Abstract
Context Nigella sativa L. (Ranunculaceae) (NS) is traditionally used to treat many conditions such as inflammation.
Objective This study evaluates the effects of NS seeds ethanol extract in paracetamol-induced acute nephrotoxicity in rats.
Materials and methods Forty-eight female Wistar Albino rats were divided into eight groups: I = sham; II = sham + 1000 mg/kg NS; III = sham + 140 mg/kg (N-acetyl cysteine) NAC; IV = 2 g/kg paracetamol; V = 2 g/kg paracetamol + 140 mg/kg NAC; VI, VII and VIII = 2 g/kg paracetamol + 250, 500 and 1000 mg/kg NS, respectively. Paracetamol administration (oral) was carried out 1 h after NS and NAC administrations (oral), and all animals were sacrificed 24 h later.
Results Paracetamol administration significantly increased serum urea (88.05 U/L) and creatinine (0.80 U/L) when compared with the sham group (49.80 and 0.31 U/L, respectively). However, serum urea level was reduced to 65.60, 56.00 and 54.18 U/L, with 250, 500 and 1000 mg/kg doses of the extract, respectively. Also, serum creatinine level was reduced to 0.64, 0.57 and 0.52 U/L with 250, 500 and 1000 mg/kg doses of the extract, respectively. NS administration increased superoxide dismutase and glutathione, and decreased malondialdehyde levels in the kidneys. Kidney histopathological examinations showed that NS administration antagonized paracetamol-induced kidney pathological damage.
Discussion and conclusions The results suggest NS has a significant nephroprotective activity on paracetamol-induced nephrotoxicity. It may be suggested that the antiinflammatory and antioxidant effects of NS ethanolic extract originated from different compounds of its black seeds.
Introduction
Paracetamol (acetaminophen) is one of the most commonly used analgesic–antipyretic drugs worldwide, and in most countries, it is available without a prescription. Paracetamol overdose is known to cause hepatotoxicity and numerous studies about paracetamol-induced hepatotoxicity and its mechanisms are available in the literature. Significant paracetamol-induced hepatotoxicity usually triggers nephrotoxicity. Renal insufficiency is reported to occur in 1–2% of patients exposed to paracetamol toxicity (Prescott Citation1983). After oral administration, about 63% of paracetamol is metabolized via glucuronidation and 34% via sulphation primarily in the liver. The water-soluble metabolites consisting of these metabolic pathways are excreted via the kidney. N-Acetyl-p-benzoquinone (NAPQI) is a reactive intermediate that occurs when oxidization of <5% percent of paracetamol takes place by the microsomal P-450 enzyme system. NAPQI is detoxified by intracellular glutathione (GSH) in therapeutic doses (Dahlin et al. Citation1984). Accordingly, NAPQI has been implicated as responsible metabolite of paracetamol toxicity (Waring Citation2012). In paracetamol-overdosing cases, glutathione stores are depleted, and a rapid increase in the concentration of NAPQI causes necrosis (Bessems & Vermeulen Citation2001). Additionally this condition results in the production of massive metabolites, causing large amounts of unbound reactive species. N-Acetyl-cysteine (NAC) is an antidote currently being clinically used for paracetamol toxicity (Flanagan & Meredith Citation1991). NAC metabolizes to cysteine and increases the intracellular GSH level (Dilger & Baker Citation2007). Paracetamol toxicity creates acute tubular necrosis, which is one of the main causes of acute renal failure (Blantz Citation1996). Serum urea and creatinine levels may be indicators of acute tubular necrosis induced by paracetamol (Cobden et al. Citation1982; Blantz Citation1996). Free radicals are produced by exposure to drug toxicity in an organism, and oxidative damage plays an important role in paracetamol-induced hepatorenal injuries (Das et al. Citation2010; Kheradpezhouh et al. Citation2010). Therefore, natural compounds having antioxidant activity could be used for alternative treatments of paracetamol toxicity.
Nigella sativa L. (Ranunculaceae) (NS), commonly known as black seed, is a prominent herb among various medicinal plants, with a rich historical background and wide spectrum of pharmacological potential. NS seeds are widely used for many diseases throughout the world, including bronchitis, asthma, cough, diarrhoea, rheumatism, influenza and skin disorders. Additionally, it has been widely used as an antihypertensive, liver tonics, diuretics, digestive, antidiarrhoeal, appetite stimulant, antianxietic and antibacterial agents (Abdel-Sater Citation2009; Boskabady et al. Citation2010; Abel-Salam Citation2012; Ahmad et al. Citation2013; Bin Sayeed et al. Citation2014). Many active compounds have been isolated in different varieties of black seed thus far. Some of the most important active compounds are thymoquinone (30–48%), thymohydroquinone, dithymoquinone, p-cymene, carvacrol, α-pinene, thymol, quercetin, kaempferol and quercetin-3 (Al-Jassir Citation1992; Ahmad et al. Citation2013). Today, numerous clinical and animal investigations conducted on NS have explored important pharmacological actions, including antidiabetic, anticancer, immunomodulator, analgesic, antimicrobial, antiinflammatory, spasmolytic, bronchodilator, hepatoprotective, renoprotective, gastroprotective and antioxidant properties (Kokoska et al. Citation2008; Ravindran et al. Citation2010; Ashraf et al. Citation2011; Effenberger-Neidnicht & Schobert Citation2011; Ahmad et al. Citation2013). Studies have shown that NS ethanol extract and its major compounds quercetin, kaempferol and so forth have strong antioxidant properties and palliative effects on inflammation (Hadjzadeh et al. Citation2007; Dwarampudi et al. Citation2012). We have previously reported the antioxidant effect and anti-inflammatory activity of NS ethanol extract (Bayir et al. Citation2012a, Citation2012b). In the literature, there is no experimental study about protective effects of NS in paracetamol-induced nephrotoxicity. In this study, we investigated the potential reno-protective effects of NS ethanol extract in rats with paracetamol-induced nephrotoxicity.
Materials and methods
Animals
The study was approved by and conducted in accordance with the Institutional Animal Care and Use Committee at Ataturk University under protocol no. 31.05.2013/361. In total, 48 adult (6-month-old) female Wistar albino rats weighing 200 ± 20 g obtained from the Ataturk University Experimental Animal Laboratory (ATADEM) were used. All animals were housed in facilities approved by international guidelines.
Drugs
Paracetamol
A paracetamol suspension was prepared in 1% carboxy-methyl-cellulose in saline phosphate buffer, and animals were administered 2 g/kg of this suspension orally by gastric tube (1 mL of suspension contains 0.4 g of paracetamol) (Parasetamol, Doga Drug Stock LLC, Zekeriyaköy, Istanbul).
N-Acetylcysteine (NAC)
NAC (600 mg, Mentopini Hermesarzneimittel Gmbh, Münich, Germany) was provided and a solution was prepared in 0.9% saline, pH 7.0. Groups 3 and 5 were administered 140 mg/kg of this NAC solution orally by gastric tube.
Thiopental sodium
Thiopental sodium (I.E. Ulugay, Topkapı, Istanbul) was provided and used for intraperitoneal (i.p.) euthanasia at a 50 mg/kg dose.
Chemicals
All other chemicals were of the highest analytical grade commercially available.
Preparation and administration of the N. sativa extract
NS seeds were purchased from a local herbal shop in Erzurum, Turkey, at 2013 February. The seeds were botanically identified and authenticated by Dr. Esen Sezen Karaoglan. The herbarium specimen is deposited in the Department of Pharmaceutical Botany, Faculty of Pharmacy, Ataturk University at 4 ± 2 °C. The seeds (100 g) were cleaned, dried, mechanically powdered and extracted with 300 mL of 96% ethanol three times. The obtained extracts were filtered and combined, and the solvent was evaporated under reduced pressure using a rotary, which yielded a blackish-brown liquid concentrate (yield 25%) that was kept in a domestic refrigerator at 4 °C. The doses of NS (250, 500 and 1000 mg/kg) were selected from described values in the literature (Hadjzadeh et al. Citation2007; Al-Naqeep et al. Citation2011; Hadjzadeh et al. Citation2011). The extract was emulsified in water and administered to rats using an oral gavage.
Experimental design
The rats were randomly divided into the following eight groups, with each group containing six rats: (I) sham operation group, (II) sham operation + 1000 mg/kg NS group, (III) sham operation + 140 mg/kg NAC group, (IV) 2 g/kg paracetamol group, (V) 2 g/kg paracetamol + 140 mg/kg NAC group, (VI) 2 g/kg paracetamol + 250 mg/kg NS group; (VII) 2 g/kg paracetamol + 500 mg/kg NS group and (VIII) 2 g/kg paracetamol + 1000 mg/kg NS group (). The groups were housed separately in different cages.
Table 1. Experimental protocols in the eight nephrotoxicity groups.
Experimental modelling
During the acclimatization period, the rats were fed a diet of standard commercial rat pellets and fresh water ad libitum and maintained under constant conditions (temperature: 22 ± 1 °C, humidity: 40–60% and controlled lighting using a 14 h:10 h light-dark cycle for a week prior to the experiments). Sixteen hours before the experiment, the animals were allowed water only. In view of diurnal enzyme activity variations, animals were sacrificed at a fixed time. All surgical procedures were performed under sterile conditions. The paracetamol dose to induce nephrotoxicity was selected from the values described in the literature (Kuralay et al. Citation1998; Chattopadhyay Citation2003). The rats were deprived of food after paracetamol administration but had free access to food and water 4 h after paracetamol administration until they were sacrificed. In addition, 24 h after paracetamol administration, the experiment was terminated by thiopental sodium at a 50 mg/kg dose and all animals were then sacrificed. The kidneys were rapidly removed and washed in ice-cold saline in all groups. The organs were labelled and stored at −80 °C until the biochemical analyses were conducted. Cardiac blood samples were collected immediately and transferred to the laboratory from all groups, and the sera were obtained to determine biochemical parameters.
Biochemical investigations
Biochemical investigation of kidney tissues
After macroscopic analyses, rat tissues were kept at −80 °C. All tissue samples from each rat were first perfused with PBS/heparin and then grinded in liquid nitrogen using a tissue lyser II grinding Jar Set. As well, 0.1 g was weighed and then treated with 4.5 mL of an appropriate buffer. This mixture was homogenized on ice using an Ultra-Turrax homogenizer for 5 min. Homogenates were filtered and centrifuged using a refrigerator centrifuge at 4 °C. These supernatants were then used to determine SOD and MDA levels with highly sensitive ELISA kits (Cayman Chemical SOD assay kit item number 706002, Cell Biolabs Oxi Select™ TBARS Assay STA-330 Kit, Cell Biolabs, San Diego, CA, respectively). Measurements were performed according to the manufacturers’ instructions. The GSH levels in the kidney tissues were measured using the method created by Sedlak and Lindsay (Citation1968), according to the modified methods, with an ELISA reader. The average absorbances of each sample and standard were calculated. A standard curve was plotted and an equation was obtained from the absorbances of standards. All assays were carried out at room temperature in duplicate. Linear SOD, GSH and MDA concentrations were calculated according to this equation. The results of the SOD, GSH and MDA levels in the tissues were expressed as, respectively, U/mg protein, nmol/mg protein and nmol/mg protein. All data were presented as the mean ± standard deviation (SD) results based on per mg protein.
Biochemical investigation of serum
Serum urea and creatinine levels were measured using the commercial kits (BEN) (Biochemical Enterprise, Milano, Italy) BK 151 and CR280 in an automatic biochemical analyzer (ChemWell, Palm City, FL) at 340 nm and 510 nm, respectively.
Histological examination
The kidneys of rats in all groups were fixed in 10% neutral formalin for 48–72 h. Then, tissues were trimmed and processed for a routine histopathological examination. Tissues were embedded into paraffin wax and 4–5 μm thick sections were cut. All tissue sections were stained with Haematoxylin and Eosin (H&E) and then examined under a light microscope (Olympus BX51, Tokyo, Japan).
Renal lesions in rats were assessed according to Zhang et al. (Citation2008) and graded into five categories by utilizing a scale of 0–5, where 0 = normal histology and 1 = tubular epithelial cell degeneration, without significant necrosis/apoptosis; 2–5 = <25%, <50%, <75% and ≥75% of the tubules showed tubular epithelial cell necrosis/apoptosis, respectively, accompanied by other concomitant alterations.
Statistical analysis
Data obtained from the serum urea and creatinine levels, tissue SOD activity and GSH and MDA levels were subjected to a one-way analysis of variance (ANOVA) followed by Duncan’s multiple range test (DMRT). Differences among the groups were obtained using the Duncan multiple comparison and were considered significant at p < 0.05. All results were expressed as mean ± SD using the IBM SPSS Statistics 19 software (SPSS Inc., Chicago, IL). Results of the histological score were compared between groups using a one-way ANOVA and Tukey’s multiple comparison tests. Histological statistical analyses and graphs were prepared using GraphPad Prism version 4.0 (GraphPad Software, La Jolla, CA), and p < 0.05 was considered statistically significant.
Results
The effects of NS on serum urea and creatinine levels
Serum urea and creatinine levels were found to be higher in the paracetamol group when compared with the sham group (p < 0.05). NS administration dramatically reduced serum urea and creatinine levels. The NS extract showed a dose-dependent protective effect on paracetamol-induced nephrotoxicity compared with the standard drug, NAC. The serum levels of urea and creatinine were found to be lower in the NS treatment groups (NS 250, NS 500 and NS 1000 mg/kg, groups) (p < 0.05). The extract at a dose of 1000 mg/kg had a significantly higher protective effect on nephrotoxicity than standards at 250 and 500 mg/kg; in fact, the protective effect at this dose was significantly higher than the effect of NAC (p < 0.05).
The administration of paracetamol caused increments in both serum urea and creatinine levels. The serum urea level, which increased with paracetamol treatment to 88.05 U/L, was reduced to 65.60, 56.00 and 54.18 U/L, with 250, 500 and 1000 mg/kg doses of the extract, respectively. The serum creatinine level, which increased to 0.80 U/L by paracetamol administration, was reduced to 0.64, 0.57 and 0.52 U/L with 250, 500 and 1000 mg/kg doses of the extract, respectively ().
Table 2. Effects of NS treatments on changes in paracetamol-induced nephrotoxicity in rats.
Biochemical results for oxidant and antioxidant levels of kidney tissue in rats
The activity of SOD and the levels of MDA and GSH in the paracetamol toxicity in rat kidneys are shown in . SOD activity and the GSH level were found to be decreased and the MDA level was found to be significantly increased in the paracetamol group compared with the sham group (p < 0.05).
Table 3. Effects of NS treatments on tissue SOD activity and GSH and MDA levels in paracetamol-induced nephrotoxicity in rats.
NS administration restored GSH and SOD and decreased MDA levels in kidneys (). The NS extract showed a dose-dependent inhibition compared with the standard drug, NAC. The extract at a dose of 1000 mg/kg had significantly higher scavenging activity on kidneys than standards at 250 and 500 mg/kg; in addition, the scavenging activity at this dose was significantly higher than the effect of NAC (p < 0.05).
Results of histopathological examinations
Histopathological scores of rat kidneys in all groups are presented in . Kidneys of the control group () animals showed a normal histologic morphology. The sham + NS 1000 mg/kg group () and the sham + NAC 140 mg/kg group () animal kidneys were observed to be similar to those of the control group. The paracetamol 2 g/kg + NS 1000 mg/kg group () and the paracetamol 2 g/kg + NAC 140 mg/kg group () had similar morphologies to that of the normal group, and interstitial extravasation of red blood cells (RBCs) and vascular congestion could not be observed. Restoration of the apical brush border and the narrow lumina was detected in most of the tubules.
Figure 1. Histopathological score differences in groups. *Indicates that histopathological score significantly greater than the sham group, #indicates that histopathological score is significantly lower than the paracetamol 2 g/kg group, p < 0.05 was considered statistically significant.
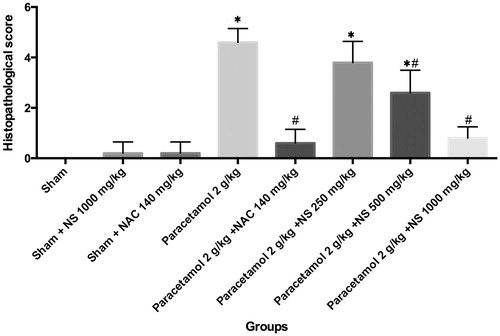
Figure 2. (A) Normal structure of kidney in control group. Haematoxylin and eosin. Magnification 200×. (B) Stained sections of kidney of sham + NS 1000 mg/kg group. Slight degenerations were seen. Haematoxylin and eosin. Magnification 200×. (C) Stained sections of kidney of sham + NAC 140 mg/kg group. Similar appearance to the control group. Haematoxylin and eosin. Magnification 200×. (D) Stained sections of kidney of paracetamol 2 g/kg + NS 250 mg/kg group. Haematoxylin and eosin. Magnification 200×. (E) Paracetamol-administered rat kidney. Severe necrosis, congestion and extravasation of red blood cells were seen. Haematoxylin and eosin. Magnification 200×. (F) Stained sections of kidney of paracetamol 2 g/kg + NS 500 mg/kg. Slightly to mild degenerations were seen. Haematoxylin and eosin. Magnification 200×. (G) Paracetamol 2 g/kg + NAC 140 mg/kg group. Structures of kidney were similar to the control group. Haematoxylin and eosin. Magnification 200×. (H) Paracetamol 2 g/kg + NS 1000 mg/kg group. Structures of kidney were similar to the control group. Haematoxylin and eosin. Magnification 100×.
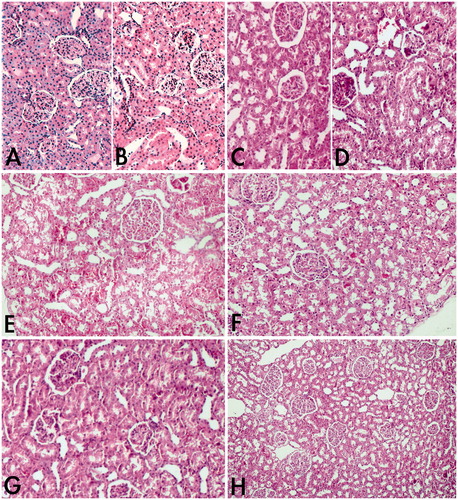
The histopathology of the kidneys of the paracetamol-treated group () showed severe proximal tubular distortion with enlarged lumina. Signs of necrosis, vacuolization, epithelial degeneration and pyknotic nuclei, in addition to the partial to complete loss of the luminal brush border, were observed. Hyaline casts, desquamated cells and necrotic cell debris were seen in the lumina of some tubules. Extravasation of RBCs and congested blood vessels were also encountered in the interstitium of the renal cortex. Stained sections of the paracetamol 2 g/kg + NS 250 mg/kg group () and the paracetamol 2 g/kg + NS 500 mg/kg group () maintained a better morphology with a marked reduction in the extent of proximal tubular necrosis. The presence of mild tubular epithelial vacuolization, luminal cast formation and cell desquamations were hardly detected.
Discussion
Although paracetamol, with the alternative name acetaminophen, has a reasonable safety profile in therapeutic doses, its overdose remains the most important cause of liver injury and even death in many parts of the world among all drug toxicities (Larson et al. Citation2005; Karakus et al. Citation2013; Yayla et al. Citation2014). Hepatotoxic and nephrotoxic effects of paracetamol overdose occur by a complex sequence of events (Hinson et al. Citation2010). Acetaminophen-induced nephrotoxicity becomes evident after hepatotoxicity in most cases, but the occurrence of renal tubular damage and acute renal failure, even in the absence of liver injury, should not be ignored (Eguia & Materson Citation1997).
Although the hepatotoxic mechanism of paracetamol overdose is well described, there is little evidence of the nephrotoxic mechanism of paracetamol. Based on the clinical and animal studies, some of the potential mechanisms of nephrotoxicity are the cytochrome P-450 pathway, prostaglandin synthesis and N-deacetylase enzymes (Bessems & Vermeulen Citation2001). At high doses of paracetamol, the reactive derivative of paracetamol occurred by the P-450-dependent metabolism, NAPQI could not be detoxified by GSH and depleted GSH levels will cause liver necrosis, leakage of hepatocellular contents into the blood and liver failure (Eguia & Materson Citation1997; Mugford & Tarloff Citation1997; Karakus et al. Citation2013). Loss of glutathione with an increased formation of reactive oxygen species and increased oxidative stress are essential events of the toxicity mechanism. In association with these essential events, many inflammatory mediators, such as certain cytokines and chemokines, are released by various mechanisms, and these mediators can modify the toxicity (Hinson et al. Citation2010). Free radicals can stimulate lipid peroxidation and play a key role in drug toxicities (Kehrer Citation1993). As a result of this lipid peroxidation, MDA levels increase and this reflects tissue damage (Draper & Hadley Citation1990). SOD and GSH are key antioxidant defence system enzymes to detoxify reactive molecules or repair the resulting damage in the cell (Whidden et al. Citation2011).
Acetyl cysteine has long been recognized as an effective antidote of paracetamol overdose and it is routinely used to minimize and repair hepatotoxicity in overdose cases. By having rich cysteine sources, acetyl cysteine provides cysteine to stimulate the replenishment of GSH, and increased GSH plays a key role in the detoxification of NAPQI (Massey & Racz Citation1981; Dawson et al. Citation1984). The antioxidant effects of NS and its biologically active components quercetin and kaempferol have been demonstrated in a large number of studies on different organs (Kokoska et al. Citation2008; Ashraf et al. Citation2011; Bourgou et al. Citation2012).
In this study, we demonstrated that NS ethanol extract plays an important role in paracetamol-induced nephrotoxicity. In the assessment of kidney injury, levels of urea and creatinine in serum should be first determined. These enzymes are very sensitive markers employed in the diagnosis of kidney diseases. Serum urea and creatinine levels may be indicators of acute tubular necrosis caused by paracetamol toxicity (Cobden et al. Citation1982; Blantz Citation1996). In our study, paracetamol administration altered serum urea and creatinine levels, indicating nephrotoxicity. These results were in accordance with previous studies (Anthony et al. Citation2012; Saxena et al. Citation2012). The administration of NS as a nephroprotective alternative decreased these altered values up to the serum levels of control groups. In fact, the highest serum urea and creatinine reductions were observed using NS at a dose of 1000 mg/kg, and this reduction was statistically significantly higher than that of NAC administration. These results have shown that NS improves the nephrotoxic effects of paracetamol.
It is well known that SOD, GSH and MDA are important indicators of the antioxidant capacity of the body that resulted in protection against the damage caused by oxidative stress. In the present study, we have investigated alterations to the tissue MDA level and reductions in the tissue GSH level and SOD activity as markers of the oxidative stress process following the oral administration of paracetamol. The increase in lipid peroxidation was accompanied by a significant reduction in the GSH level. GSH is a tripeptide, that is, found in many mammalian tissues and is an important free-radical scavenger and scavenger of NAPQI, which is a reactive intermediate of paracetamol (Yayla et al. Citation2014). It plays an important role in the antioxidant defence system and removes free-radical species, such as hydrogen peroxide and superoxide radicals, and it maintains membrane protein thiols. The decreased SOD and GSH levels and the increased MDA levels were in accordance with previous research studying paracetamol-induced nephrotoxicity (Ghosh & Sil Citation2007; Yousef et al. Citation2010; Abdul Hamid et al. Citation2012). Through NS administration, tissue MDA levels decreased and GSH levels and SOD activity increased, showing the antioxidant activity of the extract. These results suggest that NS reduces the oxidative stress in kidneys. We can say that NS prevented the depletion of antioxidant enzymes, including GSH, in the present study. This suggestion is supported by histopathologic findings, indicating that the administration of the NS extract significantly prevented necrosis and congestion, as well as reduced the degree of vascular dilatation. Previous studies suggesting potential antioxidant capacity of NS extracts support our findings (Havakhah et al. Citation2014; Mollazadeh & Hosseinzadeh Citation2014). Also, thymoquinone, which is the main ingredient of NS, supplementation reverses lead-induced oxidative stress in adult rat testes (Mabrouk & Ben Cheikh Citation2015). Previously Nagi et al. (Citation2010) suggested that thymoquinone reverses acetaminophen-induced oxidative stress, nitric oxide production and energy decline in mice liver. In previous studies, thymol, thymoquinone and other active ingredients of NS acted as scavenger of superoxide, hydroxyl radical and singlet molecular oxygen (Kruk et al. Citation2000; Nagi & Mansour Citation2000; Badary et al. Citation2003). Thymoquinone supplementation increases the expression of antioxidant genes, superoxide dismutase, catalase and glutathione peroxidase (Ismail et al. Citation2010). Thus, NS extract may reduce oxidative stress through direct antioxidant effect of its ingredients as well as induction of endogenous antioxidant enzymes leading to the prevention of paracetamol-induced nephrotoxicity.
Our results show that the selected dose of paracetamol-induced nephrotoxicity in biochemical and histopathologic aspects. The administration of NS significantly reduced the toxic effects of paracetamol on the kidneys in a dose-dependent manner. The nephroprotective properties of NS may be related to its positive effects on the antioxidant system. It is concluded that NS can protect kidneys from the damage caused by paracetamol overdose and might be a potential therapeutic candidate against paracetamol-induced acute nephrotoxicity.
Conclusion
In light of these observations, we found that the therapeutic administration of NS prevented paracetamol-induced oxidative stress changes. It can be speculated that the role of the ethanoic NS extract in preventing the formation of paracetamol-induced nephrotoxicity, as seen in the present study, is in part due to the anti-inflammatory and antioxidant effects of the different compounds (thymoquinone, qurecetin, kaempferol and so forth) of the black seeds. These compounds may interfere with antioxidant and anti-inflammatory mechanisms, which may be crucial to reducing paracetamol-induced nephrotoxicity. Further prospective and randomized clinical controlled trials are required to understand better this novel candidate for use in treating paracetamol nephrotoxicity and reducing paracetamol-related mortality.
The use of NS seeds for centuries as a condiment in Turkish dishes, without side effects, and the desirable amount of effective substances present in the seeds encourage consideration of NS as a new and natural medicinal source.
Funding information
This study was supported by the Research fund of Atatürk University (BAP-2012/077).
Acknowledgements
This research is included in Master's thesis of Dogukan Canayakin and was conducted in the Laboratory of Biochemistry, Laboratory of Pharmacology at Ataturk University, Faculty of Pharmacy and Medicine, 25240 Erzurum/Turkey. The second author (Y. Bayir) wishes to thank Professor Ahmet Cakir for his kind support in the study.
Disclosure statement
None of the authors has a commercial or financial interest or other relationship with the manufacturers of pharmaceuticals, laboratory supplies and/or medical devices or with commercial providers of medically related services.
References
- Abdel-Sater KA. 2009. Gastroprotective effects of Nigella sativa oil on the formation of stress gastritis in hypothyroidal rats. Int J Physiol Pathophysiol Pharmacol. 1:143–149.
- Abdul Hamid Z, Budin SB, Wen Jie N, Hamid A, Husain K, Mohamed J. 2012. Nephroprotective effects of Zingiber zerumbet Smith ethyl acetate extract against paracetamol-induced nephrotoxicity and oxidative stress in rats. J Zhejiang Univ Sci B. 13:176–185.
- Abel-Salam BK. 2012. Immunomodulatory effects of black seeds and garlic on alloxan-induced diabetes in albino rat. Allergol Immunopathol (Madr). 40:336–340.
- Ahmad A, Husain A, Mujeeb M, Khan SA, Najmi AK, Siddique NA, Damanhouri ZA, Anwar F, Kishore K. 2013. A review on therapeutic potential of Nigella sativa: a miracle herb. Asian Pac J Trop Biomed. 3:337–352.
- Al-Jassir MS. 1992. Chemical composition and microflora of black cumin (Nigella sativa L.) seeds growing in saudi arabia. Food Chem Toxicol. 45:239–242.
- Al-Naqeep G, Al-Zubairi AS, Ismail M, Amom ZH, Esa NM. 2011. Antiatherogenic potential of Nigella sativa seeds and oil in diet-induced hypercholesterolemia in rabbits. Evid Based Complement Alternat Med. 2011:213628.
- Anthony OE, Mbuh AF, Emmanuel MP. 2012. Phytochemical screening, and assessment of ameliorating effect of aqueous and ethanolic extracts of Gmelina arborea on drug induced hepatic and renal insufficiency in rats. Pak J Pharm Sci. 25:457–461.
- Ashraf SS, Rao MV, Kaneez FS, Qadri S, Al-Marzouqi AH, Chandranath IS, Adem A. 2011. Nigella sativa extract as a potent antioxidant for petrochemical-induced oxidative stress. J Chromatogr Sci. 49:321–326.
- Badary OA, Taha RA, Gamal el-Din AM, Abdel-Wahab MH. 2003. Thymoquinone is a potent superoxide anion scavenger. Drug Chem Toxicol. 26:87–98.
- Bayir Y, Albayrak A, Can I, Karagoz Y, Cakir A, Suleyman H, Uyanik H, Yayla N, Polat B, Karakus E, et al. 2012a. Nigella sativa as a potential therapy for the treatment of lung injury caused by cecal ligation and puncture-induced sepsis model in rats. Cell Mol Biol (Noisy-le-Grand). 58:OL1680–OL1687.
- Bayir Y, Karagoz Y, Karakus E, Albayrak A, Sengul O, Can I, Yayla N, Kuskun U, Keles MS. 2012b. Nigella sativa reduces tissue damage in rat ovaries subjected to torsion and detorsion: oxidative stress, proinflammatory response and histopathological evaluation. Gynecol Obstet Invest. 74:41–49.
- Bessems JG, Vermeulen NP. 2001. Paracetamol (acetaminophen)-induced toxicity: molecular and biochemical mechanisms, analogues and protective approaches. Crit Rev Toxicol. 31:55–138.
- Bin Sayeed MS, Shams T, Fahim Hossain S, Rahman MR, Mostofa AGM, Fahim Kadir M, Mahmood S, Asaduzzaman M. 2014. Nigella sativa L. Seeds modulate mood, anxiety and cognition in healthy adolescent males. J Ethnopharmacol. 152:156–162.
- Blantz RC. 1996. Acetaminophen: acute and chronic effects on renal function. Am J Kidney Dis. 28:S3–S6.
- Boskabady MH, Mohsenpoor N, Takaloo L. 2010. Antiasthmatic effect of Nigella sativa in airways of asthmatic patients. Phytomedicine. 17:707–713.
- Bourgou SPA, Marzouk B, Legault J. 2012. Antioxidant, antiinflammatory, anticancer and antibacterial activities of extracts from Nigella sativa (black cumin) plant parts. J Food Biochem. 36:539–546.
- Chattopadhyay RR. 2003. Possible mechanism of hepatoprotective activity of Azadirachta indica leaf extract: Part ii. J Ethnopharmacol. 89:217–219.
- Cobden I, Record CO, Ward MK, Kerr DN. 1982. Paracetamol-induced acute renal failure in the absence of fulminant liver damage. Br Med J (Clin Res Ed). 284:21–22.
- Dahlin DC, Miwa GT, Lu AY, Nelson SD. 1984. N-Acetyl-p-benzoquinone imine: a cytochrome p-450-mediated oxidation product of acetaminophen. Proc Natl Acad Sci USA. 81:1327–1331.
- Das J, Ghosh J, Manna P, Sil PC. 2010. Taurine protects acetaminophen-induced oxidative damage in mice kidney through apap urinary excretion and cyp2e1 inactivation. Toxicology. 269:24–34.
- Dawson JR, Norbeck K, Anundi I, Moldeus P. 1984. The effectiveness of N-acetylcysteine in isolated hepatocytes, against the toxicity of paracetamol, acrolein, and paraquat. Arch Toxicol. 55:11–15.
- Dilger RN, Baker DH. 2007. Oral N-acetyl-l-cysteine is a safe and effective precursor of cysteine. J Anim Sci. 85:1712–1718.
- Draper HH, Hadley M. 1990. Malondialdehyde determination as index of lipid peroxidation. Meth Enzymol. 186:421–431.
- Dwarampudi LP, Palaniswamy D, Nithyanantham M, Raghu PS. 2012. Antipsoriatic activity and cytotoxicity of ethanolic extract of Nigella sativa seeds. Pharmacogn Mag. 8:268–272.
- Effenberger-Neidnicht K, Schobert R. 2011. Combinatorial effects of thymoquinone on the anti-cancer activity of doxorubicin. Cancer Chemother Pharmacol. 67:867–874.
- Eguia L, Materson BJ. 1997. Acetaminophen-related acute renal failure without fulminant liver failure. Pharmacotherapy. 17:363–370.
- Flanagan RJ, Meredith TJ. 1991. Use of N-acetylcysteine in clinical toxicology. Am J Med. 91:131S–139S.
- Ghosh A, Sil PC. 2007. Anti-oxidative effect of a protein from cajanus indicus l against acetaminophen-induced hepato-nephro toxicity. J Biochem Mol Biol. 40:1039–1049.
- Hadjzadeh MA, Khoei A, Hadjzadeh Z, Parizady M. 2007. Ethanolic extract of Nigella sativa L. seeds on ethylene glycol-induced kidney calculi in rats. Urol J. 4:86–90.
- Hadjzadeh MA, Rad AK, Rajaei Z, Tehranipour M, Monavar N. 2011. The preventive effect of n-butanol fraction of Nigella sativa on ethylene glycol-induced kidney calculi in rats. Pharmacogn Mag. 7:338–343.
- Havakhah S, Sadeghnia HR, Hajzadeh MA, Roshan NM, Shafiee S, Hosseinzadeh H, Mohareri N, Rad AK. 2014. Effect of Nigella sativa on ischemia–reperfusion induced rat kidney damage. Iran J Basic Med Sci. 17:986–992.
- Hinson JA, Roberts DW, James LP. 2010. Mechanisms of acetaminophen-induced liver necrosis. Handb Exp Pharmacol. 196:369–405.
- Ismail M, Al-Naqeep G, Chan KW. 2010. Nigella sativa thymoquinone-rich fraction greatly improves plasma antioxidant capacity and expression of antioxidant genes in hypercholesterolemic rats. Free Radic Biol Med. 48:664–672.
- Karakus E, Halici Z, Albayrak A, Bayir Y, Aydin A, Unal D, Cadirci E, Ferah I, Odaci E. 2013. Beneficial pharmacological effects of levosimendan on antioxidant status of acute inflammation induced in paw of rat: involvement in inflammatory mediators. Basic Clin Pharmacol Toxicol. 112:156–163.
- Karakus E, Halici Z, Albayrak A, Polat B, Bayir Y, Kiki I, Cadirci E, Topcu A, Aksak S. 2013. Agomelatine: an antidepressant with new potent hepatoprotective effects on paracetamol-induced liver damage in rats. Hum Exp Toxicol. 32:846–857.
- Kehrer JP. 1993. Free radicals as mediators of tissue injury and disease. Crit Rev Toxicol. 23:21–48.
- Kheradpezhouh E, Panjehshahin MR, Miri R, Javidnia K, Noorafshan A, Monabati A, Dehpour AR. 2010. Curcumin protects rats against acetaminophen-induced hepatorenal damages and shows synergistic activity with n-acetyl cysteine. Eur J Pharmacol. 628:274–281.
- Kokoska L, Havlik J, Valterova I, Sovova H, Sajfrtova M, Jankovska I. 2008. Comparison of chemical composition and antibacterial activity of Nigella sativa seed essential oils obtained by different extraction methods. J Food Prot. 71:2475–2480.
- Kruk I, Michalska T, Lichszteld K, Kladna A, Aboul-Enein HY. 2000. The effect of thymol and its derivatives on reactions generating reactive oxygen species. Chemosphere. 41:1059–1064.
- Kuralay F, Akarca US, Ozutemiz AO, Kutay F, Batur Y. 1998. Possible role of glutathione in prevention of acetaminophen-induced hepatotoxicity enhanced by fish oil in male wistar rats. J Toxicol Environ Health Part A Toxicol Health. 53:223–229.
- Larson AM, Polson J, Fontana RJ, Davern TJ, Lalani E, Hynan LS, Reisch JS, Schiodt FV, Ostapowicz G, Shakil AO, et al. 2005. Acetaminophen-induced acute liver failure: results of a United States multicenter, prospective study. Hepatology. 42:1364–1372.
- Mabrouk A, Ben Cheikh H. 2015. Thymoquinone supplementation reverses lead-induced oxidative stress in adult rat testes. Gen Physiol Biophys. 34:65–72.
- Massey TE, Racz WJ. 1981. Effects of n-acetylcysteine on metabolism, covalent binding, and toxicity of acetaminophen in isolated mouse hepatocytes. Toxicol Appl Pharmacol. 60:220–228.
- Mollazadeh H, Hosseinzadeh H. 2014. The protective effect of Nigella sativa against liver injury: a review. Iran J Basic Med Sci. 17:958–966.
- Mugford CA, Tarloff JB. 1997. The contribution of oxidation and deacetylation to acetaminophen nephrotoxicity in female Sprague–Dawley rats. Toxicol Lett. 93:15–22.
- Nagi MN, Almakki HA, Sayed-Ahmed MM, Al-Bekairi AM. 2010. Thymoquinone supplementation reverses acetaminophen-induced oxidative stress, nitric oxide production and energy decline in mice liver. Food Chem Toxicol. 48:2361–2365.
- Nagi MN, Mansour MA. 2000. Protective effect of thymoquinone against doxorubicin-induced cardiotoxicity in rats: a possible mechanism of protection. Pharmacol Res. 41:283–289.
- Prescott LF. 1983. Paracetamol overdosage. Pharmacological considerations and clinical management. Drugs. 25:290–314.
- Ravindran J, Nair HB, Sung B, Prasad S, Tekmal RR, Aggarwal BB. 2010. Thymoquinone poly (lactide-co-glycolide) nanoparticles exhibit enhanced anti-proliferative, anti-inflammatory, and chemosensitization potential. Biochem Pharmacol. 79:1640–1647.
- Saxena M, Shakya AK, Sharma N, Shrivastava S, Shukla S. 2012. Therapeutic efficacy of Rosa damascena Mill. On acetaminophen-induced oxidative stress in albino rats. J Environ Pathol Toxicol Oncol. 31:193–201.
- Sedlak J, Lindsay RH. 1968. Estimation of total, protein-bound, and nonprotein sulfhydryl groups in tissue with Ellman's reagent. Anal Biochem. 25:192–205.
- Waring WS. 2012. Novel acetylcysteine regimens for treatment of paracetamol overdose. Ther Adv Drug Saf. 3:305–315.
- Whidden MA, Kirichenko N, Halici Z, Erdos B, Foster TC, Tumer N. 2011. Lifelong caloric restriction prevents age-induced oxidative stress in the sympathoadrenal system of Fischer 344 × brown Norway rats. Biochem Biophys Res Commun. 408:454–458.
- Yayla M, Halici Z, Unal B, Bayir Y, Akpinar E, Gocer F. 2014. Protective effect of et-1 receptor antagonist bosentan on paracetamol induced acute liver toxicity in rats. Eur J Pharmacol. 726:87–95.
- Yousef MI, Omar SA, El-Guendi MI, Abdelmegid LA. 2010. Potential protective effects of quercetin and curcumin on paracetamol-induced histological changes, oxidative stress, impaired liver and kidney functions and haematotoxicity in rat. Food Chem Toxicol. 48:3246–3261.
- Zhang J, Brown RP, Shaw M, Vaidya VS, Zhou Y, Espandiari P, Sadrieh N, Stratmeyer M, Keenan J, Kilty CG, et al. 2008. Immunolocalization of kim-1, rpa-1, and rpa-2 in kidney of gentamicin-, mercury-, or chromium-treated rats: relationship to renal distributions of inos and nitrotyrosine. Toxicol Pathol. 36:397–409.