Abstract
Context Qiancao Naomaitong Mixture (QNM) is mainly used to treat ischemic stroke patients in the clinic.
Objective This study evaluates the protective effect of QNM on neuronal damage in vitro, and clarifies the underlying mechanism against cerebral ischemia-reperfusion (I/R) injury in vivo.
Materials and methods Activity assay of caspase 3 (C-3) and caspase 8 (C-8) were measured with microplate reader and cell apoptosis was investigated. Cerebral I/R injury was induced by MCAO model. QNM groups were given at 0.27, 0.54 and 1.08 mL/100 g body weight. The weight ratio of cerebral infarction tissue was obtained. The cytokine levels in serum and brain tissue were measured using ELISA.
Results Compared with the OGD group (C-3: 29.69 ± 5.63, C-8: 74.05 ± 6.86), 100 mg/mL QNM (C-3: 19.80 ± 2.62, C-8: 48.94 ± 6.41) and 200 mg/mL QNM (C-3: 16.28 ± 4.55, C-8: 41.08 ± 4.05) treatments decreased C-3 and C-8 activities significantly, and inhibited apoptosis of SH-SY5Y cells. The weight ratios of cerebral tissues in low, medium and high dose groups were 17.33 ± 5.1%, 17.78 ± 5.4% and 14.25 ± 4.2%, respectively, significantly lower than in control group. QNM also improved the cytokine levels in serum and brain tissue. In addition, histological examination indicated that dense neuropil and largely surviving neurons were seen in treated rats.
Conclusion QNM exerted protective effect by inhibiting the cell apoptosis in vitro. The protective mechanisms of QNM were associated with its properties of anti-apoptosis and antioxidation as well as improved neuronal nutrition in I/R rats.
Introduction
Cerebral ischemia is a common and frequently occurring disease seriously threatening human health. It shows a trend of increasing incidence rate each year and has become one of the major diseases with high morbidity and mortality (Zhou et al. Citation2008; Lakhan et al. Citation2009; Wu et al. Citation2010). Recent studies on the mechanism of ischemic brain injury have made rapid progress, demonstrating that factors such as neuronal damage caused by free radicals, excitatory amino acid toxicity, inflammation and cell apoptosis play important roles during the process of ischemic brain injury (Ritz et al. Citation2008; Mukerji et al. Citation2009). The ultimate outcome of neuronal damage caused by cerebral ischemia is the dysfunction or death of neuronal cells. Although many neuroprotective agents and a variety of treatment strategies have been developed based on the mechanism of cerebral I/R injury, they still cannot meet the therapeutic need. Medicinal plants contain a wide variety of chemical components and thus may form an ideal resource for the development of effective protective agent against ischemic brain injury (Park et al. Citation2009), especially the compound drugs that comprise multiple medicines, the multiple components of which may act on the different processes of brain I/R injury to reduce or improve the ischemic brain injury synergistically, via a variety of mechanisms. These drugs thus warrant an in-depth study and exploration.
QNM is an oral liquid made from water-boiled Boschniakia himalaica Hook. f. et Thoms. (Orobanchaceae) (Jin et al. Citation2008; Wan et al. Citation2012; Zhang et al. Citation2013) and Lysimachia barystachys Bge. (Primulaceae) (Wan et al. Citation2009) by modern pharmaceutical processing technology (Zhou et al. Citation2010). It was originally derived from the folk prescription of Bai people in Dali of Yunnan, China, and had over 40 years of history in treating stroke patients. The effective rate of QNM in clinical treatment of acute ischemic stroke reached 85.6% (Bao et al. Citation2006), with no adverse reactions and side effects observed in patients during the treatment process.
Although QNM has good clinical efficacy, analysis of its composition and systemic research on its pharmacological activity remain rare. Therefore, the present study analysed the content of the main component in QNM and investigated its protective effect on neuronal damage. Its activity against cerebral I/R injury in rats and the underlying mechanism were also explored.
Materials and methods
Experimental materials
Nimodipine tablets (lot No. 20111209, Pharmaceutical Co., Ltd. Hebei Yongfeng), Acetonitrile (ACN, Merck), Peroxidase-streptavidin kit (Beijing ZSGB-Bio Technology Co., Ltd.), DAB (Beijing ZSGB-Bio Technology Co., Ltd.), CAT Elisa kit, MDA Elisa kit, NO Elisa kit, SOD Elisa kit, ICAM-1 Elisa kit, TNF-α Elisa kit, BDNF Elisa kit and NGF Elisa kit were purchased from Ya Anda Biotechnology Co. (Beijing, China). Qiancao Naomaitong Mixture (National drug approval No. Z20025214, lot No. 20120810, supplied by Yunnan Baiyao Research Institute).
Method of extraction and preparation of QNM: Lysimachia barystachys (133.3 g) and Boschniakia himalaica (66.7 g) were crushed and extracted with distilled water (2 × 2 L, each 1.5 h) at 100 °C. The extract was mixed together, filtered and concentrated to 1.10 g/cm3 by heating at 60 °C. And then the solution was precipitated by adding ethanol to a final concentration of 70% (v/v) at room temperature. After 24 h precipitation, the sample was filtered, and then ethanol was recycled under reduced pressure. The residue was re-dissolved in distilled water (1000 mL) and the pH value was adjusted to 5 ∼ 7. QNM was filled into ampoule bottle and sealed. Finally, it was sterilized and the product was obtained (relative density 1.08, pH 5.95, and the content of total flavonoids 8.11%).
Experimental instrument
UPLC (Waters Acquity H-Class), Electronic balance (AB265-S, Mettler-tol), Biological section automation system (Leica, Germany), Multi-funtional Microplate Reader (Tecan M1000, Switzerland), Flow cytometry (Münster, Germany), Fluorescence Microscope (DM400, Germany), MCAO Monofilaments (A4 grade, BEIJING Sunbio Biotech Co., LTD.), Microplate (Thermo Labsystems AC8, Finland).
Experimental animals
Sprague-Dawley rats (180–200 g) were obtained from Experimental Animal Center of PLA Academy of Military Medical Sciences (certification number: SCXK (Army) 2007–2004). All animals were kept in a barrier system with regulated temperature (17–25 °C) and humidity (45–80%) and on a 12 h light/dark cycle. The protocol of the study was approved by the Ethics Committee of the institute of medicinal plant development. The investigation was conducted in accordance with the ethical principles of animal use and care.
Determination of the content of compound A in QNM
Because compound A is the main component of Boschniakia himalaica in QNM, the content of compound A was used as a key indicator to establish the quality standard of QNM. Chromatographic separations were performed on a Diamonsil (200 × 4.6 mm², 5 μm) C18 chromatography column (Waters, USA) using an ACQUITY UPLCTM system. The binary gradient elution system consisted of water (0.1% v/v formic acid) (A) and ACN (B) and separation was achieved using the following gradient: 0–30 min, 10–25% B; 30–40 min, 25–40% B; 50 min, 100% B. The column temperature was kept constant at 35 °C. The flow-rate was 0.8 mL/min and the injection volume was 10 μL.
Establishment of standard curve: Stock solutions (1.01 mg/mL) of Compound A was prepared in methanol in volumetric flasks. Standard working solutions of Compound A (101.0, 151.5, 252.5, 353.5, 454.5, 606.0 μg/mL) were obtained by dilution of standard stock solution with methanol. All these standard working solutions were stored at –4 °C before use.
Determination of the content of compound A: To measure the content of compound A in QNM, 0.5 mL of QNM were accurately diluted into 10 mL volume with H2O. The diluted QNM was filtered through 0.22 μm membrane, and 10 μL of the filtered solution were injected with automatic sampler to determine the content of compound A by HPLC.
Protective effect assay of QNM on OGD-induced damage to SH-SY5Y neuronal cells
SH-SY5Y cells in logarithmic growth phase were detached with trypsin. A portion of the cells was inoculated onto 96-well plate at a density of 1.5 × 104 cells/well to measure the cell viability using MTT method, other cells were cultured in 25 cm2 flasks (5 × 105 cells/flask) and were used to detect the activities of C-3 and C-8 and to analysis for apoptosis by flow cytometry. The cells were cultured in DMEM-F12 containing 10% fetal bovine serum at 37 °C in 5% CO2 incubator.
After 24 h of culture, the cells were pretreated with QNM-containing DMEM-F12 for 2 h and washed twice with PBS. They were then incubated with glucose- and serum-free DMEM in nitrogen incubator under hypoxic condition for 1 h. The cell morphology was monitored to confirm the successful establishment of the OGD cell model. The culture medium was then replaced with fresh DMEM-F12 containing 0.5, 1, 5, 10, 50, 100 and 200 mg/mL QNM, respectively, and the cultures were continued at 37 °C in a 5% CO2 + 95% air incubator for another 24 h (Tang et al. Citation2005; Xu et al. Citation2013). The cell viability was then assayed using MTT method as follows: The medium was replaced with serum-free medium containing MTT (0.5 mg/mL). After another 4 h of culture at 37 °C, the MTT-containing medium was removed, and 150 μL DMSO were added into each well to dissolve the formazan product. The plate was then read at 570 nm wavelength for OD values, and the cell viability in each group was calculated using the cell viability of control group as 100%. The doses of QNM with the good cell viability were chosen for the subsequent experiments.
Activity assay of the C-3 and C-8
Cells cultured with QNM at 50, 100 and 200 mg/mL, respectively, after the treatment under OGD were collected, centrifuged at 600 g, 4 °C for 5 min, washed once with PBS, and lysed by incubating in lysis buffer on ice for 15–20 min. The lysed cells were then centrifuged at 16 000 g, 4 °C for 15 min; the supernatants (protein lysates) were transferred to a new centrifuge tube and stored at –80 °C for further use.
To assay the C-3 activity, standard p-nitroanilide (pNA) was serially diluted with 1 × assay buffer into 10, 20, 50, 100 and 200 μM, respectively; 100 μL of each dilution were added into 96-well plates, with 1 × assay buffer as blank control. Each protein lysate (5 μL) was added into an empty well and mixed with 85 μL of 1× assay buffer and 10 μL of C-3 substrate (Ac-DEVD-pNA). The final volume was 100 μL/well. A blank control was also included. The wells were mixed gently to avoid generating bubbles. After 90 min of incubation at 37 °C, the plate was measured with a microplate reader for OD values at 405 nm wavelength.
To assay the activity of C-8, standard pNA was plated as described above for C-3. Each protein lysate (5 μL) was added into an empty well and mixed with 85 μL of 1 assay buffer and 10 μL of C-8 substrate (Ac-IETD-pNA). The final volume was 100 μL/well. A blank control was also included. The wells were mixed gently to avoid generating bubbles. After 90 min of incubation at 37 °C, the plate was measured with a microplate reader for OD values at 405 nm wavelength.
Analysis of cell apoptosis by flow cytometry
Cells in different treatment groups were detached with trypsin, collected by centrifugation. After washing twice in PBS, the cells were resuspended in 500 μL binding buffer and then mixed with 5 μL of Annexin V and 5 μL of propidium iodide (PI). The cells were analysed on flow cytometry within 1 h.
Preventive effect of QNM on cerebral I/R injury in rats
The rats were randomly divided into seven groups as negative control, sham operation, I/R injury, positive control, high dose QNM, medium dose QNM and low dose QNM, with 30 animals in each group. The drug administration and I/R model establishment were performed to finally obtain at least 15 animals in each group.
The positive control group received nimodipine suspension (2.34 mg/100 g body weight); the low, medium and high dose QNM groups were given QNM at 0.27, 0.54 and 1.08 mL/100 g body weight (equivalent to 1, 2 and 4 times of the clinical doses), respectively. The drugs were prepared daily to avoid the impact of freezing on efficacy, and were administered for continuous 28 days. The I/R injury model was induced 1 h after the last drug administration.
Cerebral I/R procedure
Brain I/R injury was induced by a middle cerebral artery occlusion (MCAO). The rats were fasted for 12 h before the operation and anesthetized with 10% chloral hydrate (3.5 mL/kg, i.v.). The rats were fixed on the operation table and disinfected with conventional method. Briefly, the right common carotid artery, external carotid artery (ECA) and internal carotid artery (ICA) were isolated via a jugular midline incision after skin disinfection. The vagus nerve was stripped carefully and ECA was ligated and snipped. Then pterygopalatine artery was ligated forward along with the ICA. Monofilament suture (ϕ0.26 mm) was introduced into the ECA lumen and advanced into the ICA for a distance of about 18 ± 0.5 mm in order to block the origin of the MCA. After 2 h of ischemia, the nylon suture was withdrawn to establish reperfusion. Then the scalp was sutured and disinfected. Sham-operated animals were not exposed to I/R. The body temperature of the rats was maintained at 37.0 ± 0.5 °C during the surgical procedure with heating plate and a desk lamp of 60 W (Chen et al. Citation2014; Lu et al. Citation2010; Lan et al. Citation2014).
QNM effect on the weight ratio of cerebral infarction tissue in I/R rats
Fresh brain tissues were dissected from the animals, frozen in –20 °C refrigerator for 10–15 min, and cut into coronal sections, which was performed using fresh razor blades and a stainless steel brain matrix. The sections were then stained with 1% triphenyltetrazolium chloride (TTC) solution in the dark in 37 °C oven for 30 min. The TTC dye was then discarded, and the sections were incubated in 10% formaldehyde for another 12 h. After removing the water with filter papers, the white- and red-coloured tissues were separately weighed using analytical balance, and the ratio of the white tissue was calculated.
Immunohistochemical staining and analysis of the brain section
The brain tissues were fixed in paraformaldehyde and dehydrated in gradient ethanol and cleared with xylene in a vacuum dehydration apparatus. The tissues were then embedded in paraffin, cut from the top of the temporal lobe (biauricular line 9.4 mm, bregma 0.7 mm) into 3 μm thickness serial coronal sections. Certain sections were stained for hematoxylin and eosin (HE) and observed for pathology.
For immunohistochemical staining, the sections were dewaxed in xylene (10 min, twice), placed sequentially in 100% (5 min, twice), 95% (5 min) and 75% ethanol (5 min), and were finally washed with distilled water. The activity of endogenous peroxidase was blocked by incubating in 3% hydrogen peroxide at 37 °C for 10 min. The sections were then rinsed thrice with PBS at 2 min each time, incubated in EDTA antigen retrieval solution at 95 °C for 15 min, cooled to room temperature naturally, and rinsed thrice with PBS at 2 min each time. The sections were blocked with normal animal serum at 37 °C for 15 min and then incubated with respective anti-Bcl-2 or -Bax monoclonal antibody (l:200 or 1:400) at 4 °C overnight. Second day, the sections were first incubated at 37 °C for 50 min, rinsed with PBS thrice at 2 min each time, then incubated with biotin-labelled secondary antibody at 37 °C for 12 min, and rinsed with PBS thrice at 2 min each time. The sections were then incubated with horseradish peroxidase-conjugated streptavidin solution at 37 °C for 15 min. After washing 3 times with PBS (2 min each time), the sections were subjected to colour development by addition of the DAB solution, dehydrated then, and finally mounted using neutral resin. The results were observed and documented under a microscope, with 3 fields taken in each sample. The IPP 6.0 software was used to statistically analyse the photographs and to count the number of cells positively stained for respective targeting proteins under 400 × magnification.
Detection of the cytokine levels in serum and brain tissue homogenates
The samples were diluted and detected. The levels of CAT, NO, SOD, MDA in the serum and ICAM-1, TNF-α, BDNF, IL-6 in brain tissue homogenate were measured using ELISA (Ya Anda Biotechnology Co. (Beijing, China) following the manufacturer's instructions.
Data analysis
The data were evaluated considering both statistical difference and biological significance. One-sample Kolmogorov-Smirnov test was used to determine whether there was a normal distribution; if yes, the statistical analysis was carried out using single-factor analysis of variance (ANOVA), and SNK-q test was used to compare among multiple groups; if not, the Kruskal-Wallis test was adopted. All statistical tests were two-tailed, with the level of statistical significance set at 5% or p < 0.05.
Results and discussion
Determination of the main component of QNM
The standard curve was first established by injecting 10 μL of each serially-diluted standard solution, and the standard curve of compound A was obtained using linear regression with the concentration of the standard solution C (μg/mL) as X-axis and the peak area as Y-axis. As shown in , the regression equation was y = 8113.77x + 61299.27, and within the concentration range of 101.0 – 606.0 μg/mL, the concentration C and the peak area exhibited a good linear relationship (R2 = 0.9997).
Three samples of QNM oral liquid prepared as above described in the Materials and Methods were injected at 10 μL each sample. The chromatography results are shown in , and the compound A concentrations in the three tested samples are shown in . The average concentration was 5567.8 μg/mL.
Figure 2. Representative chromatograms. (a) HPLC chromatogram of standard solution at the concentration of 252.5 μg/mL; (b) HPLC chromatogram of 20-times diluted QNM mixture.
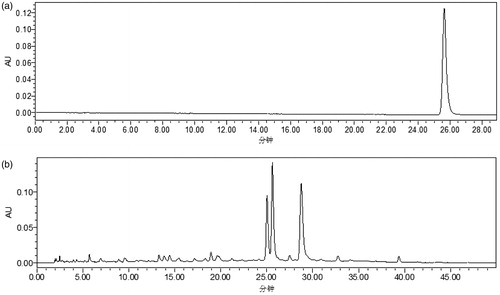
Table 1. The concentration of compound A in three tested QNM samples.
The protective effect of QNM on OGD-induced neuronal injury
In the normal state, SH-SY5Y cells have small rounded cell bodies, short extended axons, small volume of cytoplasm, and dendrite-like processes, and grow in clusters. After 1 h of OGD treatment, the number of cells reduced significantly, and most cells were shrunken, rounded, with reduced or disappeared processes. There was also the occurrence of cell death. These observations demonstrated the successful establishment of OGD model.
As shown in , the rate of cell survival was measured using MTT assay, and the viability of OGD-treated cells was significantly reduced. QNM at 100 and 200 mg/mL significantly improved the cell viability (p < 0.05 or p < 0.01, ). Thus, doses 50, 100 and 200 mg/mL were selected for the subsequent cell protection assay.
Flow cytometry analysis of C-3 and C-8 activity and the apoptotic rate of SH-SY5Y cells
As shown in , the activity of C-3 and C-8 was significantly increased in OGD-treated SH-SY5Y cells, while QNM significantly reduced the activity of C-3 (p < 0.05 or p < 0.01) and C-8 (p < 0.05 or p < 0.01).
Table 2. The effect of QNM on the activities of C-3 and C-8 in OGD-injured SH-5Y5Y cells.
As shown in and , the flow cytometry results indicated that OGD-treated cells displayed evident apoptosis, while QNM at 100 and 200 mg/mL significantly reduced the apoptosis of SH-SY5Y cells (p < 0.05 or p < 0.01), demonstrating the protective effect of QNM on OGD-treated neuronal cells.
Figure 6. The effect of QNM on the apoptosis of OGD-injured SH-SY5Y cells (*p < 0.05, **p < 0.01, compared with OGD group).
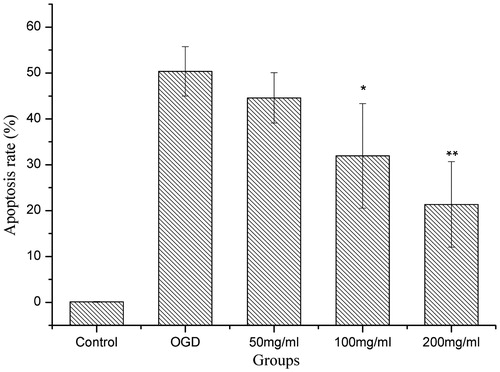
SH-SY5Y cells after 1 h of OGD treatment and 24 h of culture with restored oxygen and glucose showed significantly reduced cell viability, evidently upregulated expression of C-3 and C-8, and significantly elevated cell apoptosis rate.
C-3 and C-8 are apoptosis-specific proteases, which mainly through cleavage block the transport function of plasma membrane Ca2+ pump, resulting in the cytoplasmic or nuclear Ca2+ overload and the activation of Ca2+-Mg2+-dependent endogenous nucleic acid endonuclease, which ultimately leads to the DNA degradation and cell apoptosis. In this study, both C-3 and C-8 were activated after OGD, and the addition of QNM significantly reduced the activity of both enzymes and reduced the rate of apoptosis, indicating that QNM exerts its protective effect on OGD-induced damage to neuronal cells by inhibiting the occurrence of apoptosis via attenuating the activity of apoptotic enzymes C-3 and C-8.
The effect of QNM on the weight ratio of cerebral infarction tissue in I/R rats
As shown in , the weight ratios between the cerebral infarction tissue and the total brain after I/R in high, medium and QNM groups and positive control group were significantly lower than that in I/R injury group (p < 0.05 or p < 0.01), indicating that QNM mixture can significantly improve the status of I/R-induced cerebral injury in rats.
The results of HE staining
As shown in , in sham operation group, the neurons arrayed neatly in layers, with higher numbers of cells, intact morphology, large and evident nuclei (often with prominent nucleoli); there was no gaps between the neurons and the surrounding tissue, but proliferation of a few microglia was observed. In rats of I/R group, the perilesional blood vessels were with dilation and congestion, and there were edema and widened gaps between tissues. The proliferation of microglia was observed in the brain tissue, without significant infiltration of lymphocytes and mononuclear cells. The phenomenon of brain sag was occasionally observed, which might be caused by brain defects resulted from the cerebral liquefaction and necrosis.
Figure 8. The effect of QNM on the histopathology of brain in rats after cerebral I/R injury shown by HE staining. (a) Sham group; (b) I/R injury group; (c) Positive group; (d) Low-dose group; (e) Medium-dose group; and (f) High-dose group. The original magnification was 400×.
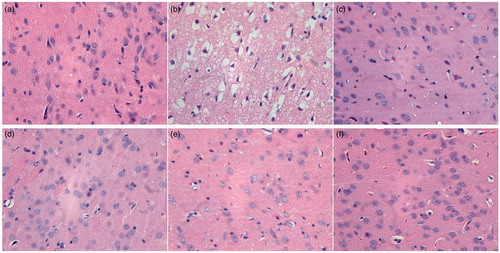
The extent and severity of the pathological changes in positive control group and groups with different dose of QNM were all lower than those in I/R injury group. The lesions in 2 cases of the high-dose QNM group were not evident, and the morphology was closer to that in normal group.
The effect of QNM on the number of Bcl-2/Bax-positive cells in the brain
The ratio of Bcl-2/Bax post I/R in the positive control group and the groups with medium and high dose QNM was significantly upregulated (), which significantly inhibited the cell apoptosis, and the difference was significantly lower than in control group the I/R injury group (p < 0.05).
Detection of the serum cytokine levels
As shown in , all the three doses of QNM significantly increased the serum CAT level in I/R treated rats (p < 0.01), and the medium dose of QNM also increased the serum level of SOD compared to the I/R injury group (p < 0.05). The low and medium doses of QNM significantly inhibited the production of serum MDA post I/R, compared with the I/R injury group (p < 0.05). Certain upregulating effect of QNM on the serum NO was observed too, but with no statistically significant difference.
Detection of the cytokine levels in brain tissue homogenate
As shown in , compared with the I/R injury group, high dose QNM significantly inhibited the expression of ICAM-1 and IL-6 (p < 0.05 or p < 0.01) but increased the expression of BDNF (p < 0.05) in rat brain tissue after I/R, while both medium and high doses of QNM significantly upregulated the expression of NGF in brain tissue after I/R (p < 0.01). Although QNM-treated groups displayed a slightly elevated TNF-α expression after I/R, the difference was not significant compared with the controls, suggesting that QNM has no significant effect on TNF-α expression.
Conclusion
The results of the present study showed that the content of the main component of QNM, compound A, was 5567.8 μg/mL. QNM exerted its protective effect on OGD-induced neuronal injury by inhibiting the cell apoptosis via attenuating the activities of C-3 and caspse-8. The study of rat model of I/R injury demonstrated that QNM provided a good protection against cerebral ischemic injury, the underlying mechanism of which is preliminarily hypothesized as follows: The anti-apoptotic effect was achieved through regulating the ratio of Bcl-2/Bax; QNM significantly upregulated the activities of CAT and SOD, reduced the level of MDA, indicating that QNM is able to mitigate the I/R injury via enhancing the body's ability to scavenge oxygen free radicals; it can also reduce the levels of IL-6 and ICAM-1 after cerebral ischemia, inhibit inflammation-caused secondary damage, and improve the neuronal nutrition via increasing the expression of NGF and BDNF in I/R rats. All these effects of QNM facilitate the brain repair after cerebral I/R injury. Nevertheless, although QNM plays a good protective role against I/R-induced brain damage, relevant studies remain scarce. The biochemical basis of its efficacy and the mechanism of its protective function against cerebral I/R injury thus require further investigations.
Funding information
The project was supported by National Natural Science Foundation of China (No. 81173645), Program for Innovative Research Team in IMPLAD (PIRTI), PUMC Youth Fund (No. 33320140076), Significant New Drugs Creation Special Science and Technology Major of China (2014ZX09201022-006) and Beijing Municipal Natural Science Foundation (No. 2132010).
Disclosure statement
The authors report no conflicts of interest. The authors alone are responsible for the content and writing of this article.
References
- Bao J, Tan Y, Xu M, Du J, Zhao XH, Niu XQ, Pan Y. 2006. Clinical study on Qiancao naomaitong oral liquid for acute ischemic stroke of 90 cases. J Tradit Chin Med. 47:920–922.
- Chen L, Zhao Y, Zhang TL, Dang X, Xie RM, Li ZZ, Li Y, Li YL, Zhao WN, Song HR. 2014. Protective effect of Sheng-Nao-Kang decoction on focal cerebral ischemia-reperfusion injury in rats. J Ethnopharmacol. 151:228–236.
- Jin YP, Zhang GG, Zheng HT, Shang Q, Ouyang J, Du SS. 2008. Chemical constituents of Boschniakia himalaica. Central South Pharmacy. 6:43–45.
- Lakhan SE, Kirchgessner A, Hofer M. 2009. Inflammatory mechanisms in ischemic stroke: therapeutic approaches. J Transl Med. 7:97–107.
- Lan R, Zhang Y, Xiang J, Zhang W, Wang GH, Li WW, Xu LL, Cai DF. 2014. Xiao-Xu-Ming decoction preserves mitochondrial integrity and reduces apoptosis after focal cerebral ischemia and reperfusion via the mitochondrial p53 pathway. J Ethnopharmacol. 151:307–316.
- Lu J, Cheng CM, Zhao XE, Liu QF, Yang P, Wang YM, Luo GA. 2010. PEG-scutellarin prodrugs: synthesis, water solubility and protective effect on cerebral ischemia/reperfusion injury. Eur J Med Chem. 45:1731–1738.
- Mukerji SS, Rainey RN, Rhodes JL, Hall AK. 2009. Delayed Activin A administration attenuates tissue death after transient focal cerebral ischemia and is associated with decreased stress-responsive kinase activation. J Neurochem. 111:1138–1148.
- Park SJ, Nam KW, Lee HJ, Cho EY, Koo U, Mar W. 2009. Neuroprotective effects of an alkaloid-free ethyl acetate extract from the root of Sophora flavescens Ait. against focal cerebral ischemia in rats. Phytomedicine. 16:1042–1051.
- Ritz MF, Ratajczak P, Curin Y, Cam E, Mendelowitsch A, Pinet F, Andriantsitohaina R. 2008. Chronic treatment with red wine polyphenol compounds mediates neuroprotection in a rat model of ischemic cerebral stroke. J Nutr. 138:519–525.
- Tang LL, Wang R, Tang XC. 2005. Huperzine A protects SHSY5Y neuroblastoma cells against oxidative stress damage via nerve growth factor production. Eur J Pharmacol. 519:9–15.
- Wan JF, Yuan JQ, Mei ZN, Yang XZ. 2012. Phenolic glycosides from Boschniakia himalaica. Chinese Chem Lett. 23:579–582.
- Wan JF, Yuan L, Tan NH, Wang Z. 2009. Chemical Constituents of Lysimachia barystachys. Nat Prod Res. 21:966–969.
- Wu PF, Zhang Z, Wang F, Chen JG. 2010. Natural compounds from traditional medicinal herbs in the treatment of cerebral ischemia/reperfusion injury. Acta Pharmacol Sin. 31:1523–1531.
- Xu B, Shan M, Wang F. 2013. Endoplasmic reticulum stress signaling involvement in manganese-induced nerve cell damage in organotypic brain slice cultures. Toxicol Lett. 222:239–246.
- Zhang WN, Luo JG, Kong LY. 2013. Chemical constituents from Boschniakia himalaica. Biochem Syst Ecol. 49:47–50.
- Zhou XQ, Zeng XN, Kong H, Sun XL. 2008. Neuroprotective effects of berberine on stroke models in vitro and in vivo. Neurosci Lett. 447:31–36.
- Zhou ZG, Jin C, Xu C, Qin Y. 2010. Determination of (+)-pinoresinol monoglucoside content in Qiancao naomaitong tablets by HPLC. China Pharmacist. 13:697–698.