Abstract
Context Chagas’ disease and leishmaniasis produce significant disability and mortality with great social and economic impact. The genus Stevia (Asteraceae) is a potential source of antiprotozoal compounds.
Objective Aerial parts of four Stevia species were screened on Trypanosoma cruzi. Stevia satureiifolia (Lam.) Sch. Bip. var. satureiifolia (Asteraceae) dichloromethane extract was selected for a bioassay-guided fractionation in order to isolate its active compounds. Additionally, the antileishmanial activity and the cytotoxicity of these compounds on mammalian cells were assessed.
Materials and methods The dichloromethane extract was fractionated by column chromatography. The isolated compounds were evaluated using concentrations of 0–100 μg/mL on T. cruzi epimastigotes and on Leishmania braziliensis promastigotes for 72 h, on trypomastigotes and amastigotes of T. cruzi for 24 h and 120 h, respectively. The compounds’ cytotoxicity (12.5–500 μg/mL) was assessed on Vero cells by the MTT assay. The structure elucidation of each compound was performed by spectroscopic methods and HPLC analysis.
Results The dichloromethane extracts of Stevia species showed significant activity on T. cruzi epimastigotes. The flavonoids eupatorin (1.3%), cirsimaritin (1.9%) and 5-desmethylsinensetin (1.5%) were isolated from S. satureiifolia var. satureiifolia extract. Eupatorin and 5-desmethylsinensetin showed IC50 values of 0.2 and 0.4 μg/mL on T. cruzi epimastigotes and 61.8 and 75.1 μg/mL on trypomastigotes, respectively. The flavonoid 5-desmethylsinensetin showed moderate activity against T. cruzi amastigotes (IC50 value = 78.7 μg/mL) and was the most active compound on L. braziliensis promastigotes (IC50 value = 37.0 μg/mL). Neither of the flavonoids showed cytotoxicity on Vero cells, up to a concentration of 500 μg/mL.
Introduction
Chagas' disease results in significant disability with great social and economic impact, including under- and unemployment. About 6–7 million people are estimated to be infected worldwide, mostly in Latin America with almost 12 000 deaths annually (DNDi Citation2015a; WHO Citation2015). Although endemic in 21 countries across Latin America, the number of affected people is growing in non-endemic, developed countries due to increased migration of Latin American people unknowingly carrying the parasite (Rodriguez Coura & Albajar Viñas Citation2010). Chagas' disease is the most important endemic disease in Argentina with 1.6–2 million people being infected. This parasite disease affects mainly people living in rural areas, where the vector lives, and people from urban centres due to migration phenomena (Franco-Paredes et al. Citation2009, Rassi et al. Citation2010).
Leishmaniasis is another poverty-associated disease responsible for different clinical forms, the most common being visceral leishmaniasis (VL) and cutaneous leishmaniasis (CL). The most common form in our country is the CL caused mainly by L. braziliensis, but since 2006, human and canine VL cases have been reported (Gould et al. Citation2013). Mixed infections caused by Leishmania spp. and T. cruzi have been reported in Argentina and Brazil (Chiaramonte et al. Citation1999; Frank et al. Citation2003).
Current treatment for Chagas' disease is limited to two drugs, benznidazol and nifurtimox, which were developed around 30 years ago. These two drugs are effective in the acute phase of infection and present drawbacks associated to side effects, lack of effectiveness and long treatment periods (DNDi Citation2015b). Leishmaniasis treatment includes pentavalent antimonials (sodium stibogluconate and meglumine antimoniate), amphotericin B, miltefosine and paromomycin. These drugs have limitations due to serious side effects, resistance, long duration of treatment, low tolerability, difficult administration and high cost (DNDi Citation2015b).
In recent years, considerable attention has been given to secondary metabolites from plants in the search for new trypanocidal and leishmanicidal compounds. Among these metabolites, terpenes and flavonoids have been pointed out as promising molecules (Schmidt et al. Citation2012).
The genus Stevia (Asteraceae) is a New World genus, distribution ranging from the Southern United States to Northeastern Paraguay, Southeastern Brazil and the South American Andean region (Kinghorn Citation2002). One member of this family, Stevia rebaudiana (Bertoni) Bertoni (Asteraceae) is of worldwide economic importance today since its ent-kaurene diterpene glycosides are used as non-nutritive natural sweeteners, mainly in Japan. This genus is rich in sesquiterpene lactones and flavonoids among which, methoxylated aglycones at the C-6 position have been reported (Cerda-García Rojas & Pereda-Miranda Citation2002). Flavonoids of this type, such as hispidulin and santin, have been reported to have trypanocidal and leishmanicidal activities (Sülsen et al. Citation2007).
In Argentina, the genus Stevia is represented by more than 30 species and several varieties growing in the Northern and Central areas of the country (Zuloaga et al. Citation2008). Among the native species, the chemical composition and bioactivity of Stevia aristata D. Don ex Hook. &Arn., S. multiaristata Spreng., S. entreriensis Hieron. and S. satureiifolia (Lam.) Sch. Bip. var. satureiifolia have not been investigated so far. The only literature data available on the chemical composition of S. aristata were published by Zdero et al. (Citation1987) in which beyerene derivatives and other terpenoids were reported. Thus, the potential of these species is still unexplored.
In this work, four native Stevia species were screened for their antiprotozoal activity on Trypanosoma cruzi. One of the most active species was selected for a bioassay-guided fractionation in order to isolate its active compounds. Additionally, the antileishmanial activity and the cytotoxicity of these compounds on mammalian cells were assessed.
Materials and methods
General experimental procedures
UV spectra were recorded on a Jasco-630 spectrophotometer (Jasco Tools, Inc., Rochester, NY). MS spectra were obtained in an Agilent 5973 spectrometer (Agilent Technologies, Inc., Santa Clara, CA). HPLC analyses were performed in a Varian Pro Star instrument (Beckman Instruments Inc., Irvine, CA) equipped with a Rheodyne injection valve (20 μL) and a diode array detector (DAD) set at 340 nm. Chromatograms were recorded and processed using the Varian Star Chromatography Workstation version 6.x (Beckman Instruments Inc., Irvine, CA). The water employed to prepare the mobile phase was of ultrapure quality (Milliq). Methanol (J. T. Baker) was of HPLC grade. Eupatorin and cirsimaritin (Sigma, St. Louis, MO) were used as standards for HPLC analysis. Silicagel (Merck, Darmstadt, Germany, 230–400 mesh) was used for column chromatographic separation and Silicagel 60 F254 (Merck, Darmstadt, Germany) was used for TLC analysis.
Plant material
The aerial parts of Stevia aristata D. Don ex Hook. & Arn. (Asteraceae) (741) and Stevia entreriensis Hieron. (Asteraceae) (762) were collected in the province of Entre Ríos, Argentina in November 2011 and March 2012, respectively. The aerial parts of Stevia satureiifolia (Lam.) Sch. Bip. var. satureiifolia (Asteraceae) (744) and Stevia multiaristata Spreng. (Asteraceae) (742) were collected in the province of Buenos Aires, Argentina, in February 2012.
The plant material was collected and identified by one of the authors (Gustavo Giberti Ph.D.) and voucher specimens were deposited at the Museo de Farmacobotánica, Facultad de Farmacia y Bioquímica (BAF), Universidad de Buenos Aires.
Parasites
Trypanosoma cruzi epimastigotes (RA strain) were grown in a biphasic medium. Leishmania braziliensis promastigotes (MHOM/BR/75/M2903) were grown in liver infusion tryptose medium (LIT). Cultures were routinely maintained by weekly passages at 28 °C and 26 °C, respectively.
Plant extracts
For the preparation of plant extracts for screening, 10 g of the dried aerial parts of each Stevia species were extracted by maceration (24 h) twice with 100 mL of dichloromethane (DCM). The extracts were filtered and taken to dryness. The extraction of S. satureiifolia var. satureiifolia (aerial parts) for bioassay-guided fractionation was performed using 500 g of plant material and DCM (5 L) as described before above.
HPLC analysis of S. satureiifolia var. satureiifolia dichloromethane extract (DCME)
A chromatografic profile of S. satureiifolia var. satureiifolia DCME was obtained using a Kinetex XB-C-18 100 Å (250 mm × 46 mm × 5 μm) column eluted with a gradient of H2O:AcOH (98:2) (A) and MeOH:AcOH (98:2) (B) from 50% B to 80% B in 40 min. The flow rate employed was 1.2 mL/min. The DCME (5 mg) was dissolved in MeOH:H2O (9:1) to a final concentration of 5 mg/mL.
Fractionation and isolation of compounds from S. satureiifolia var. satureiifolia extract
Stevia satureiifolia var. satureiifolia DCME (2.5 g) was fractionated by column chromatography (CC) (400 mm × 30 mm) on Silicagel 60 (50 g) with a gradient of DCM and ethyl acetate (EtOAc): DCM 100%, DCM:EtOAc 9:1, 3:1, 2:1, 1:1, 1:3, EtOAc 100%. Seven fractions (SS1–SS7) of 100 mL each were collected and taken to dryness in a rotatory evaporator. Each fraction was tested for trypanocidal activity on T. cruzi epimastigotes. A yellow precipitate (compound 3) (10 mg) was obtained from fraction SS2 eluted with DCM: EtOAc 9:1. The TLC analysis of fractions SS4 and SS5, eluted with DCM:EtOAc 2:1 and 1:1, respectively, was performed on Silicagel 60 F254, developed with hexane:EtOAc (1:9) and Natural Products Reagent (Sigma, ST. Louis, MO) as a revealing agent. Fraction SS5 (50 mg) was subjected to CC (240 mm × 20 mm) on Silicagel 60 (10 g) eluted with a gradient of DCM and EtOAc: DCM 100%, DCM:EtOAc 9:1, 7:3, 5:5, 3:7, 2:8, 1:9, EtOAc 100%, to obtain eight subfractions (SS5A–SS5H). From subfractions SS5B–SS5D eluted with DCM:EtOAc 9:1, 7:3, 5:5, 3:7, an amorphous powder (6 mg) was obtained (compound 1). This compound was filtered and washed three times with hexane and EtOAc. The purity of compounds 1 and 3 was assessed by HPLC and the compounds were assayed for trypanocidal and leishmanicidal activities.
Identification of compounds
The structure elucidation of compound 3 was performed by UV spectroscopy with the addition of diagnostic reagents (Mabry et al. Citation1970), by proton nuclear magnetic resonance (1H NMR) and by electron impact-mass spectrometry (EI-MS). Spectra were compared with those found in the literature.
Identification of compound 1 was performed by HPLC/DAD against a eupatorin standard. Each sample was dissolved in MeOH:H2O (9:1). Three different chromatographic systems were used: (1) a Phenomenex column C-18 Luna (2) (250 mm × 4.6 mm × 5 μm) eluted with a gradient of H2O:HCOOH (19:1) (A) and MeOH (B) from 50% B to 100% B in 35 min. The flow rate was set at 1 mL/min. (2) The same column as that used in system 1 eluted with a gradient of H2O:AcOH (98:2) (A) and MeOH:AcOH (98:2) (B) from 15% B to 100% B in 60 min. The flow rate was set at 1.2 mL/min. (3) The system was the same as that used for HPLC analysis of S. satureiifolia var. satureiifolia DCME.
Compound 2 was identified in the DCME by HPLC/DAD against a cirsimaritin standard, using the same three solvent systems as those used for compound 1.
Quantification of compounds 1–3 in S. satureiifolia var. satureiifolia DCME
The quantification of compounds 1–3 was performed by HPLC/DAD using system 3. Eupatorin, cirsimaritin (Sigma, St. Louis, MO) and the isolated compound 3 were used as a reference and 1 mg of each compound was dissolved in 25 mL of a mixture MeOH:H2O (9:1). The DCME (5 mg) was dissolved in the same solvent mixture to a final concentration of 5 mg/mL.
Activity assay on T. cruzi and L. braziliensis
The growth inhibition of T. cruzi epimastigotes and L. braziliensis promastigotes was evaluated by a [3H]-thymidine uptake assay as previously described (Sülsen et al. Citation2008). Parasites were adjusted to a cell density of 1.5 × 106/mL and cultured in the presence of Stevia spp. extracts, S. satureiifolia var. satureiifolia fractions (100 and 10 μg/mL) and compounds 1 and 3 (100–0.1 μg/mL) for 72 h. Benznidazole (5–20 μM; Elea) and Amphotericin B (0.27–1.6 μM; ICN) were used as positive controls. Radioactivity was measured as counts per minute (cpm). The percentage of inhibition was calculated as 100 − {[(cpm of treated parasites)/(cpm of untreated parasites)] × 100}.
The trypanocidal effect of compounds 1 and 3 was also tested on bloodstream trypomastigotes as previously described (Sülsen et al. Citation2008). Briefly, mouse blood containing trypomastigotes was diluted in complete LIT medium to adjust the parasite concentration to 1.5 × 106/mL. Parasites were seeded (150 μL/well) by duplicate in a 96-well microplate, and 2 μL of each compound (0–100 μg/mL, final concentration) were added per well. Plates were incubated for 24 h at 4 °C and the remaining live parasites were counted in a haemocytometer. Live trypomastigotes were calculated as {[(live parasites in wells after compound treatment)/(live parasites in untreated wells)] × 100}.
To evaluate the effect of the compounds 1 and 3 on intracellular forms of T. cruzi, 96-well plates were seeded with the non-phagocytic Vero cells at 5 × 103 per well in 100 μL of culture medium and incubated for 2 h at 37 °C in a 5% CO2 atmosphere. Cells were washed and infected with transfected bloodstream trypomastigotes expressing β-galactosidase (Sülsen et al. Citation2013) at a parasite:cell ratio of 10:1. After 24 h of co-culture, plates were washed twice with PBS to remove extracellular parasites and each pure compound was added at 1–100 μg/mL per well in 150 μL of fresh complete RPMI medium without phenol red (Gibco, Rockville, MD). Controls included infected non-treated cells (100% infection control) and uninfected cells (0% infection control). The assay was developed 5 d later by the addition of chlorophenolred-β-d-galactopyranoside (CPRG) (100 μM) and 1% Nonidet P40. Plates were then incubated for 4–6 h at 37 °C and the absorbance was measured at 570 nm in a microplate reader (Bio-Rad Laboratories, Hercules, CA). The percentage inhibition was calculated as 100–{[(absorbance of treated infected cells)/(absorbance of untreated infected cells) × 100} and the IC50 value was estimated.
Cytotoxicity assay
Vero cells were employed to determine the viability by the 3-(4,5-dimethylthiazol-2yl)-2,5-diphenyltetrazolium bromide (MTT) method (Sülsen et al. Citation2011). Cells (5 × 105) were settled at a final volume of 150 μL in a flat-bottom 96-well microplate and were cultured at 37 °C in a 5% CO2 atmosphere in the absence or presence of increasing concentrations of the pure compounds 1 and 3 (12.5–500 μg/mL). After 24 h, MTT was added at a final concentration of 1.5 mg/mL. Plates were incubated for 2 h at 37 °C. The purple formazan crystals were dissolved by adding 150 μL of ethanol and the absorbance was read at 570 nm in a microplate reader. Results were calculated as the ratio between the optical density in the presence and absence of the compound multiplied by 100.
Statistical analysis
Results are presented as mean ± SEM. The statistical significance was determined by using one-way analysis of variance (ANOVA) employing the GraphPad Prism 5.0 software (GraphPad Software Inc., San Diego, CA). Comparisons were referred to the control group. p values of <0.05 were considered significant.
Results
Trypanocidal activity of plant extracts
All the tested dichloromethane extracts showed parasiticidal activity on T. cruzi epimastigotes at concentrations of 100 and 10 μg/mL. Stevia aristata and S. satureiifolia var. satureiifolia extracts induced growth inhibition percentages of 87.3 ± 0.3% and 90.9 ± 1.2% at a concentration of 10 μg/mL ().
Figure 1. Trypanocidal activity of organic extracts of Stevia aristata, Stevia aristata, Stevia satureifolia var. satureifolia, Stevia multiaristata against T. cruzi epimastigotes. Epimastigotes were cultured for 72 h at 28 °C in the presence of the extracts at concentrations of 100 and 10 μg/mL. Values represent mean ± SEM from three independent experiments carried out in triplicate.
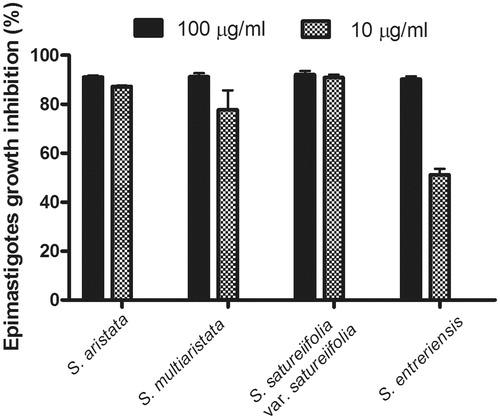
HPLC analysis of S. satureiifolia var. satureiifolia dichloromethane extract (DCME)
The DCME was analyzed by HPLC and three major compounds were detected: compounds 1 (rt: 14.4 min), 2 (rt: 15.5 min) and 3 (rt. 18.1 min) in order of elution (). The UV spectra indicated that these compounds had a flavonoid structure.
Bioassay-guided fractionation of S. satureiifolia var. satureiifolia
The DCME was fractionated using column chromatography. Seven fractions were obtained and assayed on T. cruzi epimastigotes. All the fractions (SS1–SS7) exerted significant trypanocidal activity with growth inhibition percentages that were higher than 90% at 100 μg/mL. At a concentration of 10 μg/mL, fractions SS1–SS5 were the most active ones with inhibition percentages that were higher than 80% (data not shown). Compound 3 was obtained as a yellow precipitate from fraction SS2. The purification of fraction SS5 by CC yielded an amorphous powder (compound 1).
Identification and quantification of compounds
Compound 3 was identified as 5-hydroxy-6,7,3′4′-tetramethoxyflavone (5-desmethylsinensetin). Amorphous powder. UV λmax (MeOH) nm: 338, 275, 250, 240. EIMS (70 eV), m/z (rel. int.): 358: [M]+ (100), 343 (54), 329 (19), 315 (12), 298 (3), 257 (1), 181 (8), 167 (2), 153 (16), 149 (3). 1H-NMR (600 MHz, CDCl3): δ 3.96 (3H, s), δ 4.00 (3H, s), δ 4.01 (3H, s), δ 4.02 (3H, s), δ 6.58 (1H, s, H-3), δ 6.63 (1H, s, H-8), δ 7.01 (1H, d, J = 8.6 Hz, H-5′), δ 7.37 (1H, d, J = 2.1 Hz, H-2′), δ 7.56 (1H, dd, J = 2.0 and 8.5 Hz, H-6′), 12,78 (1H, s). These data are in accordance with those reported in the literature (González et al. Citation1978, Citation1988).
Compound 1 was identified as 5,3′-dihydroxy 6,7,4′-trimethoxyflavone (eupatorin) by HPLC employing a commercial standard. Compound 2, which had been detected in the DCME, was identified as cirsimaritin by HPLC employing a commercial standard. The structures of eupatorin, cirsimaritin and 5-desmethylsinensetin are shown in . The content of these flavonoids was 1.3, 1.9 and 1.5% in the DCME, and 0.047, 0.068 and 0.054%, respectively, calculated on dry plant material.
Antiprotozoal activity of compounds
The effect of eupatorin and 5-desmethylsinensetin on T. cruzi epimastigotes is shown in . IC50 values, calculated for both flavonoids, were 0.2 and 0.4 μg/mL, respectively. IC50 values for both compounds on T. cruzi trypomastigotes were 75.1 μg/mL (5-desmethylsinensetin) and 61.8 μg/mL (eupatorin) (). On one hand, the flavone 5-desmethylsinensetin showed moderate activity on amastigotes, with an IC50 value of 78.7 μg/mL. On the other hand, eupatorin was not active against this parasite stage (). The flavones, 5-desmethylsinensetin and eupatorin, showed activity against L. braziliensis promastigotes (IC50 value= 37.0 and 55.1 μg/mL, respectively) (). The reference drug benznidazole showed IC50 values of 1.3, 9.5 and 0.98 μg/mL against epimastigotes, trypomastigotes and amastigotes, respectively. Amphotericin B had an IC50 value of 0.12 μg/mL for L. braziliensis promastigotes.
Figure 3. Effect of eupatorin and 5-desmethylsinensetin on T. cruzi epimastigotes. Parasites were cultured for 72 h at 28 °C in the presence of final compound concentrations ranging from 0.1 to 50 μg/mL. Results are expressed as mean ± SEM.
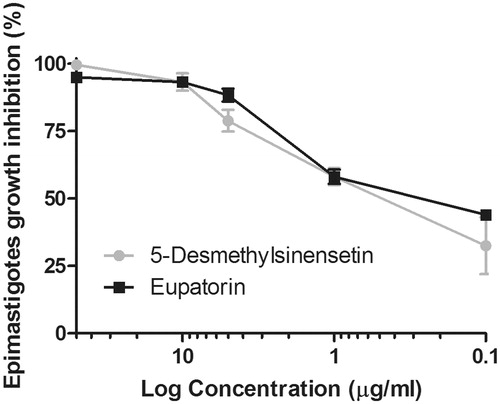
Figure 4. Trypanocidal activity of eupatorin and 5-desmethylsinensetin on T. cruzi trypomastigotes. Parasites were seeded in a 96-well microplate over 24 h at 4 °C in the presence of the compounds (0–100 μg/mL), and the remaining live parasites were counted in a Neubauer chamber. Results are expressed as mean ± SEM.
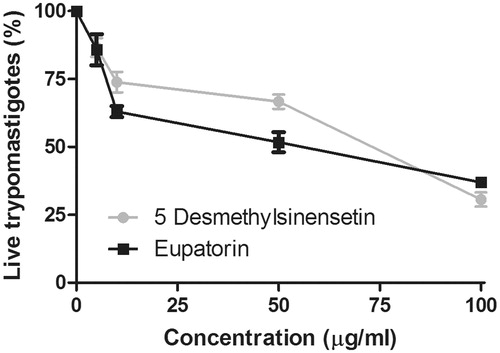
Figure 5. Trypanocidal activity of eupatorin and 5-desmethylsinensetin on T. cruzi amastigotes. Vero cells infected with transfected bloodstream trypomastigotes expressing β-galactosidase were incubated for 5 d in the presence of the compounds (0–100 μg/mL). The presence of amastigotes was developed later by adding chlorophenolred-β-d-galactopyranoside in the presence of Nonidet P40 and incubated for 4 h. The absorbance was measured at 595 nm. Results are expressed as mean ± SEM.
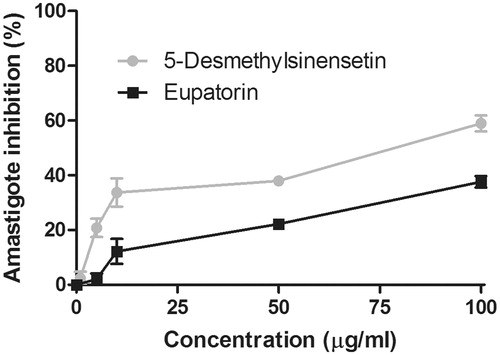
Cytotoxicity
Eupatorin and 5-desmethylsinensetin showed no cytotoxicity on Vero cells up to a concentration of 500 μg/mL ().
Table 1. Vero cells viability after treatment with eupatorin and 5-desmethylsinensetin.
Discussion
In the search for new natural antiprotozoal compounds, four native Stevia species were evaluated for their activity on T. cruzi epimastigotes. All the tested dichloromethane extracts showed parasiticidal activity. Stevia satureifolia var. satureifolia dichloromethane extract was selected for bioassay-guided fractionation in order to isolate its bioactive compounds. Three 6-methoxy flavones, eupatorin, cirsimaritin and 5-desmethylsinensetin, were identified in this extract, being cirsimaritin the major compound, as determined by HPLC. Eupatorin and cirsimaritin have been previously reported in S. satureiifolia (Sosa et al. Citation1984). However, this is the first report on the presence of 5-desmethylsinensetin in this plant species.
Eupatorin and 5-desmethylsinensetin were isolated by bioassay-guided fractionation and tested for their trypanocidal and leishmanicidal activities. Cirsimaritin was not included in this study since it has been previously reported to be active against T. cruzi and Leishmania sp. with moderate cytotoxicity on Vero cells (Tasdemir et al. Citation2006). Trypanosoma cruzi epimastigotes and trypomastigotes proved to be susceptible to both eupatorin and 5-desmethylsinensetin. When these compounds were assayed against the intracellular stage of the parasites in mammalian cells, only 5-desmethylsinensetin was moderately active. This compound was also the most active against L. braziliensis promastigotes. Nevertheless, the isolated compounds were less active than the reference drugs.
In order to determine the selectivity of the isolated compounds, the cytotoxicity of eupatorin and 5-desmethylsinensetin on Vero cells was evaluated. Neither of the flavonoids showed cytotoxicity, indicating that these compounds have the capacity to inhibit the parasite growth without displaying a significant toxicity on the host's cell.
According to the literature, 6-methoxyflavones have been reported as having antiprotozoal activity (Schmidt et al. Citation2012). In this sense, we have previously found that hispidulin and santin were active in vitro against T. cruzi epimastigotes and trypomastigotes as well as on L. mexicana promastigotes, with low cytotoxicity against murine T cells (Sülsen et al. Citation2007). Our present findings support the importance of these kinds of flavonoids as trypanocidal and leishmanicidal leads.
Conclusion
The antiprotozoal activity of 5-desmethylsinensetin and eupatorin, together with their low toxicity on Vero cells, makes them attractive leads for the discovery of new drugs against T. cruzi and Leishmania spp.
Funding information
This research was supported in part by PIP [01540, and 0935] (Consejo Nacional de Investigaciones Científicas y Técnicas) and UBACYT [20020090200478, 20020100100201 and 20020130200270] (Universidad de Buenos Aires).
Acknowledgements
The authors wish to thank Mrs. Cristina Aguilera and Mrs. Teresa Fogal for their valuable technical assistance. This investigation is part of the activities within the Research Network Natural Products against Neglected Diseases (ResNet NPND): http://www.resnetnpnd.org/.
Disclosure statement
The authors report that they have no conflicts of interest. The authors alone are responsible for the content and writing of this article.
References
- Cerda-GarcíaRojas CM, Pereda-Miranda R. 2002. The phytochemistry of Stevia: a general survey. In: Kinghorn D, editor. Stevia: the genus Stevia. New York: Taylor and Francis. p. 86–118.
- Chiaramonte MG, Frank FM, Furer GM, Taranto NJ, Margni RA, Malchiodi EL. 1999. Polymerase chain reaction reveals Trypanosoma cruzi infection suspected by serology in cutaneous and mucocutaneous leishmaniasis patients. Acta Trop. 72:295–308.
- Drugs for Neglected Disease Initiative (DNDi). 2015a. About Chagas Disease; [cited 2016 Feb 16]. Available from: http://www.dndi.org/diseases-projects/chagas/.
- Drugs for Neglected Disease Initiative (DNDi). 2015b. About Leishmaniasis; [cited 2016 Feb 16]. Available from: http://www.dndi.org/diseases-projects/leishmaniasis/.
- Franco-Paredes C, Bottazzi ME, Hotez PJ. 2009. The unfinished public health agenda of Chagas disease in the era of globalization. PLoS Negl Trop Dis. 3:e470.
- Frank FM, Fernandez MM, Taranto NJ, Cajal SP, Margni RA, Castro E, Thomaz-Soccol V, Malchiodi EL. 2003. Characterization of human infection by Leishmania spp. in the northwest of Argentina: immune response, double infection with Trypanosoma cruzi and species of Leishmania involved. Parasitology 126:31–39.
- González AG, Fraga BM, Hernández MG, Larruga F, Luis JG, Ravelo AG. 1978. Flavones from some Canary species of Sideritis. Lloydia. 41:279–280.
- González AG, Herrera JR, Luis JG, Ravelo AG, Ferro EA. 1988. Terpenes and flavones of Salvia cardiophylla. Phytochemistry. 27:1540–1541.
- Gould IT, Perner MS, Santini MS, Saavedra SB, Bezzi G, Maglianese MI, Antman JG, Gutiérrez JA, Salomón OD. 2013. Leishmaniasis visceral en la Argentina. Notificación y situación vectorial (2006–2012). Medicina. 73:104–110.
- Kinghorn D. 2002. Overview. In: Kinghorn D, editor. Stevia: the genus Stevia. New York: Taylor and Francis. p. 1–17.
- Mabry TJ, Markham KR, Thomas MB. 1970. The systematic identification of flavonoids. New York, USA: Springer-Verlag.
- Rassi AJr, Rassi A, Marin-Neto JA. 2010. Chagas disease. Lancet. 375:1388–1402.
- Rodriguez Coura J, Albajar Viñas P. 2010. Chagas disease: a new worldwide challenge. Nature. 465:S6–S7.
- Schmidt TJ, Khalid SA, Romanha AJ, Alves TM, Biavatti MW, Brun R, Da Costa FB, de Castro SL, Ferreira VF, de Lacerda MV, et al. 2012. The potential of secondary metabolites from plants as drugs or leads against protozoan neglected diseases – Part II. Curr Med Chem. 19:2176–2228.
- Sosa VE, Oberti JC, Prasad JS, Herz W. 1984. Flavonoids and eupahakonenin B from Stevia satureiaefolia. Phytochemistry. 23:1515–1516.
- Sülsen V, Cazorla S, Frank F, Redko FC, Anesini CA, Coussio JD, Malchiodi EL, Martino VS, Muschietti LV. 2007. Trypanocidal and leishmanicidal activities of flavonoids from Argentine medicinal plants. Am J Trop Med Hyg. 77:654–659.
- Sülsen VP, Frank FM, Cazorla SI, Anesini CA, Malchiodi EL, Freixa B, Vila R, Muschietti LV, Martino VS. 2008. Trypanocidal and leishmanicidal activities of sesquiterpene lactones from Ambrosia tenuifolia Sprengel (Asteraceae). Antimicrob Agents Chemother. 52:2415–2419.
- Sülsen VP, Frank FM, Cazorla SI, Barrera P, Freixa B, Vila R, Sosa MA, Malchiodi EL, Muschietti LV, Martino VS. 2011. Psilostachyin C: a natural compound with trypanocidal activity. Int J Antimicrob Agents. 37:536–543.
- Sülsen VP, Cazorla SI, Frank FM, Laurella LC, Muschietti LV, Catalán CA, Martino VS, Malchiodi EL. 2013. Natural terpenoids from Ambrosia species are active in vitro and in vivo against human pathogenic trypanosomatids. PLoS Negl Trop Dis. 7:e2494.
- Tasdemir D, Kaiser M, Brun R, Yardley V, Schmidt TJ, Tosun F, Rüedi P. 2006. Antitrypanosomal and antileishmanial activities of flavonoids and their analogues: in vitro, in vivo, structure–activity relationship and quantitative structure-activity relationship studies. Antimicrob Agents Chemother. 50:1352–1364.
- World Health Organization (WHO). 2015. Chagas disease (American trypanosomiasis) Fact sheet N°340; [cited 2015 Mar 26]. Available from: http://www.who.int/mediacentre/factsheets/fs340/es/.
- Zdero C, Bohlmann F, Schmeda-Hirschmann G. 1987. Beyerene derivatives and other terpenoids from Stevia aristata. Phytochemistry. 26:463–466.
- Zuloaga F, Morrone O, Belgrano M. 2008. Catálogo de las Plantas Vasculares del Cono Sur (Argentina, Sur de Brasil, Chile, Paraguay y Uruguay). Vol. 2 Dicotyledoneae: Acanthaceae-Fabaceae (Abarema-Schizolobium). St. Louis, MO: Missouri Botanical Garden Press.