Abstract
Context Seaweeds from the Mexican Pacific Ocean have not been evaluated as a source of chemoprotectants.
Objective The objective of this study is to evaluate chemopreventive activities of the seaweeds Phaephyceae – Padina durvillaei (Dictyotaceae) – Rodhophyceae – Spyridia filamentosa (Spyridiaceae), Gracilaria vermiculophylla (Gracilariaceae) – and Chlorophyceae – Ulva expansa (Ulvaceae), Codium isabelae (Codiaceae), Rhizoclonium riparium (Cladophoraceae) and Caulerpa sertularioides (Caulerpaceae).
Materials and methods Methanol, acetone and hexane seaweed extracts were assessed at 30 and 3 mg/mL on antioxidant capacity (DPPH and ABTS assays), 0.003–3.0 mg/plate on antimutagenic activity against AFB1 using Salmonella typhimurium TA98 and TA100 tester strains in Ames test, and 12.5 to 100 μg/mL on antiproliferative activity on Murine B-cell lymphoma. Phenols, flavonoids and pigments content were also assessed as antioxidant compounds.
Results Extraction yield was higher in methanol than in acetone and hexane extracts (6.4, 2.7 and 1.4% dw). Antioxidant capacity was higher in brown and green than in red seaweed species, particularly in P. durvillaei extracted in acetone (EC50 value= 16.9 and 1.56 mg/mL for DPPH and ABTS). Flavonoids and chlorophylls were identified as mainly antioxidant components; particularly in hexane extracts, which were correlated with the antioxidant capacity. Highest mutagenesis inhibition (> 40%) occurred in R. riparium at the lowest concentration assayed (0.003 mg/plate), while highest antiproliferative inhibition (37 and 72% for 12.5 and 25 μg/mL) occurred in C. sertularioides.
Discussion and conclusion Flavonoids and chlorophylls explained the chemopreventive activities assessed in S. filamentosa, R. riparium and C. sertularioides. These seaweeds have a high potential as a source of novel chemoprotectants.
Introduction
Seaweeds have ancestrally been used as food for human consumption, but seaweeds are also an important source of thermo-polysaccharides or phycocolloids (i.e., agar, carrageenan and alginates) that are extensively used due to their gelling, stabilizing and emulsifying properties in several food and pharmaceutical industries (Laurienzo Citation2010; Shalaby Citation2011; Stengel et al. Citation2011; Mohamed et al. Citation2012). More recently, seaweeds have been widely studied as a good source of several therapeutic biocompounds such as sulphated polysaccharides (i.e., ulvans, fucoidans and laminarans), pigments (i.e., chlorophylls and carotenoids), lipids (i.e., fatty acids and phytosterols), phenolic compounds, terpenes, flavonoids, etc., that have been demonstrated to have an extensive number of chemopreventive properties (Michalak & Chojnacka Citation2015). These secondary bioactive metabolites have demonstrated not only notable capacity of brown and red seaweeds on antioxidant (Zaragozá et al. Citation2008; Souza et al. Citation2011; Peinado et al. Citation2014) and antimutagenic activity (Okai et al. Citation1996) but also the main three major division (i.e., brown, red and green) of seaweeds have been showed antiproliferative activity against several cancer cell lines (Yuan & Walsh Citation2006; Paul & Kundu Citation2013; Murugan & Iyer Citation2014).
In Mexico, although approximately 1600 seaweed species have been identified (Llorente-Bousquets & Ocegueda Citation2008), just a few species from the Pacific Ocean and Gulf of California have been studied and exploited as raw materials for the phycocolloid industry (Zertuche-González Citation1993). In contrast, the chemoprotective properties including the antioxidant (Zubia et al. Citation2007), cytotoxic and antiproliferative activities (Moo-Puc et al. Citation2009, Citation2011a,Citationb; Caamal-Fuentes et al. Citation2014a,Citationb) have been exclusively assessed in several species of seaweeds from the Gulf of Mexico. Some of the analysed genera include species of the three major divisions, i.e., Padina spp. (brown), Gracilaria spp. (red) and Caulerpa and Codium spp. (green), with higher chemopreventive activity in Chlorophyta and Rhodophyta in comparison with Phaeophyta species (Zubia et al. Citation2007). Therefore, we hypothesize that seaweed species from the same genera distributed in the Mexican Pacific Ocean may contain at least one chemopreventive compound of interest for the pharmaceutical industry.
Thus, to determine the potential use of seaweeds from the Mexican Pacific Ocean as a source of chemopreventive compounds, the aim of the present study was to assess the antioxidant, antimutagenic and antiproliferative activities of hexane (H), acetone (A) and methanol (M) extracts of seven seaweeds belonging to the following phyla: Phaephyceae (brown) – Padina durvillaei Bory Saint-Vincent (Dictyotaceae) – Rodhophyceae (red) – Spyridia filamentosa (Wulfen) Harvey (Spyridiaceae), Gracilaria vermiculophylla (Ohmi) Papenfuss (Gracilariaceae) – and Chlorophyceae (green) – Ulva expansa (Setchell) Setchell & N. L. Gardner (Ulvaceae), Codium isabelae W. R. Taylor (Codiaceae), Rhizoclonium riparium (Roth) Harvey (Cladophoraceae) and Caulerpa sertularioides (S. G. Gmelin) M. A. Howe (Caulerpaceae) – collected from the lagoons of Sinaloa, Mexico. This study increases the general knowledge of the chemopreventive compounds of seaweeds, particularly for those species distributed in the NW of Mexico.
Materials and methods
Sampling
All the seaweed specimens were collected from different lagoons along the coast of Sinaloa, Mexico. Rhizoclonium riparium (Roth) Harvey were collected during June 2013 at Urias lagoon (23°19′0.96′′N, 106°18′46.5′′W); Spyridia filamentosa (Wülfen) Harvey and Caulerpa sertularioides (S. Gmelin) M. Howe were collected during October 2013 at Agiabampo-Bacorehuis lagoon (26°20′37.2′′N, 109°14′34.6′′W and 26°20′26′′N, 109°12′50′′W, respectively), Gracilaria vermiculophylla (Ohmi) Papenfuss was collected during October 2013 at Santa Maria-La Reforma lagoon (29°50′14.7′′N, 108°05′24′′W); Codium isabelae W. R. Taylor, Padina durvillaei Bory Saint-Vicent and Ulva expansa (Setchell) Setchell and Gardner were collected during May 2014 at Mazatlan Bay (23°1′ 29.1′′N, 106°25′29.7′′W). All the seaweeds were identified according to Ochoa-Izaguirre et al. (Citation2007) and voucher specimens were deposited at the Facultad de Ciencias del Mar Marine Algae Herbarium. At the laboratory, each specimen was washed in situ with seawater to remove epibionts as well as the necrotic parts of the plants. Algal samples were transported to the laboratory in plastic bags covered with ice. The subsamples of each collected specimen were frozen and stored at −70 °C until further analysis. Fresh samples were rinsed with distilled water, lyophilized (FreeZonePlus 4.5 Labconco, Kansas City, MO) and ground with a commercial grinder.
Seaweed extracts
Crude extracts were obtained using different solvents according to the method described by Rosaline et al. (Citation2012) with the following modifications: the powdered samples were soaked with hexane (1:3 w/v) at 21 °C for 48 h and filtered through Whatman® GF/C filter paper under vacuum; the filtrate was concentrated under negative pressure at 50 °C using a rotary vacuum evaporator (Yamato RE601 Scientific Co., LTd. Tokyo, Japan), and finally evaporated to dryness under N2 stream. The procedure was repeated sequentially using acetone and methanol in a similar manner to cold percolation method. The crude extracts were stored at −20 °C until analyzed. All the analyses were performed in triplicate.
Determination of total phenolic compound content (TPCC)
The total soluble phenolic compound content was determined using the Folin–Ciocalteu method (Marigo Citation1973). A 100 μL aliquot of each seaweed extract was combined with 150 μL of Folin solution (diluted 1:1 with deionized water) (Sigma Chemical Co., St. Louis, MO). Then, 1 mL of a 2% sodium carbonate in 0.4% sodium hydroxide solution was added to the mixture. The mixture was allowed to incubate at room temperature for 20 min in the dark. The resulting blue complex was spectrophotometrically measured at 750 nm. The phenolic content was expressed as mg acid gallic equivalent (GAE) per 100 mg of extract.
Determination of total flavonoid content
The total flavonoid content was assessed colorimetrically according to Luximon-Ramma et al. (Citation2002). Seaweed extracts (1 mL) were diluted in equal volumes of a solution of 2% aluminium chloride (2 g of AlCl3.6H2O in 100 mL of methanol, both Sigma Chemical Co., St. Louis, MO). The mixture was incubated at room temperature for 10 min. The absorbance was then assessed at 367 nm using a spectrophotometer (GENESYS 10S, Thermo Fisher Scientific, Waltham, MA). Results were expressed in mg of quercetin (QE) equivalents per gram dry weight of sample.
Determination of total chlorophylls and carotenoids content (TCC)
The total chlorophyll and carotenoid contents were assessed colorimetrically according to Wellburn (Citation1994) using the equations described below. The extracts were diluted with pure acetone until the desired concentrations (0.1–2.7 mg/mL) were reached. A known volume of extract was diluted with pure acetone, and the A470, A646 and A663, values were obtained using an 80% acetone solution as a blank. The concentration values were referred to the weight of a known quantity of dry extract
where Ca= chlorophyll a; Cb = chlorophyll b; Cx + C = total carotenoids.
Antioxidant test
DPPH radical scavenging assay
The free radical-scavenging potential of the seaweed extracts were analyzed according to the method described by Müller et al. (Citation2011) with the following modifications: a 100 μL aliquot of each extract (at a concentration of 30 mg/mL) was combined with 900 μL of a 0.3 mM 2,2-diphenyl-1-picrylhydrazyl (DPPH) radical solution in hexane:methanol (1:1); the mixture was incubated for 30 min in the dark at room temperature; the A540 nm was measured with a UV–Vis spectrophotometer (GENESYS 10S, Thermo Fisher Scientific, Waltham, MA); l-ascorbic acid (Sigma Chemical Co., St. Louis, MO) was used as a reference standard, whereas DPPH solution served as a control to calculate the extent of radical scavenging by both the extracts and the reference compound.
The percentage of DPPH radical scavenging was calculated according to the following equation:
The mean effective concentration (EC50) was calculated as the concentration of sample that reduces 50% of the DPPH radical under the assayed conditions (Chang et al. Citation2007). The total antioxidant activity was expressed as the vitamin C equivalent antioxidant capacity (VCEAC), or mg VCE per 100 mg of dry extract.
ABTS radical scavenging assay
The 2,2′-azinobis [3-ethylbenzthiazoline]-6-sulphonic acid (ABTS) radical scavenging activity was determined according to the method described by (Przygodzka et al. Citation2014) with the following modifications: the ABTS radical was previously activated for 12–16 h at room temperature in the dark; the resultant ABTS•+ radical solution was diluted with ethanol to obtain an A734 nm value of approximately 0.70; a 100 μL aliquot of each seaweed extract (at a concentration of 3 mg/mL) was combined with 2.9 mL of ABTS solution and the absorbance was spectrophotometrically (GENESYS 10S, Thermo Fisher Scientific, Waltham, MA) measured 10 min after mixing.
The percentage of ABTS radical scavenging was calculated using the following equation:
where A = A734 nm of ABTS•+ radical at time cero (without sample); B = A734 nm of ABTS•+ radical with sample after 10 min.
The mean effective concentration (EC50) was calculated as the concentration of sample that reduced 50% of the ABTS•+ radical under the assayed conditions. Vitamin C and Trolox (Sigma Chemical Co., St. Louis, MO) were used as a control.
Antimutagenic activity
Bacteria cultures
Salmonella typhimurium TA98 and TA100 tester strains (purchased from Molecular Toxicology Inc., Annapolis, MD) were routinely checked to confirm genetic features using the procedure described by Maron and Ames (Citation1983). A metabolic activation system S9 mix (Aroclor 1254-induced, Sprague–Dawley male rat liver in 0.154 M KCl solution) was also purchased from Molecular Toxicology, Inc. (Boone, NC).
Antimutagenicity assay
The seaweed extracts were reconstituted and serially diluted with DMSO or acetone, according to the extract polarity and were spiked with pure aflatoxin B1 (AFB1) (Sigma-Aldrich, St. Louis, MO). Residual AFB1 mutagenicity was determined using the standard plate incorporation procedure described by Maron and Ames (Citation1983), and 500 ng of aflatoxin B1 per plate was used as a control for both tester strains. Each assay was performed in triplicate, and the antimutagenicity was expressed as the percentage of AFB1 mutagenicity inhibition:
where A = the number of His + revertants in the presence of seaweed extract samples; B = the number of His + revertants in the absence of seaweed extract samples (positive control); C = spontaneous revertants.
Antiproliferative activity
Cell lines
The cell line M12.C3.F6 (murine B-cell lymphoma) was kindly provided by Dr. Emil R. Unanue (Department of Pathology and Immunology, Washington University at St. Louis, MO). The cells were cultured in Dulbecco’s modified Eagle’s medium (DMEM) (Gibco, Grand Island, NY) supplemented with 5% heat inactivated foetal calf serum and grown at 37 °C in an atmosphere of 5% CO2.
Antiproliferation assay
The effect of the seaweed extracts on the proliferation of the M12.C3F6 cell line was determined using the standard MTT assay (Mosmann Citation1983). Briefly, 10 000 cells (50 μL) were placed in each well of a flat 96-well plate. After a 12 h incubation at 37 °C in an atmosphere of 5% CO2 to allow cell attachment, the cell cultures were incubated with 100 μL of medium containing various concentrations of the seaweed extracts and incubated for 48 h. All the extracts were first re-suspended in DMSO and diluted in supplemented DMEM media. The control cell cultures were incubated with DMSO (final concentrations of DMSO 0.06–0.5% v/v). The control cell cultures did not show any evidence of cell damage. Prior to the last 4 h of the cell culture, 10 μL of MTT stock solution (5 mg/mL) were added to each well.
The formed formazan crystals were dissolved with acid isopropanol (HCl 0.04 N at 1%), and the plates were read at a test wavelength of 570 nm and a reference wavelength of 630 nm in an ELISA plate reader (Benchmark Microplate Reader; Bio-Rad, Hercules, CA). The plates were typically read within 15 min after the addition of isopropanol.
Statistical analysis
One-way ANOVA was performed to analyze the yield extraction assessed with three different organic solvents, total phenol content, total flavonoid content, chlorophyll and carotenoid content, as well antioxidant, antimutagenic and antiproliferative activities of each extract obtained from the selected seaweeds, followed by a post hoc Tukey’s test to assess significant differences between the means. The percentage results were transformed to arcsine before analyses (Zar Citation1999), but only the untransformed means are given. Factor analysis (Varimax normalized using principal components extraction) was used to extract the maximum variance from data sets (total phenolic compounds, total flavonoid content, chlorophyll and carotenoid content) with each principal component as a liner combination of antioxidant components of seaweed extracts, considering factors loadings ≥ 0.7 and eigenvalues >1.0. In order to analyse significant variation of each PC between seaweed species extracted with each organic solvent, we used them as new variables in one-way ANOVA followed by Tukey post hoc testing (Hurtado et al. Citation2012). Finally, these new variables that combine the antioxidant components analyzed were correlated (Pearson correlation) with radical scavenging activity of DPPH and ABTS. The data are reported as the mean ± standard error and were analyzed with Statistica v. 6.0 (Statsoft, Inc., Tulsa, OK).
Results
Extraction yield
Significant differences (p < 0.05) were observed among the extraction yields of the selected seaweeds extracted with hexane, acetone and methanol (). The highest values were obtained for R. riparium, S. filamentosa and C. sertularioides extracted with methanol (9.5, 8.3 and 6.7%), and the remaining seaweed species extracted with methanol showed relatively high values (4.2–5.8%). Contrary to most of the seaweed species tested, a significant high value (6.7%) was obtained for P. durvillaei extracted with acetone. The yield extraction values of the selected seaweeds assessed with acetone, hexane and methanol averaged 6.4 ± 0.5, 2.7 ± 0.5 and 1.4 ± 0.3%, respectively. The principal components obtained from data set of antioxidant compounds (see below) were not correlated with extraction yield assessed with hexane and methanol, while acetone extractions were positive and significant correlated (r = 0.75, p < 0.05, ) with the PC2 () that explained 31.4% of the variance and had significant contribution of total phenolic compounds and total carotenoids content ().
Figure 1. Extraction yield (%) of selected seaweed species assessed with three different organic solvents. The data are expressed as the mean ± standard error and were analyzed by unifactorial ANOVA followed by a Tukey test to assess significant differences. Means not sharing the same superscripts are significantly different (p < 0.05). n = 3 samples for treatment. Hexane, acetone and methanolic extractions are represented by black, grey and white bars, respectively.
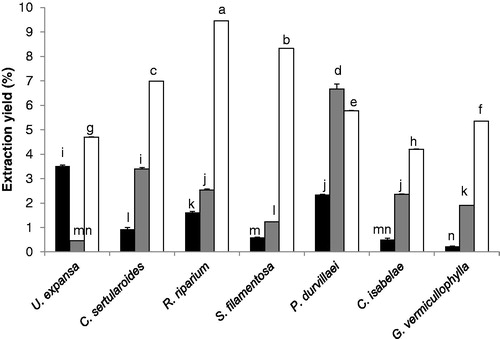
Figure 2. (a) PC1 and (b) PC2 obtained from total antioxidant compounds (flavonoids, total phenolic compounds, total chlorophylls content, chlorophyll a and b, and total carotenoids content) and antioxidant activity assessed with (b) DPPH and (c) ABTS in selected seaweeds extracted in hexane, acetone and methanol. The data are expressed as the mean ± standard error. See for statistical analysis. DPPH are expressed in vitamin C equivalents of antioxidant capacity (VCEAC) and ABTS in mg of VCE per 100 mg of dry extract (n = 3).
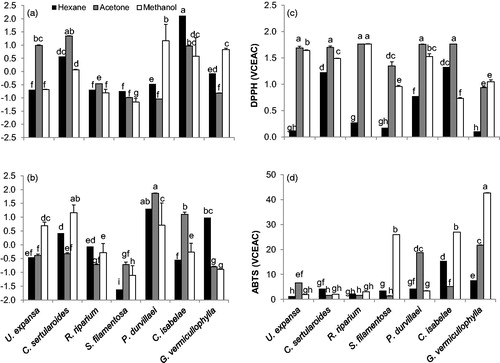
Table 1. Factor loadings (Varimax normalized) using principal components extraction and correlation of PC with antioxidant capacity of seaweed extracted in hexane, acetone and methanol.
Antioxidant activity
Total phenolic compounds, total flavonoid content, chlorophylls and carotenoid content
The total phenolic compounds, total flavonoid content, total chlorophylls, chlorophyll a and b and total carotenoids content showed significant (p < 0.05) differences among treatments and species, the individual values of all these variables are showed in . Significant differences were found in the two principal components (PC1 and PC2, ) obtained from the linear combination of data set of all antioxidant compounds mentioned above. The first two components derived from seaweeds extracted in hexane explained 57.5% and 21.6% of the variance and had significant contribution of total flavonoids (0.86), total chlorophylls content and chlorophylls a and b content (0.98, 0.92 and 0.91) for the PC1, while the PC2 had a significant but negative contribution of total phenolic compounds (−0.80) and total carotenoids content (0.73, ). The first two components derived from seaweeds extracted in acetone explained 59.7 and 31.3% of the variance and had significant contribution of total flavonoids (0.84) and total chlorophylls content and chlorophylls a and b content (0.99, 0.96 and 0.94) for the PC1, while the PC2 had a significant contribution of total phenolic compounds (0.92) and total carotenoid content (0.96, ). Three principal components were obtained in seaweed extracted in methanol, which explained 52.4, 19.8 and 16.7% of the total variance (). The PC1 had a significant contribution of total chlorophylls and chlorophyll a and b content (0.98, 0.94 and 0.97), the PC2 had a significant contribution of total flavonoids (0.88) and the PC3 had a significant but negative contribution of total phenolic compounds (−0.99).
Table 2. Total phenolic content (TPC), flavonoid content (FC), total chlorophylls and carotenoid content (TCC: Ct, total chlorophyll; Ca, chlorophyll a; Cb, chlorophyll b; and Cx + C, total carotenoids) in hexane (H), acetone (A) and methanol (M) extracts of selected seaweeds.
DPPH and ABTS radical scavenging activity
Significant differences were observed in DPPH and ABTS radical scavenging activity among the selected seaweed species (). The highest values of DPPH radical scavenging activity was obtained for R. riparium, C. isabelae, P. durvillaei and C. sertularioides, all extracted with acetone, as well as for R. riparium extracted in methanol (1.74% average). In general, the higher DPPH radical scavenging activity values were obtained from the seaweeds extracted with acetone, followed by those obtained with methanol, and the lowest were obtained for those seaweed species extracted with hexane (1.56, 1.31 and 0.57%, respectively). The lowest seaweed extract concentrations to achieve a 50% reduction of DPPH radical was observed for P. durvillaei and U. expansa both extracted with acetone (17 and 34 mg/mL, respectively), followed of P. durvillaei and R. riparium extracted with methanol and S. filamentosa extracted with acetone (). In hexane seaweeds extracts, but not in acetone or methanol, antioxidant activity assessed with DPPH was positive and significantly correlated (r = 0.81, p < 0.05, ) with the PC1 () that explained 57.5% of the variance with significant contribution of total flavonoids, total chlorophyll content and chlorophyll a and b content ().
Figure 3. Antioxidant activity of selected seaweed extracts and controls (vitamin C and Trolox) expressed as the effective concentration (EC50 mg/mL) to assess 50% of the radical scavenging activity of (a) DPPH and (b) ABTS. The data are expressed as the mean ± standard error. See for statistical analysis. A = acetone, M = methanol.
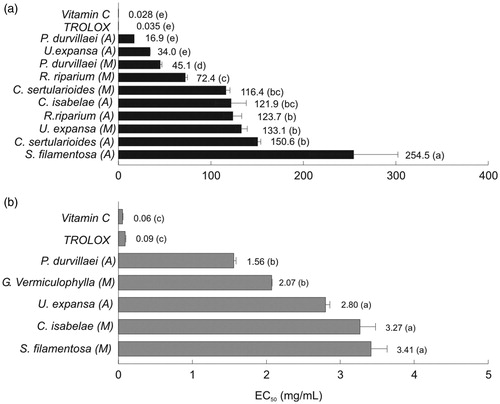
In contrast, the highest values of ABTS were obtained for G. vermiculophylla, C. isabelae and S. filamentosa extracted with methanol (42.7, 26.9 and 25.9%, respectively), followed of G. vermiculophylla and P. durvillaei extracted with acetone (21.8 and 18.7%, respectively) and C. isabelae extracted with hexane (15.3%). The remaining seaweed extracts showed lower activity (< 7.6%, ). The concentration of the seaweed extracts to achieve 50% reduced ABTS radical was the lowest for P. durvillaei and G. vermiculophylla extracted with acetone and methanol (1.56 and 2.07 mg/mL, respectively), followed by U. expansa, C. isabelae and S. filamentosa extracted with acetone and methanol, respectively (). In hexane extracts, antioxidant capacity assessed with ABTS was positive and significantly correlated (r = 0.91, p < 0.01, ) with the first component () that explained 57.5% of the variance with significant contribution of total flavonoids content, total chlorophylls content and chlorophyll a and b (). No significant correlations were observed between ABTS and principal components obtained in acetone and methanol extracts ().
Antimutagenic activity
The highest inhibition (≥ 95%) of aflatoxin B1 (AFB1) mutagenicity on S. typhimurium TA98 and TA100 tester strains was obtained for C. sertularioides, R. riparium and S. filamentosa extracted with acetone at both concentrations assayed (3 and 0.3 mg/plate, ). Inhibition of AFB1 mutagenicity on both tester strains decreased as the seaweed extract concentration decreased, particularly in C. sertularioides and S. filamentosa; however, in R. riparium mutagenicity, the inhibition values were higher (54 and 78%) in both tester strains at the lowest concentration assayed (0.003 mg/plate, )
Figure 4. Antimutagenic activity (% inhibition) of acetone extracts of C. sertularioides, R. riparium and S. filamentosa assessed at different concentrations (0.003–3.0 mg/plate) on (a) S. typhimurium TA98 and (b) TA100. Mutagen aflatoxin B1 (500 ng/plate) was used as a positive control and spontaneous revertants in S. typhimurium culture were metabolically activated in all the treatments. The data are expressed as the means ± standard error (n = 3). Activity reference values (% of reversion inhibition > 40 = strong-, 25–40 = moderate- and <25 = weak antimutagenic activity) were used according to Ikken et al. (Citation1999).
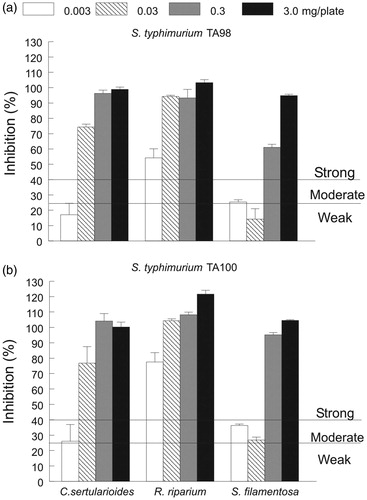
Table 3. Antimutagenic activity of seaweed extracts against aflatoxin B1mutagen on S. typhimurium TA98 and S. typhimurium TA100.
According to the classification proposed by Ikken et al. (Citation1999) for terrestrial vegetables, most of the seaweed extracts showed a strong antimutagenic activity, particularly R. riparium with both tester strains at all concentrations assayed. Additionally, C. sertularioides and S. filamentosa maintained strong antimutagenic activity at concentrations of 0.03–3.0 mg/plate and 0.3–3.0 mg/plate, respectively, but the activity decreased at the lowest concentrations ().
Antiproliferative activity
The seaweeds extracted with acetone triggered visible cellular damage (i.e., apoptotic bodies and stress shaped cells) in the M12.C3.F6 (murine B-cell lymphoma) cell line after 24 h of exposition. This damage was particularly evident for C. sertularioides, R. riparium and S. filamentosa (). Non-visible changes in the shape or cellular damages were observed in the cancerous cell line treated with the seaweeds extracted with hexane and methanol (). In comparison with the hexane- and methanol-extracts, the seaweeds extracted with acetone showed higher antiproliferative activity, particularly for C. sertularioides, R. riparium and S. filamentosa (). The antiproliferative activity decreased with an increase of the seaweed extract concentration; however, for S. filamentosa and R. riparium, the major effect on cell proliferation was observed at the highest concentration (25 and 29% for 100 μg/mL, respectively). Caulerpa sertularioides, at the lowest concentration, exhibited a significant decrease in cell proliferation (63 and 28% for 12.5 and 25 μg/mL, respectively) ().
Figure 5. Representative images (20×) for changes observed in cell morphology of M12.C3.F6 (murine B-cell lymphoma) after treatment with seaweed extracts (100 μg/mL) and concentration effect of acetone extract of C. sertularioides (CsA). Code images: XxY, where Xx = seaweeds species (Ue, U. expansa; Cs, C. sertularioides; Rr, R. riparium; Sf, S. filamentosa; Pd, P. durvillaei; Ci, C. isabelae; and Gv, G. vermiculophylla) and Y = type of extract (H, hexane; A, acetone; M, methanol) CU1 and CU2: Are untreated controls, with DMSO used in the screening and CsA treatment, respectively.
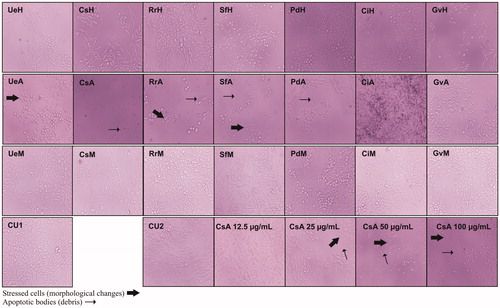
Figure 6. (a) Effect of the extraction solvent (i.e., hexane, white bars; acetone, grey bars or methanol, black bars) on the cell proliferation (%) of selected seaweeds and (b) concentration effect (12.5–100 μg/mL) of the acetone extract of S. filamentosa, R. riparium and C. sertularioides on cell proliferation (%). Control cell cultures were incubated with DMSO (0.5%), which represented 100% proliferation. The data are expressed as the means ± standard error. See for statistical analysis.
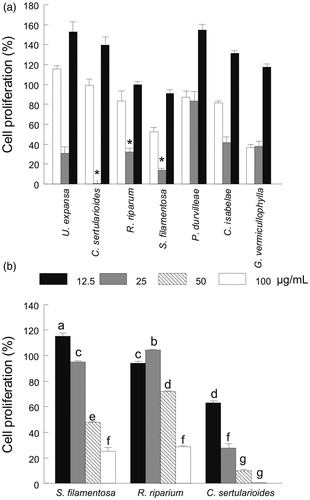
Discussion
In the present work, both the solvent extraction and seaweed species exerted a significant effect on the yield extraction, where higher values were obtained in most of the seaweed species extracted with methanol, followed by those extracted with acetone and hexane ().
According to the results derived from principal components analysis, yield extraction was not correlated with the content of antioxidant compounds (i.e., phenolic compounds, flavonoids content, total chlorophylls and chlorophyll a and b, and total carotenoids), excepting acetone extracts, that a positive correlation with the PC2 was obtained (), where the total phenolic compounds and total carotenoids content exerted a significant contribution (). The higher yield values observed in methanol seaweed extracts could be related to some other polar molecules as the cellular membrane lipids (i.e., glycolipids and phospholipids), that are characterized by their content of highly unsaturated fatty acids (i.e., eicosapentaenoic and docosahexaenoic fatty acids) in red and brown but not in green seaweeds, which are characterized by their higher content of saturated and monounsaturated fatty acids (Serviere-Zaragoza et al. Citation2015).
The lipophilic extracts from seaweed species are well known to be a potential source of lipophilic antioxidants, which have been demonstrated to increase in their antioxidative activity as the content of unsaturated fatty acids in the seaweed increases (Huang & Wang Citation2004). Additionally, the presence of other lipophilic compounds such as pigments, vitamins and/or phytosterols in the methanolic extracts from the selected seaweeds studied in the present work has not been discarded.
Antioxidant capacity depends of several issues that are mainly related to the assay conditions, the nature of the sample (i.e., animal or plant, terrestrial or aquatic, etc.) and the organic solvent used to perform the biological extractions to evaluate the redox ability to scavenge free radicals. The DPPH and ABTS methods significantly correlate when sorghum products (Awika et al. Citation2003) and several fruits, vegetables and beverages (Floegel et al. Citation2011) have been studied using both these techniques; however, DPPH and ABTS correlated in guava fruit extracted with methanol, but not when dichloromethane was used (Thaipong et al. Citation2006). In this work, the DPPH and ABTS methods did not correlate between the seaweed species extracted with any of the three solvents assayed (i.e., acetone, hexane and methanol); however, differences between both the antioxidant activity assays appeared to be related to the seaweed species and to a lesser extent the organic solvent used to perform the extraction. The antioxidant activity of crude extracts was expected to strongly depend on the type of solvent used because the compounds with different polarities, exhibiting different rates of antioxidant potential, are partitioned among the extracting solvents (Marinova & Yanishlieva Citation1997). In fruits and vegetables, vitamins and phenols are hydrophilic antioxidants, while antioxidant pigments are lipophilic (Halliwell Citation1996). In contrast, higher contents of phenols and flavonoids were extracted from selected seaweeds using chloroform and ethyl acetate than when water and methanol were employed, suggesting a higher affinity to lipophilic rather than hydrophilic antioxidants (Murugan & Iyer Citation2014).
In this work, the content of antioxidant compounds assessed on selected seaweed species extracted with hexane, acetone and methanol exerted a significant effect on antioxidant capacity assessed with DPPH and ABTS assays. According to principal component analysis, flavonoids and chlorophylls explained the main variation of antioxidant compounds analyzed on seaweeds extracted in hexane and acetone, while only chlorophylls on seaweeds extracted in methanol (). However, the PC1 that combines these antioxidant compounds was positive and significantly correlated with antioxidant capacity assessed with both DPPH and ABTS (), but only in seaweeds extracted in hexane (). This result suggested that organic solvent used to perform the extraction exerted a significant effect on stability of extracted compounds, or some other compounds contained at each solvent extraction are interacting or enhancing the antioxidant capacity, particularly in hexane seaweed extracts.
The antioxidant activity (as assessed using the DPPH and ABTS methods) of selected seaweeds extracts measured as the concentration to reduce 50% (EC50) of radicals was significantly different than the antioxidants used as controls (vitamin C and Trolox, ). In general, we observed higher antioxidant activity in the brown and green than in the red seaweeds, whose main source of variation was the content of flavonoids and chlorophylls and only chlorophylls for hexane and acetone and methanol seaweed extracts, respectively (). A similar tendency was reported for several green, brown and red seaweeds (1.44–51.48, 0.32–34.88 and 2.48–77.71 mg/mL, respectively) from the Gulf of Mexico that were extracted with dichloromethanol (Zubia et al. Citation2007). Although the differences between our results and those mentioned above could be attributed to the seaweed species and assay conditions, a higher antioxidant capacity assessed using the DPPH and ABTS methods was obtained in the brown seaweed P. durvillaei (EC50 value= 16.9 and 1.56 mg/mL, respectively), while lower values were obtain in the red seaweed S. filamentosa (EC50 value= 254.5 and 3.41 mg/mL, ). In accordance, a related species Padina gymnospora from the Gulf of Mexico showed the second highest antioxidant capacity (EC50 value = 3.45 mg/mL) as assessed using DPPH among all of the brown seaweed species analyzed (Zubia et al. Citation2007).
Antioxidants exert an important effect on decreasing the concentration of free radicals that produce lipid peroxidization and DNA damage in living cells, thus protecting against mutations that cause cancer (Duthie et al. Citation1996). Different compounds have been identified as possible of exerting antimutagenic, anticancer and antiangiogenic activities. Among them, the photosynthetic pigments have been described as chemoprotective agents in some seaweed species (Pangestuti & Kim Citation2011). In the edible red seaweed Porphyra tenera, β-carotene, chlorophyll a and lutein extracted with methanol:acetone (1:1 v/v) have been identified as the main chemoprotective compounds against mutagen-induced umu C gene expression in S. typhimurium TA1535/pSK 1002 (Okai et al. Citation1996). A similar trend was observed in the green algae Chlorococcum humicola, whose methanolic extracts showed that the composition of total and individual carotenoids (i.e., astaxanthin, lutein and β-carotene) exerted a high antimutagenic activity on S. typhimurium TA98, TA100 and TA 102 (Bhagavathy et al. Citation2011). In agreement, we found that the antimutagenic activity of the green seaweeds, C. sertularioides and R. riparium, and in the red seaweed. S. filamentosa (both extracted with acetone) against AFB1 as assessed on S. typhimurium TA98 and TA100 in the Ames test showed the highest inhibition (≥95%) (). This particularly high antimutagenic activity was explained by the relative higher content of flavonoids and chlorophyll and phenols, and lesser extent of phenolic compounds and total carotenoids, assessed in the green and red seaweeds extracts, respectively ( and ). The relatively low content of chlorophylls assessed in the red seaweeds compared with the green seaweeds is likely compensated with other antioxidant compounds (i.e., phenols) to maintain their antioxidant and antimutagenic capacity as well.
As mentioned above, the seaweeds C. sertularioides, R. riparium and S. filamentosa extracted with acetone exhibited the highest antimutagenic activity (≥ 95%) assessed in both tester strains. Therefore, these seaweeds were selected for repeating the assay at lower concentrations (). As expected, the inhibition effect decreased as the seaweed extract concentration (3.0–0.0003 mg/plate) decreased (); however, according to the scale (% of reversion inhibition > 40 = strong-, 25–40 = moderate-, and <25 = weak antimutagenic activity) proposed by Ikken et al. (Citation1999), the antimutagenic activity remained strong (> 40%) in R. riparium assayed in both S. typhimurium tester strains at the lowest seaweed extract concentration. A similar effect was observed in the extracts of C. sertularioides and S. filamentosa, but the antimutagenic activity remained strong (> 40%) at higher concentrations (10 and 100 times) than that observed for R. riparium. The diminishing of the antimutagenic activity along with the seaweed extract concentration could be explained by a decrease in the antioxidant compounds contained in each seaweed extract. This effect was observed in the red seaweed S. filamentosa, but not in the green seaweeds C. sertularioides and R. riparium because the antimutagenic activity was more highly reduced in the former than in the latest seaweed species (approximately 5 versus 2 times), despite significant differences in the total chlorophyll content (90 versus 26 μg/mg of dry extract) between both the species. Such differences might be explained by the content of other antioxidant compounds (i.e., specific carotenoids, flavonoids and/or phenols) that could be interacting with the chlorophylls at distinct levels, thus exerting different responses in the antimutagenic activity among the seaweed extracts. Chlorophyll a, lutein and β-carotene are capable of differentially inhibiting the mutagenic activity of S. typhimurium (TA 1535/Psk 1002) when they were individually evaluated, but the inhibition was the highest when all these pigments were mixed, suggesting an additive suppressive effect of a combined treatment on the antimutagenic activity. Moreover, the inhibition of the mutagenic activity of S. typhimurium (TA 1535/Psk 1002) depended on the pigment composition and the relative content in the extract or sample, suggesting a strong association with the functions of pigments in the seaweeds (Okai et al. Citation1996). This finding was observed not only in the pigments isolated from the red seaweed P. tenera but also in the extracts from other sources (i.e., cyanobacteria, green tea and carrot) used as chemical standards (Okai et al. Citation1996). Therefore, the composition of pigments (i.e., carotenoids) was analyzed by HPLC to evaluate their particular effects on the antimutagenic activity.
In this work, the seaweeds extracted with acetone, but not with hexane or methanol, showed visible damage (i.e., apoptotic bodies and stress-shaped cells) to the cancer cell line M12.C3.F6 (murine B-cell lymphoma) (). We found that the highest antiproliferative activity occurred with the green seaweeds, C. sertularioides and R. riparium, and the red seaweed, S. filamentosa (). In accordance, most of the cytotoxic and antiproliferative activities of the 27 seaweed species from the Gulf of Mexico, assessed against several cancer cell lines, were mainly observed in the green and brown seaweed extracts, while only a red seaweed exhibited an outstanding cytotoxic activity (Moo-Puc et al. Citation2009).
Although some other seaweed species showed noteworthy antiproliferative values (), C. sertularioides, R. riparium and S. filamentosa showed the highest antimutagenic activity. Thus, these species were selected to perform the MTT assay at lower seaweed extracts concentrations (). As expected, cancer cell proliferation inhibition decreased as the concentration of the seaweed extract increased. Although in R. riparium the lowest cell proliferation (29% or 71% of antiproliferative effect) occurred at the highest seaweed extract concentration (100 μg/mL), this result is promising because the same seaweeds species collected in India showed a higher IC50 value on the proliferation of HeLA cells (506 μg/mL) (Paul & Kundu Citation2013). In contrast, the highest cancer cell proliferation inhibition was exclusively achieved by the green seaweed, C. sertularioides, at the lowest concentration assayed (37 and 72% for 12.5 and 25 μg/mL, respectively). According to the classification of the US National Cancer Institute, an extract is considered active if exhibits an IC50 value ≤ 30 μg/mL against cancer cell lines (Suffness & Pezzuto Citation1990). Thus, the extract from this seaweed species should be considered for further purification to identify and isolate the bioactive compounds that are involved in the chemoprotective activity.
The cytotoxic and antiproliferative activity of several green, brown and red seaweed extracts appear to be related to their content of lipophilic compounds, phenols and lectins, respectively (Moo-Puc et al. Citation2009); however, variations in lipid content and composition (i.e., fatty acids) did not explained the antiproliferative activity assessed against several cancer cell lines in cultured green seaweeds Penicillus dumetosus (Moo-Puc et al. Citation2011b) and Udotea flavelum (Moo-Puc et al. Citation2011a). Moreover, flavonoids and to a lesser extent phenolic compounds, contained in brown and red seaweeds mainly extracted with organic solvents, exerted a higher toxicity and growth cell inhibition in lung adenocarcinoma (A549) and osteosarcoma (MG-63) than in colon (HCT-15) and prostate (PC-3) cancer cells (Murugan & Iyer Citation2014). A screening among brown seaweed species Dictyota ciliolata, Sargassum fluitans, Turbinaria tricostata and Padina sancta-crusis showed that only the last species extracted with dichloromethane and ethanol inhibited the cell proliferation of human breast cancer (MCF-7), but they were ineffective on kidney (HEK 293), prostate (LNCaP) and liver (Hep-G2) cancer cell lines (Caamal-Fuentes et al. Citation2014b). Later work identified that two sterols (i.e., fucosterol and 24ξ-hydroperoxy-24-vinylcholesterol) and two diterpenes (i.e., pachydictol A and dictol B) from the same brown seaweed species inhibited cell proliferation of both human breast (MCF-7) and cervix (SiHa) cancer (Caamal-Fuentes et al. Citation2014a). Meanwhile, phenolic compounds (i.e., phlorotannins) and mycosporine-like amino acids (MAAs) contained in some brown and red seaweeds were found to inhibit cell proliferation of human cervical adenocarcinoma (Yuan & Walsh Citation2006). Therefore, the higher antiproliferative activity observed in green seaweeds C. sertularioides and R. riparium could be due to their content of lipophilic compounds, while in the red seaweed S. filamentosa, this activity was possibly related to some particular proteins (i.e., lectins) or functional polysaccharides. Based on the above, further studies to evaluate the specific composition of pigments, sterols and fatty acids of these seaweed species are suggested. Additionally, the acquisition and study of aqueous extracts to assess their composition and chemoprotective activity is also suggested.
In general, marine organisms have been considered as an important source of therapeutic natural compounds. The increase in research focused on seaweeds is due to the great diversity of the species and also the considerable advances that have been made regarding their farming. These specimens are also characterized as producing several secondary metabolites that have shown high chemoprotective activity, but the observed responses suggest a species-specific effect that could also be related to the general extraction conditions. Therefore, novel extractions techniques (i.e., supercritical fluid extraction) that are characterized by minimal or no use of organic solvents should be evaluated to increase not only the efficiency of the extraction in terms of time and yield but also to increase the stability of the biocompounds without losing their activity (Michalak & Chojnacka Citation2014). This method will allow a more accurate evaluation of the yield and effectiveness of extracts of several species, including seaweeds.
In conclusion, the chemoprotective activity was evaluated in seven seaweed species from the coast of Sinaloa in the Pacific Ocean. Higher yield extraction was obtained in seaweeds extracted with methanol, compared with acetone and hexane. Despite this, the variations in yield extraction were not explained by the content of antioxidant compounds assayed (i.e., phenolic compounds, flavonoids and pigments), except in acetone seaweeds extracts, where variations in the yield extraction were correlated with the content of phenolic compounds and total carotenoids. Antioxidant capacity was higher in green (i.e., U. expansa, C. sertularioides, R. riparium and C. isabelae) and red (i.e., S. filamentosa and G. vermiculophylla) in comparison with brown (i.e., P. durvillaei) seaweed species. Antioxidant capacity was higher in acetone and methanol than that in hexane seaweeds extracts; however, antioxidants assessed (i.e., flavonoids and pigments) explained the variations in antioxidant capacity of seaweeds extracted in hexane. This result suggest that organic solvent used to perform the extraction exerted a significant effect on the stability of extracted compounds, or some other compounds contained at each solvent extraction are interacting or enhancing the antioxidant capacity, particularly in hexane seaweed extracts. The highest antioxidant, antimutagenic and antiproliferative activities occurred in the acetonic extracts, particularly for the green seaweeds, C. sertularioides and R. riparium, and red seaweed, S. filamentosa. The antioxidant and antimutagenic activities were explained by the content of flavonoids and chlorophylls, and to a lesser extent, by the phenolic compounds and carotenoids content assessed in these seaweed species. Significant efforts should be made to identify and isolate the bioactive compounds that are involved in the antioxidant, antimutagenic and antiproliferative activities of these seaweed species, but particularly in C. sertularioides, which showed a high potential for further investigations to propose novel therapeutic biocompounds.
Funding information
This research was supported by grants PROFAPI 2013/084 to M.A. Hurtado-Oliva and PROFAPI 2013/124 to M. Nieves-Soto. The authors are especially grateful to Laboratorio de Ecosistemas y Variabilidad Climática and Grant PROFAPI 2013/106 to M. M. Manzano-Sarabia for their invaluable assistance during the seaweed sampling. The first author was supported by a fellowship (No. 336726) from CONACYT.
Acknowledgements
The authors are grateful to Rosa Stephanie Navarro Peraza, Francisco Flores Cardenas, Maricruz Paredes Magaña, Juan Manuel Flores Alarcón, Jennifer Guadalupe Carrillo Tirado, Evelia Lorena Coss Navarrete, Rolando Inzunza Arroyo, Gloria Berenice Loaiza Aguilar and Karen Lillian Rodríguez for their technical assistance during the collection and analysis of the samples.
Disclosure statement
The authors report that they have no conflicts of interest.
References
- Awika JM, Rooney LW, Wu X, Prior RL, Cisneros-Zevallos L. 2003. Screening methods to measure antioxidant activity of sorghum (Sorghum bicolor) and sorghum products. J Agric Food Chem. 51:6657–6662.
- Bhagavathy S, Sumathi P, Madhushree M. 2011. Antimutagenic assay of carotenoids from green algae Chlorococcum humicola using Salmonella typhimurium TA98, TA100 and TA102. Asian Pac J Trop Dis. 1:308–316.
- Caamal-Fuentes E, Chale-Dzul J, Moo-Puc R, Freile-Pelegrin Y, Robledo D. 2014a. Bioprospecting of brown seaweed (Ochrophyta) from the Yucatan Peninsula: cytotoxic, antiproliferative, and antiprotozoal activities. J Appl Phycol. 26:1009–1017.
- Caamal-Fuentes E, Moo-Puc R, Freile-Pelegrín Y, Robledo D. 2014b. Cytotoxic and antiproliferative constituents from Dictyota ciliolata, Padina sanctae-crucis and Turbinaria tricostata. Pharm Biol. 52:1244–1248.
- Chang H-Y, Ho Y-L, Sheu M-J, Lin Y-H, Tseng M-C, Wu S-H, Huang G-J, Chang Y-S. 2007. Antioxidant and free radical scavenging activities of Phellinus merrillii extracts. Bot Stud. 48:407–417.
- Duthie SJ, Ma A, Ross MA, Collins AR. 1996. Antioxidant supplementation decreases oxidative DNA damage in human lymphocytes. Cancer Res. 56:1291–1295.
- Floegel A, Kim D-O, Chung S-J, Koo SI, Ock K Chun. 2011. Comparison of ABTS/DPPH assays to measure antioxidant capacity in popular antioxidant-rich US foods. J Food Compost Anal. 24:1043–1048.
- Halliwell B. 1996. Antioxidants in human health and disease. Annu Rev Nutr. 16:33–50.
- Huang H-L, Wang B-G. 2004. Antioxidant capacity and lipophilic content of seaweeds collected from the Qingdao coastline. J Agric Food Chem. 52:4993–4997.
- Hurtado MA, Racotta IS, Arcos F, Morales-Bojórquez E, Moal J, Soudant P, Palacios E. 2012. Seasonal variations of biochemical, pigment, fatty acid, and sterol compositions in female Crassostrea corteziensis oysters in relation to the reproductive cycle. Comp Biochem Physiol B: Biochem Mol Biol. 163:172–183.
- Ikken Y, Morales P, Martínez A, Marín ML, Haza AI, Cambero MI. 1999. Antimutagenic effect of fruit and vegetable ethanolic extracts against N-nitrosamines evaluated by the Ames test. J Agric Food Chem. 47:3257–3264.
- Laurienzo P. 2010. Marine polysaccharides in pharmaceutical applications: an overview. Mar Drugs. 8:2435–2465.
- Llorente-Bousquets J, Ocegueda S. 2008. Capital natural de México, Vol. I: conocimiento actual de la biodiversidad. México: CONABIO.
- Luximon-Ramma A, Bahorun T, Soobrattee MA, Aruoma OI. 2002. Antioxidant activities of phenolic, proanthocyanidin, and flavonoid components in extracts of Cassia fistula. J Agric Food Chem. 50:5042–5047.
- Marigo G. 1973. Sur une méthode de fractionnement et d’estimation des composés phénoliques chez les végétaux. Analusis. 2:106–110.
- Marinova E, Yanishlieva N. 1997. Antioxidative activity of extracts from selected species of the family Lamiaceae in sunflower oil. Food Chem. 58:245–248.
- Maron DM, Ames BN. 1983. Revised methods for the Salmonella mutagenicity test. Mutat Res-Environ Mutat. 113:173–215.
- Michalak I, Chojnacka K. 2014. Algal extracts: technology and advances. Eng Life Sci. 14:581–591.
- Michalak I, Chojnacka K. 2015. Algae as production systems of bioactive compounds. Eng Life Sci. 15:160–176.
- Mohamed S, Hashim SN, Rahman HA. 2012. Seaweeds: a sustainable functional food for complementary and alternative therapy. Trends Food Sci Technol. 23:83–96.
- Moo-Puc R, Robledo D, Freile-Pelegrin Y. 2011a. Enhanced antitumoral activity of extracts derived from cultured Udotea flabellum (Chlorophyta). Evid Based Complement Alternat Med. 2011:969275.
- Moo-Puc R, Robledo D, Freile-Pelegrin Y. 2011b. Improved antitumoral activity of extracts derived from cultured Penicillus dumetosus. Trop J Pharm Res. 10:177.
- Moo-Puc R, Robledo D, Freile-Pelegrín Y. 2009. In vitro cytotoxic and antiproliferative activities of marine macroalgae from Yucatán, Mexico Actividad citotóxica y antiproliferativa in vitro de macroalgas marinas de Yucatán, México. Cienc Mar. 35:345–358.
- Mosmann T. 1983. Rapid colorimetric assay for cellular growth and survival: application to proliferation and cytotoxicity assays. J Immunol Methods. 65:55–63.
- Müller L, Fröhlich K, Böhm V. 2011. Comparative antioxidant activities of carotenoids measured by ferric reducing antioxidant power (FRAP), ABTS bleaching assay (αTEAC), DPPH assay and peroxyl radical scavenging assay. Food Chem. 129:139–148.
- Murugan K, Iyer VV. 2014. Antioxidant and antiproliferative activities of extracts of selected red and brown seaweeds from the Mandapam Coast of Tamil Nadu. J Food Biochem. 38:92–101.
- Ochoa-Izaguirre MJ, Aguilar-Rosas R, Aguilar-Rosas LE. 2007. Catálogo de Macroalgas de las lagunas costeras de Sinaloa. 1st ed. Mazatlán, Sinaloa, Mexico: Universidad Nacional Autónomade México, Institutode Cienciasdel Mary Limnología.
- Okai Y, Higashi-Okai K, Yano Y, Otani S. 1996. Identification of antimutagenic substances in an extract of edible red alga, Porphyra tenera (Asadusa-nori). Cancer Lett. 100:235–240.
- Pangestuti R, Kim S-K. 2011. Biological activities and health benefit effects of natural pigments derived from marine algae. J Funct Foods. 3:255–266.
- Paul S, Kundu R. 2013. Antiproliferative activity of methanolic extracts from two green algae, Enteromorpha intestinalis and Rizoclonium riparium on HeLa cells. DARU. 21:72. doi:10.1186/2008-2231-21-72.
- Peinado I, Girón J, Koutsidis G, Ames JM. 2014. Chemical composition, antioxidant activity and sensory evaluation of five different species of brown edible seaweeds. Food Res Int. 66:36–44.
- Przygodzka M, Zielińska D, Ciesarová Z, Kukurová K, Zieliński H. 2014. Comparison of methods for evaluation of the antioxidant capacity and phenolic compounds in common spices. LWT-Food Sci Technol. 58:321–326.
- Rosaline XD, Sakthivelkumar S, Rajendran K, Janarthanan S. 2012. Screening of selected marine algae from the coastal Tamil Nadu, South India for antibacterial activity. Asian Pac J Trop Biomed. 2:S140–S146.
- Serviere-Zaragoza E, Hurtado MA, Manzano-Sarabia M, Mazariegos-Villarreal A, Reza M, Arjona O, Palacios E. 2015. Seasonal and interannual variation of fatty acids in macrophytes from the Pacific coast of Baja California Peninsula (Mexico). J Appl Phycol. 27:1297–1306.
- Shalaby E. 2011. Algae as promising organisms for environment and health. Plant Signal Behav. 6:1338–1350.
- Souza BW, Cerqueira MA, Martins JT, Quintas MA, Ferreira AC, Teixeira JA, Vicente AA. 2011. Antioxidant potential of two red seaweeds from the Brazilian coasts. J Agric Food Chem. 59:5589–5594.
- Stengel DB, Connan S, Popper ZA. 2011. Algal chemodiversity and bioactivity: sources of natural variability and implications for commercial application. Biotechnol Adv. 29:483–501.
- Suffness M, Pezzuto JM. 1990. Assays related to cancer drug discovery. Methods Plant Biochem: Assays Bioact. 6:71–133.
- Thaipong K, Boonprakob U, Crosby K, Cisneros-Zevallos L, Hawkins Byrne D. 2006. Comparison of ABTS, DPPH, FRAP, and ORAC assays for estimating antioxidant activity from guava fruit extracts. J Food Compost Anal. 19:669–675.
- Wellburn AR. 1994. The spectral determination of chlorophylls a and b, as well as total carotenoids, using various solvents with spectrophotometers of different resolution. J Plant Physiol. 144:307–313.
- Yuan YV, Walsh NA. 2006. Antioxidant and antiproliferative activities of extracts from a variety of edible seaweeds. Food Chem Toxicol. 44:1144–1150.
- Zar J. 1999. Biostatistical analysis. New Jersey: Prentice-Hall.
- Zaragozá M, López DP, Sáiz M, Poquet M, Pérez J, Puig-Parellada P, Màrmol F, Simonetti P, Gardana C, Lerat Y, et al. 2008. Toxicity and antioxidant activity in vitro and in vivo of two Fucus vesiculosus extracts. J Agric Food Chem. 56:7773–7780.
- Zertuche-González J. 1993. Situación actual de la industria de las algas marinas productoras de ficocoloides en México. In: Zertuche-González JA, editor. Situación Actual De La Industria De Macroalgas Productoras De Ficocoloides En América Latina Y El Caribe FAO, Mexico 33–37.
- Zubia M, Robledo D, Freile-Pelegrin Y. 2007. Antioxidant activities in tropical marine macroalgae from the Yucatan Peninsula, Mexico. J Appl Phycol. 19:449–458.