Abstract
Context Oxymatrine (OMT) is beneficial to human health by exerting various biological effects. Objective To investigate the absorption mechanism of OMT and discover absorption enhancers using Madin–Darby canine kidney (MDCK) cell monolayers.
Materials and methods Concentration effects on the transport of OMT were measured in the range of 1.0 × 10−5–1.0 × 10−3 M in 2 h. Then, the effect of time, direction, temperature and pH on the transport of OMT at 10−4 M was studied. Moreover, Papp of OMT was determined in the absence/presence of cyclosporine and surfactants at 100 μM to further confirm the relative transport mechanism.
Results The Papp AP→BL ranged from (3.040 ± 0.23) × 10−6 to (3.697 ± 0.19) × 10−6 cm/s as the concentration varied from 10−5 to 10−3 M. OMT showed similar Papp at 4 and 37 °C (p > 0.05). Increasing the apical pH 7.4 and 8.0 resulted in Papp versus pH 5.0 (p < 0.01). Furthermore, in the presence of cyclosporine and surfactants including sodium citrate, sodium dodecyl sulphate (SDS) and deoxysodium cholate, Papp was (0.318 ± 0.033) × 10−5, (0.464 ± 0.048) × 10−5, (0.897 ± 0.115) × 10−5 and (1.341 ± 0.122) × 10−5 cm/s, respectively. In the presence of surfactants, Papp significantly increased up to 1.5–4.3-fold (p < 0.05).
Discussion and conclusion OMT transport across MDCK cell monolayers was by passive diffusion. Sodium citrate, SDS and deoxysodium cholate serve as excellent absorption enhancers which are useful for the related research improving the oral bioavailability of OMT.
Introduction
Oxymatrine (OMT), a quinolizidine alkaloid from Sophora flavescens Ait. (Leguminosae), is a traditional Chinese medicine with a long history in China (Yuan et al. Citation2003), and has been reported to possess various pharmacological effects. OMT has been extensively used in China for the treatment of viral hepatitis, cancer, cardiac diseases (such as viral myocarditis) and skin diseases (such as psoriasis and eczema) (Wang et al. Citation2005a; Song et al. Citation2006).
In China, OMT, also called kushensu, is frequently used in clinical practice, especially for oral administration. When taken orally, OMT can be reduced to the more absorbable MT by intestinal bacteria in the gastrointestinal tract and liver, which might have pharmacological and toxicological implications in clinical practice (Tang et al. Citation2013). To improve patient compliance, many studies had focused on the development of its oral administration.
However, the development of oral OMT preparations remains a huge challenge. OMT is a water solubility monomer compound. The oral bioavailability of OMT solution was low (only 19.4%), which had been described in our previous investigation (Wang et al. Citation2004). Although the oral bioavailability and bioactivity of some OMT preparations had been improved to some extent compared with OMT solution in above studies, there has no detailed information on the mechanism of its absorption across the human epithelial intestinal barrier (Leppert & Fix Citation1994; Shen & Wang Citation2003).
The experiment was based on animal experiments (Wang et al. Citation2005b), developing a more convenient method which is using in vitro Madin–Darby canine kidney (MDCK) cell monolayer model to clarify the transport mechanism of OMT and foreshadowing the research with a view to subsequent preparations. We selected the MDCK cell line because it has been widely employed as an alternative to Caco-2 model for high throughput drug permeability screening (Horio et al. Citation1989). Besides, compared with Caco-2, MDCK cells express lesser subtypes and the research has shown that in the study of the way of passive diffusion through the intestinal absorption, the optimization results of MDCK are slightly better than Caco-2 (Jiang et al. Citation2002). So, in recent years, the study of the plasma membrane which using MDCK as a model is increasing (Yang et al. Citation2014). However, no previous work about the OMT transport system in the MDCK cell has been reported. Therefore, the objectives of this study were to further determine whether OMT is absorbed by passive way in MDCK cell and explore the effect of time, concentration, temperature, pH and absorption enhancers on the transport of OMT. Our study may shed light on the successful development of OMT oral formulations in the future.
Materials and methods
OMT (>99% purity) was purchased from the National Institute for the Control of Pharmaceutical and Biological Products (Beijing, China). Acetonitrile was of HPLC grade from Fisher Scientific (Pittsburgh, PA). Dulbecco’s modified Eagle’s medium (DMEM), foetal bovine serum (FBS), non-essential amino acids solution (NEAA), 100 U/mL penicillin and 100 μg/mL streptomycin solution, trypsin solution, cyclosporine were obtained from Invitrogen (Carlsbad, CA). 3-(4,5-Dimethylthiazol-2-yl)-2,5-diphenyltetrazolium bromide (MTT) was purchased from Sigma-Aldrich (St. Louis, MO). Twenty-four well Millicell (0.6 cm2 and 0.1 μm) was purchased from Millipore Corporation (Milford, MA). Sodium dodecyl sulphate (SDS), sodium citrate and deoxysodium cholate were purchased from NICPBP (Beijing, China). Distilled, deionized water was purified using a Milli-Q system (Millipore). All other chemical products were commercially available and of analytical reagent grade.
Analysis methods
The quantification of OMT was performed by Shimadzu 2010A LC-MS system (Shimadzu Company, Japan) with ESI probe (Q-array-Octapole-Quadrupole mass analyser). The method of detection was described previously (Wang et al. Citation2005a).
Cell culture
The MDCK cell lines were obtained from the Type Culture Collection of the Chinese Academy of Sciences (Shanghai, China). Cells were grown and maintained in DMEM supplemented with 10% FBS, 1% NEAA, 100 U/mL penicillin and 100 μg/mL streptomycin solutions. The cultures were kept at 37 °C, 5% CO2 humidified incubator.
For transport studies, cells were seeded at a density of 5 × 104 cells/cm2 onto 24-well Millicell. The medium was replaced every day. Cell attachment and growth were monitored by phase-contrast microscopy. Following incubation in a period of 6–8 days in culture, the cell monolayers were used for drug transport experiments. MDCK cells with the passage numbers of 70–78 were used in this study. The formation of confluent monolayers and tight junctions was confirmed by measuring the paracellular transportation of fluorescein and the transepithelial electrical resistance (TEER) using a Millicell ERS® voltohmmeter (Millipore). The MDCK cell monolayers that displayed a TEER of 200–300 Ω cm2 with the Papp value of fluorescein of 1–3 × 10−6 cm/s were used in the experiments.
Cytotoxicity study
Cytotoxicity of OMT was evaluated by MTT (methyl tetrazolium) assay. MDCK cells were seeded in 96-well plates (Corning) at a density of 5 × 104 cells/well and cultured for 24 h. Subsequently, OMT was added to the wells at various concentrations including 10−6, 10−5, 10−4, 10−3 and 10−2 mol/L. After 2 h of co-incubation at 37 °C, 100 μL of fresh MTT solution (0.5 mg/mL) was added the cells that were incubated for a further 4 h. After incubation, the medium was removed, and 150 μL of dimethylsulphoxide (DMSO) was added to the residual precipitates. The absorbance of the resulting DMSO solution was determined at a wavelength of 560 nm using an ELISA reader. Cell viability was expressed as a percentage of the absorbance relative to that of the control. Control cells were not exposed to any materials, which represented 100% cell viability. Experiments were performed with five replicate wells for each sample and control (Twentyman & Luscombe Citation1987).
TEER measurement
The TEER was measured before and after the transport experiment. After the experiment the monolayers were washed three times with HBSS and filled up with complete medium for 24 h and the TEER values were measured to evaluate the regeneration of cell monolayers. The TEER values measured before the experiment were taken as the initial values expressed as 100%. All subsequent TEER values are expressed as a percentage of the initial value. The TEER values (Ω cm2) were calculated according to the following equation:
where R is the measured resistance across a cell monolayer, Rblank is the resistance of a blank well and A is the surface area of the Millicell filter (0.6 cm2).
Transport studies
Transepithelial transport was studied with MDCK cells grown on Millicell for 6–8 days. Hank’s buffered salt solution (HBSS) (137 mM NaCl, 1.0 mM CaCl2, 5.4 mM KCl, 0.8 mM MgSO4, 0.4 mM KH2PO4 and 0.4 mM Na2HPO4) was used as the transport medium. The cell monolayers were rinsed three times with HBSS and pre-incubated for 30 min at 37 °C with HBSS before use. At the beginning of the experiment, transport medium containing OMT was added to the apical (0.4 mL) or basolateral (0.6 mL) compartment. Samples (100 μL) were withdrawn from the receiver chamber at pre-determined time points 15, 30, 45, 60, 90, 120 min, and replaced with equal volume of fresh HBSS buffer solution to maintain sink conditions. Dilutions were taken into account for calculations of permeant concentrations. At the selected times, the flux across the cell monolayer was determined under various conditions. The concentrations of OMT were determined. All experiments were performed at 37 °C.
The apparent permeability coefficient Papp (cm/s) of the compounds was calculated by the following equation:
where dQ/dt is the increase in the amount of drug in the receiver chamber per time interval, C0 is the initial concentration in the donor compartment and A is the permeation area of the cell culture insert which is 0.6 cm2 in this study.
Statistical analysis
All results are expressed as mean ± SD (standard deviation) of three replicates. Statistical comparisons were performed by Student’s t-test for two groups with a p value of less than 0.05 or 0.01 being considered statistically significant.
Results
Validation of the method
Selectivity, linearity, precision and stability of OMT determination
The retention time of OMT was approximately 1.5 min. The calibration curve was constructed in the range 0.005–4.0 μg/mL for OMT. The regression equation of this curve and their correlation coefficients (r) was calculated as follows: y = 0.0037x + 0.0351 (r = 0.9997). It showed good linear relationships between the peak areas and the concentrations. Signals 3 and 10 times higher than the peak noise height were regarded as the detection and quantification limits. The detection and quantification limits were 0.6 and 5 ng/mL, respectively. The reproducibility of the method was defined by examining both intra- and inter-day variance. The intra-day and inter-day CV data of OMT assays at low to high concentrations were within 15%. All stability results are shown in . OMT was stable when stored at pH more than 5.0 (p > 0.05).
Table 1. Stability of OMT (1 μg/mL) in different pH Hanks (mean ± SD, n = 5).
Cytotoxicity study
The potential cytotoxicity of OMT on MDCK cells was evaluated by the MTT assy. The cell viability of MDCK cells treated with OMT at 10−6, 10−5, 10−4, 10−3 and 10−2 mol/L in MTT assay was 99.12 ± 5.13%, 99.67 ± 4.22%, 98.48 ± 5.18%, 97.66 ± 5.08% and 94.89 ± 6.36%, respectively. MDCK cells viability was still more than 90% even at a dose of 10−2 mol/L of OMT, which is considered to be an acceptable level of biocompatibility. Results of the MTT assay showed that OMT over a concentration range of 10 6–10−2 mol/L had no apparent cytotoxicity on MDCK cells.
Time and concentration effect of OMT transport
To confirm whether OMT transport has character of saturation, the time course of OMT (10−4 M, 2 h) transport across MDCK cell monolayer was studied at 37 °C in the apical to basolateral (AP-to-BL) direction (). The transport amount of OMT increased linearly from 0 to 2 h with no latent period (R2 = 0.99), indicating that non-saturated phenomenon occurred over the time period studied.
Figure 1. Time effects on the transport of OMT (10−4 M) across MDCK cell monolayers on AP side at 37 °C. Each point represents the mean ± SD of three different cell monolayers.
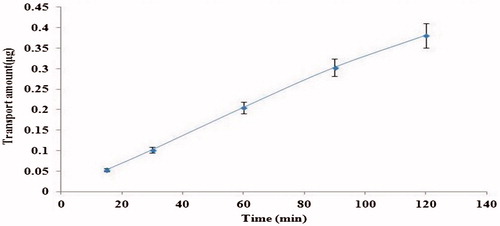
The effect of concentration on the OMT transporting across MDCK cell monolayers in the AP-to-BL direction after 2 h incubation at 37 °C was investigated (). The transport amount of OMT exhibited acceptable linearity over the concentration range from 1.0 × 10−5 to 1.0 × 10−3 mol L−1 and was not saturated over the entire concentration range examined. The typical regression equation (n = 5) was y = 4.28x−0.0151 (r = 0.9999), where y represents transport amount of OMT and x represents the concentration. It showed that the cumulative permeation amount of OMT crossing the cell monolayer was gradually increased with the increasing concentration in AP side. These results showed that OMT transport across MDCK cell monolayers were concentration dependent, which were the typical characteristics of passive diffusion. As shown in , transport significantly diminished as concentration was lowered. Therefore, the middle concentration was selected for subsequent OMT transport experiments unless otherwise mentioned. Moreover, Papp of OMT at different concentration and directions across MDCK monolayer shown in , which indicated that the efflux ratio (PBL-AP/PAP-BL) of OMT did not rise with the concentration and its value was in the range of 0.97–1.0 (p > 0.05), which suggested that passive diffusion was the main mechanism.
Figure 2. Concentration effects on the transport of OMT across MDCK cell monolayers. OMT transport amount was measured at 37 °C after 120 min incubation. Data are presented as mean ± SD of three different cell monolayers.
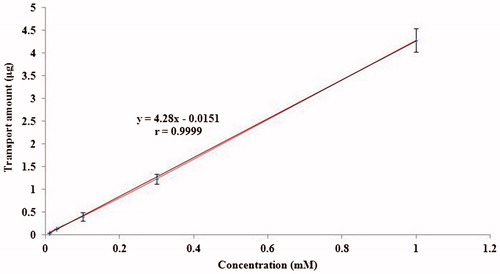
Figure 3. Concentration effects on the transport of OMT across MDCK cell monolayers. Cells were incubated with OMT 10−4 M from AP to BL compartment for 2 h at 37 °C. Each point represents the mean ± SD of three different cell monolayers.
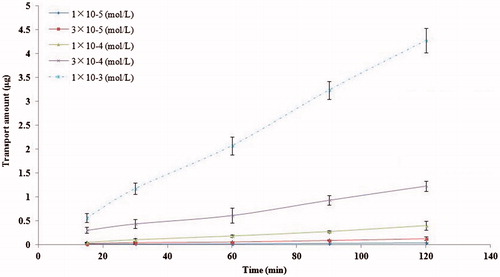
Table 2. Papp of OMT at different concentration across MDCK monolayers (Mean ± SD, n = 3).
Temperature dependence of OMT transport
In order to determine the possible involvement of an active transport mechanism in OMT transport across MDCK cell monolayers, the Papp of OMT (10−4 M, 2 h) in the AP-to-BL direction was measured at 37 and 4 °C, respectively. Changing temperature should have no or slight effect on their transport because passive diffusion does not require energy. As shown in , the transport of OMT was slightly decreased (p > 0.05). Taken together, OMT transport across in vitro cell monolayers was not energy-dependent, which is similar to that of passive diffusion markers. All of these indicated that the mechanism of OMT absorption across MDCK cell monolayers was passive transport.
Effect of buffer pH and different materials on the transport of OMT
The Papp of OMT (10−4 M) in the AP-to-BL direction was measured at pH 5, 6.5, 7.4 and 8, respectively. clearly indicates that the transport of OMT increased as the buffer pH was raised from 5.0 to 8.0. Experiments demonstrated that high pH value (7.4, 8.0) can promote transport of OMT (p < 0.01). Therefore, we can also try to combine the OMT with alkaline drugs to observe the absorption.
Figure 5. Effect of pH on OMT (10−4 M) transport across MDCK cell monolayers. Transport of OMT was measured at 37 °C for 30 min. Asterisks signs denote significant differences; **p < 0.01 versus control (pH 5).
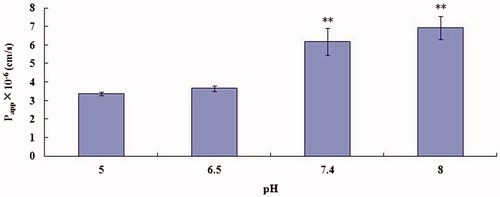
To further confirm the passive transport mechanism of OMT across MDCK cell monolayers and to evaluate the potential utility of absorption enhancers in future formulation design, the Papp of OMT (10−4 M, 2 h) in the AP-to-BL direction at 37 °C was measured after the tight junction of cells were opened (shown in ). In this experiment, OMT transport was studied in the presence of cyclosporine, sodium citrate, SDS and deoxysodium cholate, Papp of OMT was reached to (0.318 ± 0.033) × 10−5, (0.464 ± 0.048) × 10−5, (0.897 ± 0.115) × 10 − 5 and (1.341 ± 0.122) × 10−5 cm/s, respectively. Meanwhile, cyclosporine showed no significant difference (p > 0.05); sodium citrate, SDS and deoxysodium cholate increased the Papp up to 1.5-, 2.7- and 4.3-fold, respectively. These data indicated that sodium citrate, SDS and deoxysodium cholate at these concentrations were proved to significantly increase OMT transport, the order of enhancement effect was deoxysodium cholate > SDS > sodium citrate.
Figure 6. Effect of transport enhancers on Papp of OMT (10−4 M) across MDCK cells. Transport of OMT was measured at 37 °C for 15 min. Results are expressed as mean ± SD of three different cell monolayers. Asterisks signs denote significant differences; *p < 0.05 versus control; **p<0.01 versus control.
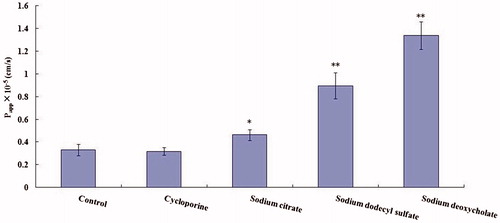
Discussion
In this study, MDCK cell monolayer was chosen as in vitro model. Since oral absorption capacity is a crucial selection criterion at the early stage of drug development and formulation screening, there is an urgent need for quick and reliable drug absorption assessment models. Although Caco-2 cell monolayers have been approved by U.S. Food and Drug Administration (FDA) as a standard permeability screening assay to predict the absorption of subtotal drugs, it is inaccurate for prediction of drugs absorbed paracellularly or via delivery carrier in many cases because of its ‘tighter’ tight junctions compared with the small intestine, MDCK cell derived from a normal male cocker spaniel, could differentiate into columnar epithelium and form tight junctions when cultured on semi-permeable membranes. Its main advantages include the shorter cell culture times (7 days) compared to Caco-2 cell (21 days), and the similar tight junctions of cell monolayers to that of physiological small intestine. MDCK cell monolayer has been considered as an alternative for Caco-2 for studying absorption capacity and transport mechanisms of passive transport drugs in small intestine, especially for paracellular passive transport drugs (Artursson & Borchardt Citation1997). Therefore, MDCK cells were used in this study.
The first step of this study was to demonstrate whether active transport and endocytosis transport are involved in the OMT transport across MDCK cell monolayers. Experimental results of time and concentration effect showed that the cumulative permeation amount of OMT was increased linearly with the concentration or time increasing, and no saturation phenomenon was observed over all time period and concentration range, which were the typical characteristics of passive diffusion. Results of temperature effect and bidirectional transport showed that OMT transport across MDCK cell monolayers cannot be significantly affected by temperature change and it is not energy dependent, which is similar to passive diffusion markers. Results of pH effect showed that the transport of OMT was increased as the buffer pH was raised from 5.0 to 8.0 and maximal at pH 8.0 (Luo et al. Citation2006). Cyclosporine is a classical P-glycoprotein inhibitor (O’Brien et al. Citation2014). In this experiment, cyclosporine group showed a similar Papp compared with the control group (p > 0.05), which demonstrated that OMT was not the P-glycoprotein substrates. Experiment to evaluate the potential utility of absorption enhancers is useful for the future formulation designed to increase OMT bioavailability (Varma et al. Citation2003).
The way of transportation divided into two main pathways: the paracellular pathway between the cells and transcellular pathway through the cells. Transcellular transport often involves energy expenditure, whereas paracellular transport is unmediated and passive down a concentration gradient. Paracellular transport also has the benefit that absorption rate is matched to load because it has no transporters that can be saturated (Balkovetz Citation2006; Tervonen et al. Citation2014). The paracellular pathway allows the permeation of hydrophilic molecules which are not able to permeate through the lipid membrane by the transcellular pathway of absorption. Tight junctions in the intestinal epithelium are also known to be size-selective, such that large molecules (with molecular radii less than about 4.5 Å) are included (Fromter & Diamond Citation1972; Chediack et al. Citation2003; Turner et al. Citation2014). This is particularly important for hydrophilic pharmaceuticals, which may not have affinity for membrane-bound transporters, and therefore, it may be excluded from the transcellular pathway. The vast majority of drug molecules are transported through the transcellular pathway, and few rely on the paracellular pathway of transportation typically have a much lower bioavailability.
In this paper, OMT is a water-soluble, micro-molecule, traditional Chinese medicine monomer drug, and is mainly by passive paracellular pathway.
After being treated by absorption enhancers to open the tight junction of MDCK cell monolayers, the Papp of OMT was considerably increased comparing with that in untreated cell monolayers. These data indicated that sodium citrate, SDS and deoxysodium cholate on these concentrations were proved to significantly increase OMTtransport. The mechanism of these absorption enhancers as follows: The Ca2+ dependent of occluding junction loosed and the penetrability improved was made by sodium citrate. The mechanism of sodium citrate may have relation with interaction of Ca2+ and it exerted an impact on the forms of a tight connection between the MDCK cells, which made the gaps of the cells to increased and this effect contributed to the increasing of OMT cross the cell gaps and thus, the absorption of OMT was promoted. The mechanisms of the increasing drug across epithelial of sodium deoxycholate were various in literature described, mainly including forming reverse micelles which attributes to form a polar channel that allows the polarity drug diffusion through the plasma membrane, reducing the viscosity of the mucous layer on the surface of the epithelium that makes the drug easily spread through the plasma membrane, interacting with Ca2+ that affects the formation of a tight connection between the cells and this increases the gaps of the cells, consequently, the penetrability is increased, reversing multidrug resistance factor. The concentration of sodium deoxycholate used in the test was 100 μM less than the formation of the critical concentration of 5 mM, therefore, the increasing of penetrability of OMT may not be due to micelles formation. And TEER reversibility reduced was observed in the process of the test. TEER values within 8 h return to the original level. The results suggested that absorption enhancers increased the penetrability of OMT may through slackening the tight connection.
Conclusion
In this study, the transepithelial transport capacity, properties and mechanism of OMT across MDCK cell monolayers model were investigated for the first time. OMT transport across the MDCK cell models was concentration dependent, direction and energy independent, and can be significantly improved when the tight junction of cells opened. These transport characteristics suggested that OMT transport across the intestinal epithelium mainly by passive pathway. And sodium citrate, SDS and deoxysodium cholate may be potential excipients to improve oral efficacy of OMT. This study is useful for designing oral formulation for OMT with improving bioavailability.
Funding information
This work was supported by National Natural Science Foundation of China (81073141), Guangdong Provincial Natural Science Foundation (9152402301000007) and Guangdong Province ‘12-5’ medical key subject.
Disclosure statement
The authors declare no conflicts of interest.
References
- Artursson P, Borchardt RT. 1997. Intestinal drug absorption and metabolism in cell cultures: Caco-2 and beyond. Pharm Res. 14:1655–1658.
- Balkovetz DF. 2006. Claudins at the gate: determinants of renal epithelial tight junction paracellular permeability. Am J Physiol Renal Physiol. 290:572–579.
- Chediack JG, Caviedes-Vidal E, Fasulo V, Yamin LJ, Karasov WH. 2003. Intestinal passive absorption of water-soluble compounds by sparrows: effect of molecular size and luminal nutrients. J Comp Physiol B Biochem Syst Environ Physiol. 173:187–197.
- Fromter E, Diamond J. 1972. Route of passive ion permeation in epithelia. Nat New Biol. 238:9–13.
- Horio M, Chin KV, Currier SJ, Goldenberg S, Williams C, Pastan I, Gottesman MM, Handler J. 1989. Transepithelial transport of drugs by the multidrug transporter in cultured Madin–Darby canine kidney cell epithelia. J Biol Chem. 264:14880–14884.
- Jiang XH, Jia YT, Yuan Y. 2002. Application of Caco-2 cell model in the study of oral drug absorption process. Chin Pharm J. 37:325–327.
- Leppert PS, Fix JA. 1994. Use of everted intestinal rings for in vitro examination of oral absorption potential. J Pharm Sci. 83:976–81.
- Luo S, Kansara VS, Zhu X, Mandava NK, Pal D, Mitra AK. 2006. Functional characterization of sodium-dependent multivitamin transporter in MDCK-MDR1 cells and its utilization as a target for drug delivery. Mol Pharm. 3:329–339.
- O'Brien FE, O'Connor RM, Clarke G, Donovan MD, Dinan TG, Griffin BT, Cryan JF. 2014. The P-glycoprotein inhibitor cyclosporin A differentially influences behavioural and neurochemical responses to the antidepressant escitalopram. Behav Brain Res. 261:17–25.
- Shen K, Wang JT. 2003. Progress in experimental methods for study of drugs’ intestinal absorption. Chin J New Drugs. 12:988–991.
- Song G, Luo Q, Qin J, Wang L, Shi Y, Sun C. 2006. Effects of oxymatrine on proliferation and apoptosis in human hepatoma cells. Colloids Surf B Biointerfaces. 48:1–5.
- Tang L, Dong LN, Peng XJ, Li Y, Zhou FY, Liu ZQ. 2013. Pharmacokinetic characterization of oxymatrine and matrine in rats after oral administration of radix Sophorae tonkinensis extract and oxymtrine by sensitive and robust UPLC–MS/MS method. J Pharm Biomed Anal. 83:179–185.
- Tervonen A, Vainio I, Nymark S, Hyttinen J. 2014. Prediction of passive drug permeability across the blood-retinal barrier. Pharm Res. 31:2297–2311.
- Turner JR, Buschmann MM, Romero-Calvo I, Sailer A, Shen L. 2014. The role of molecular remodeling in differential regulation of tight junction permeability. Semin Cell Dev Biol. 36:204–212.
- Twentyman PR, Luscombe MA. 1987. A study of some variables in a tetrazolium dye (MTT) based assay for cell growth and chemosensitivity. Br J Cancer. 56:279–85.
- Varma MV, Ashokraj Y, Dey CS, Panchagnula R. 2003. P-glycoprotein inhibitors and their screening: a perspective from bioavailability enhancement. Pharmacol Res. 48:347–359.
- Wang SJ, Wang GJ, Li XT, Sun JG, Ma RL, Sheng LS. 2004. Pharmacokinetics of oxymatrine in Beagle dogs by LC–MS. Chin J Clin Pharmacol Ther. 25:585–587.
- Wang SJ, Wang GJ, Li XT, Ma RL, Sheng LS, Sun JG. 2005a. Pharmacokinetics of oxymatrine and its metabolite in beagle dogs detected by LC–MS. J Chin Mataria Med. 30:133–136.
- Wang SJ, Wang GJ, Li XT, Ma RL, Sun JG, Sheng LS. 2005b. Simultaneous determination of oxymatrine and its active metabolite matrine in dog plasma by liquid chromatography–mass spectrometry and its application to pharmacokinetic studies. J Chromatogr B. 817:319–325.
- Yang JH, Hou YH, Ji GJ, Song Z, Liu Y, Dai G, Zhang Y, Chen J. 2014. Targeted delivery of the RGD-labeled biodegradable polymersomes loaded with the hydrophilic drug oxymatrine on cultured hepatic stellate cells and liver fibrosis in rats. Eur J Pharm Sci. 52:180–190.
- Yuan J, Zhang ZJ, Cong B. 2003. Biological activity of matrine and its research progress. Pesticides. 42:1–4.