are expressed as mean ± S.D. *p < 0.05 compared with control group.
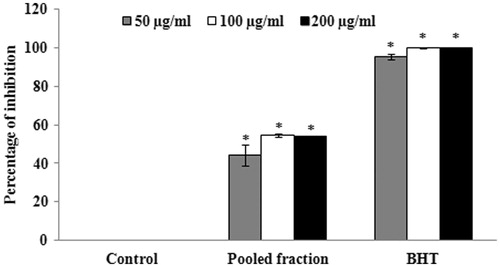
Figure 5. (a) Determination of AChE inhibitory activity of pooled column fractions. The values are expressed as mean ± SD. *p < 0.05 compared with control. (b) Determination of BuChE inhibitory activity of pooled column fractions. The values are expressed as mean ± SD. *p < 0.05 compared with control.
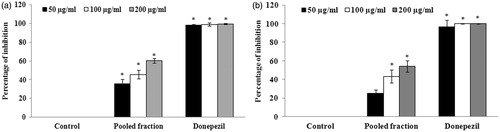
Identification of active compounds from the pooled column fraction through LC-MS analysis
A total of 13 compounds were obtained through LC-MS analysis of the pooled column fraction (). The major compounds identified in the pooled fractions include coumaryl alcohol, sinapyl alcohol, citramalic acid and phytol. Of the major compounds identified, phytol has been reported earlier to possess potential bioprotective potentials. Moreover, phytol is an acyclic monounsaturated diterpene alcohol terpenoid and a part of the chlorophyll molecule. It is also present in vitamin K, vitamin E and other tocopherols. Based on this background information on phytol, in the present study, the antioxidant and anticholinesterase activity was evaluated.
Table 1. Identification of active components presents in the pooled column fractions of G. acerosa benzene extract by LC-MS analysis.
Assessment of antioxidant and anticholinesterase potential of phytol
The antioxidant potential of phytol was evaluated through DPPH radical scavenging assay and the results suggest that phytol possesses significant (p < 0.05) scavenging activity at the concentration of 100 μg/ml with the percentage of inhibition of 52.63 ± 2.67% (. The IC50 value for phytol was found to be 95.27 ± 1.65 μg/ml. BHT, the standard antioxidant, was used as positive control. The cholinesterase inhibitory potential of phytol was also evaluated through AChE and BuChE inhibition assay. The results of the experiment demonstrated that phytol was found to possess significant AChE inhibitory activity (at the concentration of 5 μg/ml) with the percentage of inhibition of 92.41 ± 5.83% (). Similarly, phytol was also able to inhibit BuChE significantly (p < 0.05) at the concentration of 10 μg/ml with the percentage of inhibition of 74.21 ± 3.57% (). The IC50 values of phytol against AChE and BuChE was found to be 2.704 ± 0.07 and 5.798 ± 0.72 μg/ml, respectively.
Figure 6. Evaluation of the radical scavenging effect of various concentrations (25–125 μg/ml) of phytol. The values are expressed as mean ± SD. *p < 0.05 compared with control.
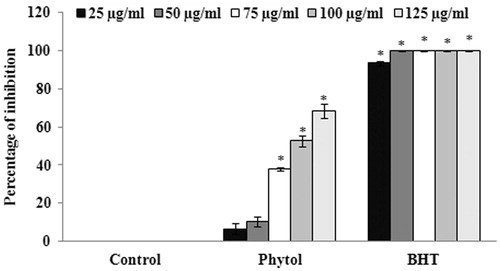
Figure 7. (a) Evaluation of AChE inhibitory activity of various concentrations (5–25 μg/ml) of phytol. The values are expressed as mean ± SD. *p < 0.05 compared with control. (b) Evaluation of BuChE inhibitory activity of various concentrations (5–25 μg/ml) of phytol. The values are expressed as mean ± SD. *p < 0.05 compared with control.
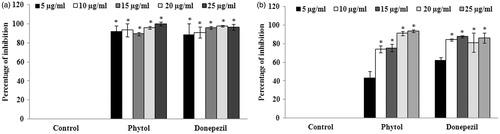
Investigation of binding mode of phytol in the binding site of AChE through docking studies
The most challenging task in evaluating the therapeutic potential of active compounds is to identify its affinity and selectivity towards their respective targets. Molecular docking has been regarded as the simple and rapid computational method, which is used for the assessment of the binding affinity of the ligands for the receptor (Wichapong et al. Citation2014). Hence, in the present study, the ability of phytol to interact with the binding pocket of the enzyme AChE in all the possible poses was studied. About 23 binding sites were predicted in AChE enzyme (the receptor molecule) through the binding pocket analysis using Ligand Fit module. Among these 23 binding sites, site 17 of AChE was found to have the maximum binding capability to bind with that of the control ligands (rivastigmine, donepezil, galantamine). illustrates the binding mode of the control ligands and the test ligand (phytol). ARG177 has been identified as the active site residue in AChE, which is essential for the establishment of strong interaction with the control ligands. Interestingly, the test ligand showed a similar kind of interaction with the arginine residue (ARG177) with a docking score of 27.372. The interaction of the control and test ligands with the AChE enzyme has been illustrated in .
Figure 8. Molecular interaction of (a) donepezil, (b) galantamine, (c) rivastigmine and (d) phytol with the enzyme acetylcholinesterase.
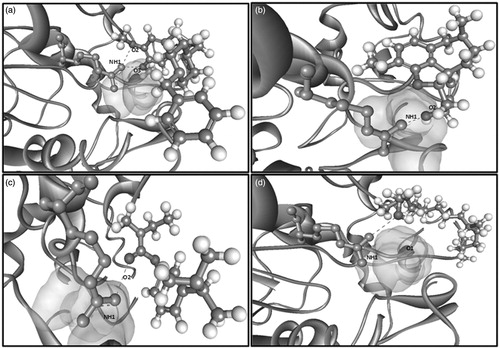
Table 2. Comparison of docking score and interactions of phytol with standard anti-AD drugs.
Quantitative HPTLC for the analysis of pooled active fractions
HPTLC is a simple and rapid technique for the qualitative and quantitative analysis of the natural compounds. This technique is a modern adaptation of TLC with advanced separation efficiency and detection limits (Shivatare et al. Citation2013). Since phytol possesses excellent antioxidant and anticholinesterase activities, the amount of phytol present in the pooled active column fraction was quantified by HPTLC analysis. illustrates the HPTLC chromatogram of pooled column fraction in comparison with phytol. The results suggest that the pooled fraction of G. acerosa contains 6.266 μg/mg of phytol (Rf value – 0.79).
Discussion
In the present study, the bioactive compound, which is responsible for the observed neuroprotective effect, was identified through the bioactive-guided fractionation. A wide range of solvents in different ratios in the order of increasing polarity were employed for fractionation. The fractions separated from G. acerosa benzene extract were assessed for antioxidant and anticholinesterase activities. The results demonstrated that the benzene:ethyl acetate (F8–F14) fractions (obtained from benzene extract of G. acerosa) exhibited excellent antioxidant activity among all the fractions evaluated. Apart from antioxidant activity, the benzene:ethyl acetate fractions (F9–F13) also showed inhibitory activity against both AChE and BuChE. Yoon et al. (Citation2008) demonstrated that the hexane:ethyl acetate fractions obtained from an ethanolic extract of the marine brown alga, Ecklonia stolonifera Okamura (Lessoniaceae) was found to exhibit inhibitory potential against AChE and BuChE. Since the benzene:ethyl acetate fractions (F9–F14) were found to possess both antioxidant and antiChE activities, these fractions were pooled together and again verified for the activities. The results indicated that the pooled fraction also holds potent antioxidant and antiChE activities and therefore, to identify the constituents present in the pooled active fractions, they were subjected to LC-MS analysis. About 13 compounds were obtained through the analysis among which phytol was reported to possess many bioprotective potentials. Earlier reports indicated that phytol has anticonvulsant effect in pilocarpine-induced seizures in mice (Costa et al. Citation2012). In addition to that, phytol derivatives have been found to act as immunostimulants by providing long-term memory induction and activating both innate and acquired immunity (Chowdhury & Ghosh Citation2012). The diterpene phytol has also been reported to possess antimicrobial potentials against Staphylococcus aureus and tuberculosis (Inoue et al. Citation2005; Saikia et al. Citation2010). Chang et al. (Citation2007) demonstrated that phytol exhibit neuroprotective effects on human neuroblastoma SH-SY5Y cells when evaluated in vitro by using a simulated ischemia model. In addition to the above-mentioned potentials, phytol holds an antioxidant and antinociceptive activity in in vitro and in vivo systems, respectively (Santos et al. Citation2013).
Since phytol holds promising therapeutic potential, in the present study, the compound phytol was verified for the antioxidant and anticholinesterase activities. The results suggest that phytol has the potent antioxidant capability and excellent cholinesterase inhibitory activity. In order to identify the mode of interaction of phytol with AChE, molecular docking was performed and the results suggest that phytol interacts with AChE through arginine residues thereby it exerts its inhibitory effect. The above results indicate that phytol might be the active compound responsible for the observed neuroprotective effects of G. acerosa and the HPTLC analysis further confirms the presence of phytol in the pooled column fraction.
Conclusion
The results of the present study demonstrated that the partially purified column fractions obtained from G. acerosa benzene extract possess potential antioxidant and antiChE activities and the presence of active compound phytol might be responsible for the observed effects of the seaweed extract. Since phytol holds promising therapeutic potentials, the compound could be developed as a potent therapeutic drug upon a deeper understanding of its mode of action.
Acknowledgements
KPD wishes to thank UGC, India, for offering Major Research Grant [F.No.36-6/2008 (SR)]. ANS wishes to thank UGC-MANF for the Senior Research Fellowship provided. The authors gratefully acknowledge the computational and bioinformatics facility provided by the Alagappa University Bioinformatics Infrastructure Facility (funded by the Department of Biotechnology, Government of India; Grant No. BT/BI/25/015/2012).
Disclosure statement
The authors report that there is no declaration of interest.
References
- Chang HJ, Kim HJ, Chun HS. 2007. Quantitative structure-activity relationship (QSAR) for neuroprotective activity of terpenoids. Life Sci. 80:835–841.
- Chowdhury RR, Ghosh SK. 2012. Phytol-derived novel isoprenoid immunostimulants. Front Immunol. 3:1–11.
- Costa JP, Ferreira PB, De Sousa DP, Jordan J, Freitas RM. 2012. Anticonvulsant effect of phytol in a pilocarpine model in mice. Neurosci Lett. 523:115–118.
- Duraikannu K, Rani KS, Anithajothi R, Umagowsalya G, Ramakritinan CM. 2014. In vivo anticancer activity of red algae (Gelidiela acerosa and Acanthophora spicifera). Int J Pharm Sci Res. 5:3347–3352.
- Ellman G, Courtney KD, Andres JV, Featherstone R. 1961. A new and rapid colorimetric determination of acetylcholinesterase activity. Biochem Pharmacol. 7:88–95.
- Elsie BH, Dhanarajan MS. 2010. Evaluation of antimicrobial activity and phytochemical screening of Gelidiella acerosa. J Pharm Sci Res. 2:704–707.
- Elsie BH, Dhanarajan MS, Sudha PN. 2011. In vitro screening of secondary metabolites and antimicrobial activities of ethanol and acetone extracts from red seaweed Gelidium acerosa. Int J Chem Res. 2:27–29.
- Ingkaninan K, Best D, Heijden VD, Hofte AJP, Karabatak B, Irth H, et al. 2008. High-performance liquid chromatography with on-line coupled UV, mass spectrometric and biochemical detection for identification of acetylcholinesterase inhibitors from natural products. J Chromatogr A. 872:61–73.
- Inoue Y, Hada T, Shiraishi A, Hirose K, Hamashima H, Kobayashi S. 2005. Biphasic effects of geranylgeraniol, teprenone, and phytol on the growth of Staphylococcus aureus. Antimicrob Agents Chemother. 49:1770–1774.
- Krishnamurthy V, Joshi HY. 1970. Checklist of Indian marine algae. Bhavnagar, India: CSMCRI.
- Megha MN, Anjali SB. 2013. Antioxidant potential of seaweeds from Kunakeshwar along the west coast Maharashtra. Asian J Biomed Pharm Sci. 3:45–50.
- Oza RM, Zaidi A. 2003. A revised checklist of Indian marine algae. Bhavnagar, India: CSMCRI.
- Saikia D, Parihar S, Chanda D, Ojha S, Kumar JK, Chanotiya CS, Shanker K, Negi AS. 2010. Antitubercular potential of some semisynthetic analogues of phytol. Bioorg Med Chem Lett. 20:508–512.
- Santos CC, Salvadori MS, Mota VG, Costa LM, Almeida AC, Oliveira GAL, Costa JP, Sousa DP, Freitas RM, Almeida RN. 2013. Antinociceptive and antioxidant activities of phytol in vivo and in vitro models. Neurosci J. 2013:949452.
- Shafreen RB, Pandian SK. 2013. Molecular modeling and simulation of FabG, an enzyme involved in the fatty acid pathway of Streptococcus pyogenes. J Mol Graph Model. 45:1–12.
- Shimada K, Fujikawa K, Yahara K. 1992. Antioxidative properties of xanthin and autooxidation of soybean oil in cyclodextrin emulsion. J Agric Food Chem. 40:945–948.
- Shivatare RS, Nagore DH, Nipanikar SU. 2013. ‘HPTLC’ an important tool in standardization of herbal medical product: a review. J Sci Innov Res. 2:1086–1096.
- Suganthy N, Nisha SA, Pandian SK, Pandima Devi K. 2013. Evaluation of Gelidiella acerosa, the red algae inhabiting South Indian coastal area for antioxidant and metal chelating potential. Biomed Prev Nutr. 3:399–406.
- Syad AN, Shunmugiah KP, Kasi PD. 2012. Assessment of anticholinesterase activity of Gelidiella acerosa: implications for its therapeutic potential against Alzheimer’s disease. Evid Based Comp Alt Med. 2012:1–8.
- Syad AN, Shunmugiah KP, Kasi PD. 2013. Seaweeds as nutritional supplements: analysis of nutritional profile, physicochemical properties and proximate composition of G. acerosa and S. wightii. Biomed Prev Nutr. 3:139–144.
- Vivek M, Kumar PS, Steffi S, Sudha S. 2011. Biogenic silver nanoparticles by Gelidiella acerosa extract and their antifungal effects. Avicenna J Med Biotechnol. 3:143–148.
- Wichapong K, Rohe A, Platzer C, Slynko I, Erdmann F, Schmidt M, Sippl W. 2014. Application of docking and QM/MM-GBSA rescoring to screen for novel Myt1 kinase inhibitors. J Chem Inf Model. 54:881–893.
- Yoon NY, Chung HY, Kim HR, Choi JS. 2008. Acetyl- and butyrylcholinesterase inhibitory activities of sterols and phlorotannins from Ecklonia stolonifera. Fisheries Sci. 74:200–207.