Abstract
Context: Co-administration of amodiaquine with MAMA decoction (MD), an herbal antimalarial drug comprising the leaves of Mangifera indica L. (Anacardiaceae), Alstonia boonei De Wild (Apocynaceae), Morinda lucida Benth (Rubiaceae) and Azadirachta indica A. Juss (Meliaceae) was investigated. The practice of concurrent administration of herbal medicines with orthodox drugs is currently on the increase globally.
Objective: The study was designed to investigate the possible enhancement of the antimalarial potency as well as possible herb–drug interaction resulting from concurrent administration of MAMA decoction with amodiaquine (AQ).
Materials and methods: Combinations of MD with AQ were investigated in chloroquine (CQ)-sensitive Plasmodium berghei NK 65 in varying oral doses (mg/kg) at: sub-therapeutic [MD30 + AQ1.25], therapeutic [MD120 + AQ10] and median effective [MD40 + AQ3.8], using chemosuppressive and curative antimalarial test models. Secondly, P. berghei ANKA (CQ-resistant)-infected mice were orally treated with MD 120, 240, [MD120 + AQ10] and [MD240 + AQ10] mg/kg, using both models. The survival times of mice were monitored for 28 d.
Results: ED50 values of MD and AQ were 48.8 and 4.1 mg/kg, respectively. A total parasite clearance of CQ-sensitive P. berghei NK65 was obtained with the therapeutic combination dose in the curative test giving an enhanced survival time. In CQ-resistant P. berghei ANKA-infected mice, [MD120 + AQ10] and [MD240 + AQ10] mg/kg gave comparable activities with AQ (10 mg/kg) in both models.
Conclusion: The therapeutic combination dose gave total parasite clearance of CQ-sensitive P. berghei NK65, whereas none of the doses tested showed notable activity against CQ-resistant P. berghei ANKA.
Introduction
Malaria is an endemic disease in the tropics, presenting with about 198 million new cases and 0.6 million mortalities yearly (WHO, 2014). In Africa, the use of herbal preparations is common practice in the management of diseases and is often considered as first line therapy in malarial infection, most often as self-medication initiatives (Gasquet et al. Citation1993; Idowu et al. Citation2008). Historically, plants as major sources of drugs have produced two important antimalarial drugs, namely quinine and artemisinin, both of which have served as chemical leads to several synthetic antimalarial analogues. The increasing spread of Plasmodium resistance to the anti-folates and 4-aminoquinolines, which have been the mainstay of malaria chemotherapy for several years, has led the WHO to recommend the current artemisinin-based combination therapy (ACT). Nevertheless, the reported incidence of decreased sensitivity of Plasmodium parasites to the ACT drugs in some parts of Asia is of great concern and challenge (Dondorp et al. Citation2009). Therefore, there is an urgent need for innovative and alternative strategies to combat the resistant strains of the parasite. Some studies have shown that plant-based preparations, co-administered with orthodox drugs, produced better antimalarial activities on both chloroquine (CQ)-sensitive and CQ-resistant Plasmodium strains (Ahorlu et al. Citation1997; Muregi et al. Citation2003, Citation2007; Adibe Citation2009; Anagu et al. Citation2014). MAMA decoction (MD), an herbal remedy prepared from a mixture of the leaves of Mangifera indica L. (Anacardiaceae), Alstonia boonei De Wild (Apocynaceae), Morinda lucida Benth (Rubiaceae) and Azadirachta indica A. Juss (Meliaceae) in equal proportions, has been reported to possess antimalarial activities on CQ-sensitive P. berghei NK65 in rodents with acceptable safety (Akanmu et al. Citation2013; Adepiti et al. Citation2014; Odediran et al. Citation2014). It has been pilot-produced and marketed by the Drug Research and Production Unit, Faculty of Pharmacy, Obafemi Awolowo University, Nigeria, since 2000 with observational reports of efficacy and safety by voluntary users. Amodiaquine (AQ), a 4-aminoquinoline drug with antimalarial activity against both CQ-sensitive and CQ-resistant P. falciparum (Mariga et al. Citation2004), has a long duration of action, which has made it a partner drug-of-choice in the WHO-recommended ACT. The medical culture in Africa, poor economy, ready availability of medicinal herbs and direct accessibility to over-the-counter antimalarial drugs may have encouraged the local populations to indulge in herb-drug combination therapies with unreported and undocumented possible harmful or beneficial interactions. Hence, this study was carried out to evaluate the antimalarial property of MD when combined with AQ and tested on mice infected with either CQ-sensitive or CQ-resistant strains of P. berghei.
Materials and methods
Drug and reagent
Amodiaquine hydrochloride dihydrate (Sigma, St. Louis, MO) Giemsa stain (Wuhan Chemicals, China).
Experimental animals and parasites
Swiss albino mice (18–24 g), with free access to water and commercial food pellets (Premier Feed Mills, Nigeria), were used in accordance with the ‘Guide for the care and use of laboratory animals’ (National Research Council Citation1996). The Health Research Ethics Committee of the Obafemi Awolowo University, Ile-Ife, Nigeria, approved the study (IPHOAU/12/90).
P. berghei NK65 (CQ-sensitive) and P. berghei ANKA (CQ-resistant) parasites (Malaria Research and Reference Reagent Resource Centre [MR-4, USA]) were maintained by continuous blood passage in different donor mice. A standard inoculum of 107 parasitized red blood cells (RBC) was administered by intra-peritoneal route (0.2 mL) to each test mouse.
Preparation of MD
MD was prepared as before (Adepiti et al. Citation2014) from a 1:1:1:1 mixture of leaves of Mangifera indica L. (Anacardiaceae), Alstonia boonei De Wild (Apocynaceae), Morinda lucida Benth. (Rubiaceae) and Azadirachta indica A. Juss (Meliaceae).
Determination of doses
The median effective doses (ED50) were determined from previous experiments using MD and AQ as single doses at 15–240 mg/kg and 1.25–10 mg/kg, respectively (Adepiti et al. Citation2014). Sub-therapeutic doses were taken as doses less than the ED50 values while doses that gave chemosuppression greater than 75% were taken as therapeutic doses.
Chemosuppressive (four d) test model (Peters et al. Citation2002).
(a) Different groups of mice (5 mice per group) infected with P. berghei NK 65 (CQ-sensitive) were treated with oral single doses of MD at 30, 40 and 120 mg/kg and AQ at 1.25, 3.8 and 10 mg/kg as well as their combinations at sub-therapeutic [MD30 + AQ1.25] mg/kg, median effective [MD40 + AQ3.8] mg/kg and therapeutic [MD120 + AQ10] mg/kg doses, 4 h (D0) post-inoculation (p.i.) and repeated daily for three more days (D1–D3). The untreated (negative control) group received 0.2 mL distilled water. On day 4 p.i., a thin blood smear was made from the tail of each mouse, fixed with methanol and stained with 10% Giemsa solution followed by microscopic evaluation (× 1000 magnification) of the parasitized and total red blood cells.
Similarly, in a separate chemosuppressive experiment, P. berghei ANKA-infected (CQ-resistant) mice were treated with MD120, MD 240, [MD120 + AQ10] and [MD240 + AQ10] mg/kg, respectively, for 4 d (D0–D3). The untreated (negative control) group received 0.2 mL distilled water. On days 4 and 7 p.i., thin blood smears were made and the procedure completed as above.
Curative (established malaria infection or Rane) test model (Ryley & Peters Citation1970).
(a) P. berghei NK65 (CQ-sensitive)-infected mice were treated orally with single doses of MD30, MD120 mg/kg, AQ1.25 and AQ10 mg/kg as well as their respective combinations at [MD30 + AQ1.25] and [MD120 + AQ10] mg/kg, once daily at 72 h p.i. for 5 d (D3–D7). The untreated group received 0.2 mL distilled water while thin blood smears were prepared from the tail of each mouse daily, to monitor the parasitaemia levels and the procedure completed as above.
In a separate experiment, P. berghei ANKA-infected (CQ-resistant) mice were orally treated with single doses of MD at 120, 240 and 480 mg/kg and AQ at 10 mg/kg alone (positive control) as well as their combinations at [MD120 + AQ10] and [MD240 + AQ10] mg/kg/day at 72 h p.i. for 5 d (D3–D7). The untreated group received 0.2 mL distilled water while thin blood smears were prepared from the tail of each mouse daily, to monitor the parasitaemia levels and the procedure completed as described above.
Determination of the rectal temperature and survival time
In both test models, the rectal temperature of each mouse was taken daily throughout the period of drug administration. Temperature values at the start (day 0) and at the end [day 3 for chemosuppressive or day 7 for curative test] of drug treatment were compared. In addition, mortality was monitored daily (after the commencement of drug administration) and particularly for days 21 and 28 in order to determine the survival rate for each group of mice.
Data and statistical analysis
The average parasitaemia was determined by obtaining the percentage of the ratio of parasitized to the total number of RBC. Average percentage chemosuppression (or parasite clearance) was calculated as 100 × [(A–B)/A], where A is the average parasitaemia of the negative control group and B is the average parasitaemia of the test group. The effective median doses (ED50) of MD and AQ were determined using Microsoft Excel while the one way analysis of variance between groups (ANOVA) and post hoc Dunnett test were used to compare data for the treatment groups (GraphPad Instat Citation2003). The value of p < 0.05 was considered as being statistically significant.
Results
Chemosuppressive (4 d) test model
Chloroquine-sensitive P. berghei NK65
The effects of the combination of MD and AQ at sub-therapeutic, median effective and therapeutic doses on P. berghei NK65 (CQ-sensitive) are presented in . From the results obtained for the individual agents of the combination, the ED50 values of MD and AQ were determined as 48.8 and 4.1 mg/kg, respectively. Treatments with [MD15 + AQ1.25], [MD30 + AQ1.25] and [MD120 + AQ10] mg/kg gave chemosuppression values of 25.12 ± 7.87, 30.83 ± 13.68 and 79.10 ± 1.59%, respectively. The values obtained for infected mice treated individually with MD15, MD30 and AQ1.25 mg/kg alone were not significantly different from those of their respective combinations (p > 0.05), though they were significantly different from the values obtained with their respective negative controls (p < 0.0001). However, the activity level of 51.6% observed in mice treated with MD40 mg/kg alone was significantly different (p = 0.0022) from that of its median-effective-dose combination [MD40 + AQ3.8] mg/kg (83.0%). Although treatment with [MD120 + AQ10] mg/kg gave a non-significant increase (p > 0.05) in chemosuppression compared with the activity of the individual drugs, a significant (p <0.05) increase in temperature reversal was observed (). Mice treated with AQ and MD at both sub-therapeutic and therapeutic combination doses gave 40% survival by day 21 ().
Table 1. Average parasitaemia, chemosuppression and survival rate of CQ-sensitive P. berghei NK65-infected mice treated with single and combination doses of MAMA decoction and amodiaquine.
Table 2. Effects of single and combination doses of MAMA decoction and amodiaquine on the temperatures of CQ-sensitive P. berghei NK65-infected mice using the chemosuppressive and Rane tests.
The effects of the individual and combination doses of MD and AQ on the temperatures of CQ-sensitive P. berghei-infected mice are shown in . Unlike in human malaria, body temperatures are known to decrease in murine malarial infection but increase when the malaria is effectively treated. The temperatures of mice treated with MD30, MD120 and [MD120 + AQ10] mg/kg at the end of drug treatment (D3) were significantly different (p = 0.003) from the corresponding initial values at day 0.
Chloroquine-resistant P. berghei ANKA
The effects of the individual and combination doses of MD and AQ on mice infected with P. berghei ANKA (CQ-resistant) are presented in . The chemosuppression of MD 120 alone was 0.0% on day 4 but increased significantly to 58.9% on day 7, whereas MD240 mg/kg alone gave 27.5% on day 4, which was not significantly different from its activity of 28.5% on day 7. The combination doses of [MD120 + AQ10] mg/kg and [MD240 + AQ10] mg/kg showed comparable activities (p > 0.05) with the chemosuppression values of 83.4 and 84.7% on day 4 while day 7 values were 90.5 and 90.5%, respectively. The positive control (AQ10 mg/kg alone), exhibited an activity of 72.5% on day 4, which was significantly different (p < 0.05) from its activity on day 7 (87.7%). Although there was no chemosuppressive activity observed for MD120 mg/kg alone (0.0%) on day 4, only 20% of the treated mice survived by day 21 while the higher combination dose of [MD240 + AQ10] mg/kg showed consistent and significantly good profile with 80% of the treated mice surviving on day 21 up to the end of the experiment (day 28) []. Similarly, the mice treated with AQ alone exhibited 80% survival till day 21 but only 40% survived up to the end of the experiment.
Table 3. Effects of single and combination doses of MAMA Decoction and amodiaquine on CQ-resistant P. berghei ANKA-infected mice using the chemosuppressive test.
Curative test
Chloroquine-sensitive P. berghei NK65
Parasitaemia values, in the negative (untreated) control for P. berghei NK65-infected (CQ-sensitive) mice, increased steadily from 11.9 to 48.7% (D3 to D7) as shown in . The combination dose of [MD120 + AQ10] mg/kg gave a 100% parasite clearance on day 5 and no recrudescence was observed when monitored till termination of the experiment (28 d post-drug administration). In addition, 100% survival was recorded for all the animals in this group (), which was higher than the survival of mice treated with the individual drugs of the combination. The mean temperature of mice treated with the combination was also significantly (p < 0.05) increased when compared to the negative control group and the individual drugs alone ().
Chloroquine-resistant P. berghei ANKA
The P. berghei ANKA (CQ-resistant)-infected mice in the negative control group showed parasitaemia values increasing from 5.3 to 20.9% on D3 to D7 (). MD120 mg/kg exhibited no curative effect during the period of the drug administration. In addition, MD 240 mg/kg gave parasite clearance between 17.1 and 60.1% on D3 and D6 but reduced to 40.2% on D7 while MD480 mg/kg showed activity from 18.4 ± 7.7 to 48.8 ± 3.7%. On D6, the curative activity of [MD240 + AQ10] mg/kg (94.3 ± 0.5%) was significantly higher than that of AQ (89.7 ± 0.5%). MD120 + AQ10 (82.0 ± 4.6%) and [MD240 + AQ10] mg/kg (91.9 ± 0.8%) on day 7 of the experiment, were comparable (p > 0.05) to one another and to AQ (). The treated mice did not exhibit any favourable temperature reversal since all the treatment groups showed significantly lower temperature values at the end of drug administration (D7) compared to the start of the experiment (D3) (). Mortality, as expected, occurred in the negative control group between days 4 and 7 while mice in the groups treated with AQ10 or [MD120 + AQ10] mg/kg showed 40 and 20% survival on day 21 and 40 and 0.0% survival on day 28, respectively ().
Figure 2. Effects of the combination of amodiaquine and MAMA decoction on CQ-resistant P. berghei ANKA-infected mice using the Rane test model.
Negative control (□), AQ 10 mg/kg (•), MD 120 mg/kg (Δ), MD 240 mg/kg (▪), MD 480 mg/kg (⋄), MD120 plus AQ 10 mg/kg (▴), MD240 plus AQ10 mg/kg (×).
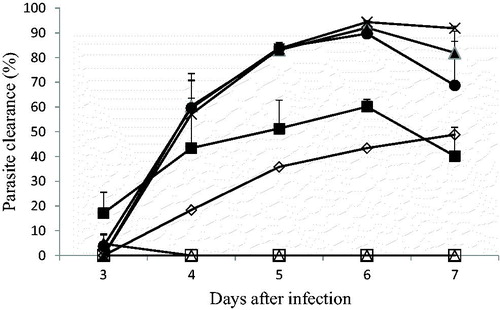
Table 4. Effects of single and combination doses of MAMA Decoction and amodiaquine on the temperatures of CQ-resistant P. berghei ANKA-infected mice.
Table 5. Survival rate of CQ-resistant P. berghei ANKA-infected mice treated with single and combination doses of MAMA Decoction and amodiaquine using the Rane test.
Discussion
The primary goal of this study was to obtain a favourable herb-drug outcome, such as activity-enhancement and/or combating the problem of parasite resistance using the combination of MD and AQ. The study has achieved a remarkable potentiation of the antimalarial action of AQ when given in combination with MD, against CQ-sensitive P. berghei, at the therapeutic doses of both drugs. The observed herb–drug interaction exhibited a significant reduction in parasitaemia, achieving a total ‘parasite cure’ (100% clearance) with enhanced survival rate and a remarkable temperature reversal in the treated animals. Pharmacologically, herb–drug interactions can be divided into three well-defined mechanistic outcomes: additive, synergistic and antagonistic effects. Potentiation of the activities of both MD and AQ was evident in this study for the CQ-sensitive parasite, which is suggestive of an additive or synergistic effect. Previous reports have shown that MD alone possessed remarkable chemosuppressive and moderate curative activities in CQ-sensitive P. berghei-treated mice (Adepiti et al. Citation2014; Odediran et al. Citation2014). The combination of MD with AQ at sub-therapeutic doses in this study did not exhibit any significant parasitaemia reduction, chemosuppression, parasite clearance or temperature reversal in the treated mice when compared with the individual drugs alone. On the other hand, an extract of Khaya grandifoliola at sub-therapeutic doses, was found to potentiate the chemosuppressive activities, with enhanced parasite clearance, of sub-optimal doses of halofantrine and artemisinin (Ijarotimi et al. Citation2010; Agbedahunsi et al. Citation2013). Sub-therapeutic doses can facilitate the study of potential interaction outcomes with a view to exploring the cost-effectiveness of the partner drugs when eventually co-formulated. The optimal dose combination for the CQ-sensitive murine Plasmodium infection in this study therefore, was [MD120 + AQ10] mg/kg, which could be likened to artesunate-amodiaquine combination therapy given in their respective therapeutic doses. AQ is a 4-aminoquinoline anti-malarial drug with structural similarity to CQ and has been reported to possess significant activity against CQ-resistant Plasmodium falciparum and P. vivax (Gelb Citation2007; Tarning et al. Citation2012).
When screened against the CQ-resistant Plasmodium strain, and at the lower dose of MD120 mg/kg plus the therapeutic dose of AQ10 mg/kg, the curative activity was merely comparable to AQ alone. Hence, the study has not demonstrated any significant herb-drug effect by MD when co-administered with AQ against CQ-resistant P. berghei ANKA strain. However, the higher dose combination of [MD240 + AQ10] mg/kg gave a significantly higher curative activity (94.3%) than AQ alone (89.7%) on day four post-drug administration but this potency was not significantly sustained on day five post-drug administration. The current global interest in antimalarial herb-drug research by scientists should be intensified in order to achieve the two major goals of safety and therapeutic conquest of parasite resistance when adequate efficacy has been established. Although Vernonia amygdalina L. (Asteraceae) leaf alone, when screened against CQ-resistant P. berghei, gave antimalarial activity (62.7%) merely comparable to CQ alone (57.2%), there was a higher chemosuppressive activity (80.7%) with a higher survival rate, when combined with CQ (Iwalokun Citation2008). Conventional drug-drug combinations have been documented for malaria and pain as well as other disease conditions, such as cancer and tuberculosis (Blomberg et al. Citation2001; Miranda et al. Citation2006; Zeidan et al. Citation2014). Several studies have also shown that combining conventional drugs with medicinal plant preparations could produce beneficial effects (Nwafor et al. Citation2003; Mohd Ridzuan et al. Citation2007). Therefore, as the world remains confronted with the resistance of Plasmodium to the commonly-used antimalarial drugs, urgent intellectual efforts are required to find an alternative strategy, such as herb-drug formulation, that will combat this scourge in antimalarial therapy. If successful for malarial therapy, such herb-drug theory could be proposed for other endemic, infectious and non-infectious diseases especially for Africa where herbal poly-pharmacy or multi-component formulation culture already exists. From the history and experience of Cinchona bark and Artemisia herb, it is believed that the fastest and most logical approach to the challenges of global threat by malaria parasite resistance would most probably be found in this type of traditional medical culture of the people.
Conclusion
The study concluded that the combination of MD and AQ at their individual therapeutic doses were effective in CQ-sensitive malaria infection when 100% parasite clearance was achieved. However, neither MD alone nor any of its combinations with AQ possessed notable activity against CQ-resistant murine malaria. Detailed pharmacokinetic studies are required to assess the clinical implications of the combination in human CQ-sensitive malaria therapy.
Funding information
This work was supported by the University Research Committee (URC) of the Obafemi Awolowo University, Ile-Ife, Nigeria [URC Grant 11813AFL].
Acknowledgements
We are grateful to Prof. O. G. Ademowo of the University College Hospital, Ibadan, Nigeria and Dr. G. Olayiwola of the Obafemi Awolowo University, Ile Ife, Nigeria for kindly providing access to P. berghei NK65 and P. berghei ANKA strains, respectively. Mr. O. A. Ajetunmobi is also acknowledged for technical assistance.
Disclosure statement
The authors report no declarations of interest.
References
- Adepiti AO, Elujoba AA, Bolaji OO. 2014. In vivo antimalarial evaluation of MAMA decoction on Plasmodium berghei in mice. Parasitol Res. 113:505–511.
- Akanmu MA, Akanmu AO, Adepiti AO, Osasan SA, Obuotor EM, Elujoba AA. 2013. A preliminary study on the toxicity and novelty-induced behavioral effects of herbal medicine (MAMA decoction®) in rats. Afr J Pharm Pharmacol. 7:1880–1885.
- Adibe MO. 2009. Prevalence of concurrent use of herbal and synthetic medicines among outpatients in a mission hospital in Nigeria. Int J Drug Dev Res. 1:60–66.
- Agbedahunsi JM, Umeevuruo IF, Elufioye TO, Adepiti AO. 2013. In vivo interaction between extracts of Khaya grandifoliola (Welw) CDC (Meliaceae) and artemisinin in a murine malarial model. Eur J Med Plant. 3:552–560.
- Ahorlu CK, Dunyo SK, Afari EA, Koram KA, Nkrumah FK. 1997. Malaria-related beliefs and behaviour in southern Ghana: implications for treatment, prevention and control. Trop Med Int Health. 2:488–499.
- Anagu OL, Attama AA, Okore VC, Gugu HT, Ngene AA, Esimone CO. 2014. Azadirachta indica extract-artesunic acid combination produces an increased cure rate of Plasmodium berghei-infected mice. Pharm Biol. 52:883–889.
- Blomberg B, Spinaci S, Fourier B, Laing R. 2001. The rationale for recommending fixed-dose combination tablets for treatment of tuberculosis. Bull World Health Organ. 79:61–68.
- Dondorp AM, Nosten F, Yi P, Das D, Phyo AP, Tarning J, Lwin KM, Arley F, Hanpithakpong W, Lee SJ, et al. 2009. Artemisinin resistance in Plasmodium falciparum malaria. N Engl J Med. 361:455–467.
- Gasquet M, Delmas F, Timon-David O, Keita P, Keita A, Guindo M, Koita N, Diallo D, Doumbo O. 1993. Evaluation of in vitro and in vivo of traditional antimalarial, “Malarial-5”. Fitoterapia. 64:423–426.
- Gelb MH. 2007. Drug discovery for malaria: a very challenging and timely endeavor . Curr Opin Chem Biol. 11:440–445.
- Graphpad Instat. 2003. GraphPad for windows v 3.06. San Diego, USA: GraphPad Software Inc.
- Idowu OA, Mafiana CF, Luwoye IJ, Adehanloye O. 2008. Perceptions and home management practices of malaria in some rural communities in Abeokuta, Nigeria. Travel Med Infect Dis. 6:210–214.
- Ijarotimi SO, Agbedahunsi JM, Onyeji CO, Adewunmi CO. 2010. Chemotherapeutic interaction between Khaya grandifoliola (WELW) CDC stem bark extract and two anti-malarial drugs in mice. Afr J Tradit Complement Altern Med. 7:370–376.
- Iwalokun BA. 2008. Enhanced antimalarial effects of chloroquine by aqueous Vernonia amygdalina leaf extract in mice infected with chloroquine resistant and sensitive Plasmodium berghei strains. Afr Health Sci. 8:25–35.
- Mariga ST, Gil JP, Sisowath C, Wernsdorfer WH, Bjorkman A. 2004. Synergism between amodiaquine and its major metabolite, desethylamodiaquine against Plasmodium falciparum in vitro. Antimicrob Agents Chemother. 48:4089–4096.
- Miranda HF, Puig MM, Prieto JC, Pinardi G. 2006. Synergism between paracetamol and non-steroidal anti-inflammatory drugs in experimental acute pain. Pain. 121:22–28.
- Mohd Ridzuan MA, Sow A, NooRain A, Mohd Ilham A, Zakiah I. 2007. Eurycoma longifolia extract-artemisinin combination parasitaemia suppression of Plasmodium yoelii infected mice. Trop Biomed. 24:111–118.
- Muregi FW, Chhabra SC, Njagi EN, Lang at-Thoruwa CC, Njue WC, Orago AS, Omar SA, Ndiege IO. 2003. In vitro antiplasmodial activity of some plants used in Kisii, Kenya against malaria and their chloroquine potentiation effects. J Ethnopharmacol. 84:235–239.
- Muregi FW, Ishih A, Suzuki T, Kino H, Amano T, Mkoji GM, Miyase T, Terada M. 2007. In vivo antimalarial activity of aqueous extracts from Kenyan medicinal plants and their chloroquine (CQ) potentiation effects against a blood-induced CQ-resistant rodent parasite in mice. Phytother Res. 21:337–343.
- National Research Council (NRC). 1996. Guide for the care and use of laboratory animals. 8th ed. Washington DC, USA: The National Academies Press.
- Nwafor SV, Akah PA, Okoli CO, Onyirioha AC, Nworu CS. 2003. Interaction between chloroquine sulphate and aqueous extract of Azadirachta indica A. Juss (Meliaceae) in rabbits. Acta Pharm. 53:305–311.
- Odediran SA, Elujoba AA, Adebajo AC. 2014. Influence of formulation ratio of the plant components on the antimalarial properties of MAMA decoction. Parasitol Res. 113:1977–1984.
- Peters W, Fleck SS, Robinson BB, Stewart LB, Jefford CW. 2002. The chemotherapy of rodent malaria. LX. The importance of formulation in evaluating the blood schizontocidal activity of some endoperoxide antimalarials. LXThe importance formulation evaluating blood activity some antimalarias. Ann Trop Med Parasitol. 96:559–573.
- Ryley JF, Peters W. 1970. The antimalarial activity of some quinoline esters. Am J Trop Med Parasitol. 84:209–211.
- Tarning J, Chotsiri P, Jullien V, Rijken MJ, Bergstrand M, Cammas M, McGready R, Singhasivanon P, Day NPJ, White NJ, et al. 2012. Population pharmacokinetic and pharmacodynamic modelling of amodiaquine and desethylamodiaquine in women with Plasmodium vivax malaria during and after pregnancy. Antimicrob Agents Chemother. 56:5764–5773.
- WHO (World Health Organisation). 2014. World malaria report; [cited 2015 Feb 19]. Available from: http://www.who.int/malaria/publications/world_malaria_report_2014/wmr-2014-key-points.pdf?ua=1.
- Zeidan A, Mazoit JX, Abdullah MA, Maalike H, Ghattas TH, Soifan A. 2014. Median effective dose (ED50) of paracetamol and morphine for postoperative pain: A study of interaction. Br J Anaesth. 112:118–123.