Abstract
Context: Amaranthus spinosus Linn. (Amaranthaceae), commonly known as ‘‘spiny pigweed’’, is used in both Indian traditional system and folk medicine for treatment of infectious diseases for a long time in several traditional herbal medicinal preparations. A novel fatty acid [(14E, 18E, 22E, 26E)-methyl nonacosa-14, 18, 22, 26 tetraenoate] is the major metabolite present.
Objective: This study examines the antibacterial potential of the fatty acid isolated from the A. spinosus against some Gram-positive and Gram-negative bacteria.
Materials and methods: Three Gram-positive and seven Gram-negative bacterial strains were used for antibacterial assay. The minimum inhibitory concentration (MIC) of the fatty acid was analysed by dilution method and the effects of the fatty acid on the bacterial membrane were studied in detail by flow cytometry analysis.
Results and discussion: All the studied bacterial strains were found to be inhibited at a concentration of 100 μg/mL. Staphylococcus aureus ML-59, Bacillus lycheniformis 10341, Shigella boydii 8, Vibrio cholera 811, Vibrio cholera 854 and Vibrio alginolyteus were susceptible and sensitive to the tested fatty acid with a MIC value of 25 μg/mL. It proved a full spectrum of antibacterial activity associated with alterations in the permeability of bacterial membranes.
Conclusion: The fatty acid from the A. spinosus possesses potent antibacterial action.
Introduction
Infectious diseases are one of the leading causes of mortality worldwide, especially in developing countries such as India (Dzoyem et al. Citation2010). After regular use of antibiotics in the treatment of infections, many strains of Gram-positive and Gram-negative bacteria have developed drug resistance (Kaushik & Goyal Citation2008). The search for new, safe, nontoxic and effective antibacterial agents is a priority for many researchers. Plants have been used for centuries for the treatment of human diseases as they contain many bioactive components of therapeutic potential (Soberón et al. Citation2010). Traditional system of medicines as an alternative form of health care has led scientists to investigate the antimicrobial activity of medicinal plants (Nostro et al. Citation2000). The present work investigates the antibacterial potential of one of the active metabolites from Amaranthus spinosus stem bark against some Gram-positive and Gram-negative bacteria.
Amaranthus spinosus Linn. (Amaranthaceae) is known as ‘spiny pigweed’, mainly used as a vegetable and cultivated in India, Sri Lanka and other tropical countries (Kirtikar & Basu Citation2001). Traditionally, this plant is used in diabetic complications, also as a diuretic, analgesic, anti-pyretic, or anti-leprotic agent, and in the treatment of bronchitis and piles (Rai et al. Citation2014). The ethnomedicinal uses include, the leaves and roots applied as poultice to relief bruises, abscesses, burns, wound, inflammation, menorrhagia, gonorrhea, eczema and inflammatory swelling (Bulbul et al. Citation2011). Leaves and roots of A. spinosus possess significant antibacterial activity (Maiyo et al. Citation2010; Harsha Citation2011). Active constituents responsible for such antimicrobial activity have not yet been documented. In this regard, we reported the isolation, purification and quantification of a novel fatty acid from A. spinosus in our previous publication (Mondal et al. Citation2015). However, the effect of the purified fatty acid as antibacterial agent was not analysed. Therefore, we examined the antibacterial potency of this isolated fatty acid from A. spinosus against few Gram-positive and Gram-negative strains.
Materials and methods
Chemicals and reagents
All media components were purchased from HiMedia, India. Propidium iodide (PI) and sodium dodecyl sulfate (SDS) were purchased from Sigma-Aldrich, St. Louis, MO. All other chemicals (analytical grade) were procured from Merck (Bangalore, Karnataka, India) and Thermo Fisher Scientific International, Inc. (Mumbai, Maharashtra, India). Other materials and chemicals were purchased locally.
Plant material, extraction and isolation
The whole plant was collected in the month of June 2011 and was identified by Dr. V. P. Prasad, Botanical Survey of India, Howrah, India. A voucher specimen (no. CNH/18/2011/Tech.II/419) was deposited in the Department of Pharmaceutical Technology, Jadavpur University, Kolkata, India. The collected plant material was washed, shade-dried and then milled to coarse powder by a mechanical grinder for further studies.
The plant material (2 kg) was extracted with methanol at room temperature for 48 h. The extract was filtered and dried under reduced pressure at 45 °C, to afford a crude methanol extract (59.46 g). A part of the methanol extract (50 g) was suspended in water and fractioned successively with chloroform, ethyl acetate and n-hexane. Then the extracts were evaporated under reduced pressure in rotatory evaporator and lyophilized to give residues of the chloroform (7.76 g), ethyl acetate (7.06 g) and n-hexane (20.12 g) fractions. All the fractions were evaluated for antibacterial activity. The chloroform fraction was found to be more potent than the ethyl acetate and n-hexane fraction. Hence, the chloroform fraction was further exploited, which led to the isolation of a fatty acid. The isolated metabolite was characterized as a novel fatty acid [(14E, 18E, 22E, 26E)-methyl nonacosa-14, 18, 22, 26 tetraenoate] () based on spectroscopic (IR, 1H-NMR and MS) data. The detail isolation procedure and its structural elucidation were reported in our previous publication (Mondal et al. Citation2015).
Antibacterial assay
Microorganisms
Three Gram-positive (Staphylococcus aureus ML-59, Staphylococcus aureus 29737, Bacillus lycheniformis 10341), and seven Gram-negative bacterial strains (Escherichia coli K-12 ROW, Shigella sonnei 2, Shigella boydii 8, Vibrio cholera 811, Vibrio cholera 854, Vibrio alginolyteus, Salmonella typhimurium NCTC 74) were used for antimicrobial activity. These bacterial strains were obtained from Department of Pharmaceutical Technology, Jadavpur University, Kolkata, India. All sub-cultured microbes used were preserved in slant agar culture at a temperature of 4 °C.
Bactericidal activity
Determination of minimum inhibitory concentrations
Bacteria were cultured overnight at a temperature of 30 °C. The test samples were dissolved in 5% DMSO. Dilutions were prepared in a 96-well microtiter plates to get final concentrations ranging from 0 to 200 μg/mL (Bajpai et al. Citation2010). Finally, 20 μL of inoculum (107 CFU/mL) was inoculated onto the microplates and the tests were performed in a volume of 200 μL. Plates were incubated at 30 °C for 24 h. Ciprofloxacin was used as a positive control for the tested pathogenic bacterium. The lowest concentrations of the tested samples, which did not show any visual growth after macroscopic evaluation, were determined as MICs (expressed in μg/mL).
Membrane permeabilization study
Flow cytometry analysis of PI incorporated S. aureus cells were performed. Membrane permeabilization after fatty acid treatment was estimated by flow cytometry [FACS instrument (LSR Fortessa, BD Biosciences, USA)]. SDS was used as a standard drug for positive control. Approximately 2 × 104 cells/mL was incubated with fatty acid for 60 min at 37 °C. The samples were centrifuged at 6000×g for 8 min, washed three times with PBS and incubated with PI (5 mg/mL) for 30 min at room temperature in the dark, followed by removal of the unbound dye through extensive washing with PBS. Positive control experiments were carried out in bacterial cells with 0.1% SDS (which disrupts lipids in the bacterial membrane) whereas the negative control was performed using DMSO (without fatty acid). Relative fluorescence intensities within the Q4 region were taken as dead cells.
Results and discussion
Antibacterial activity
The results summarized in reveal the MIC of the isolated fatty acid on the tested microorganisms. It shows that fatty acid was active against all the studied microbial strains. All the bacterial strains were found to be inhibited at a concentration of 100 μg/mL. The stains such as S. aureus ML-59, B. licheniformis 10341, V. cholera 811, V. cholera 854, V. alginolyteus and S. boydii 8 were found to be inhibited at the concentration of 25 μg/mL, whereas the stains such as S. aureus 29737, E. coli K-12 ROW and S. sonnei 2 were inhibited at a concentration of 50 μg/mL.
Table 1. Minimal inhibitory concentration (MIC) of isolated fatty acid from A. spinosus against different bacterial pathogens.
Membrane permeabilization studies of S. aureus ML-59 and E. coli K-12 ROW cells
The effect of the fatty acid was examined on the integrity of bacterial membrane by PI-based flow cytometry analysis. The negative control showed 92% viability of bacterial S. aureus cells without fatty acid treatment (). The addition of fatty acid led to a significant membrane disruption as manifested by the fact that 45% of S. aureus cells were PI-positive in quadrant 4 (Q4) (). Similar observations were found in E. coli membrane integrity also after subsequent treatment with fatty acid in PI-based flow cytometry analysis. Ninety-four percent viability was observed in negative control () with 26% of E. coli cells were PI-positive in quadrant 4 (Q4) after drug treatment (). Cells cultured in the presence of SDS were used as positive controls ( and ).
Figure 2. Membrane permeability analyses of Staphylococcus aureus ML-59 cells by flow-cytometry in terms of propidium iodide incorporation. (A) Control cells without fatty acid treatment. (B) Cells incubated with fatty acid at 37 °C for 60 min. (C) Dead control cells (incubated with 0.1% SDS).
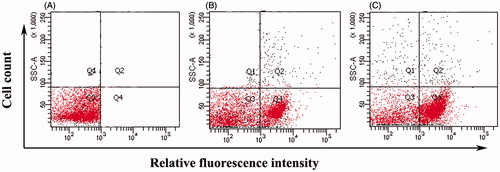
Figure 3. Membrane permeability analyses of Escherichia coli K-12 ROW cells by flow-cytometry in terms of propidium iodide incorporation. (A) Control cells without fatty acid treatment (B) Cells incubated with fatty acid at 37 °C for 60 min. (C) Dead control cells (incubated with 0.1% SDS).
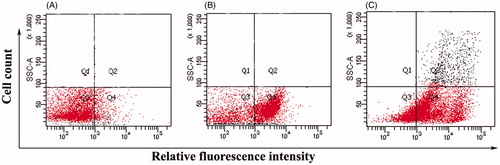
The fatty acid was active against all the pathogenic bacterial strains. The variation observed in the diameters of inhibition zone of the bacteria tested can be attributed to their mechanism of action on Gram-positive and Gram-negative bacteria (De León et al. Citation2005), supported by the cell permeation studies by flow cytometry analysis.
Flow cytometry is a rapid and reliable technique to quantify the viable cells in a cell suspension, which is essential for evaluating the response to active metabolites by the use of the dye exclusion method. Dead cells should be detected in a cell suspension in order to exclude them from the analysis as it can generate artefacts due to nonspecific antibody binding or unwanted uptake of fluorescent probes. Viable cells have intact membranes that exclude a dye PI, whereas it can easily penetrate the damaged, permeable membranes of non-viable cells and binds with double stranded DNA by intercalating between base pairs.
Conclusions
The current result shows the broad spectrum antibacterial potential of fatty acid (14E, 18E, 22E, 26E)-methyl nonacosa-14, 18, 22, 26 tetraenoate, against some bacterial pathogens. The fatty acid obtained from Amaranthus spinosus can potentially be used in the treatment of various infectious diseases caused by bacterial pathogens. The present work lays the foundation for future research, to validate the possible use of fatty acid (14E, 18E, 22E, 26E)-methyl nonacosa-14, 18, 22, 26 tetraenoate as a candidate in the treatment of bacterial infections.
Acknowledgements
We like to thank CSIR-Indian Institute of Chemical Biology, Kolkata, India, for providing instrumental analysis facilities and Department of Pharmaceutical Technology, Jadavpur University, Kolkata, for providing bacterial strains.
Disclosure statement
The authors report no declarations of interest.
References
- Bajpai VK, Dung NT, Suh H, Kang SC. 2010. Antibacterial activity of essential oil and extracts of Cleistocalyx operculatus buds against the bacteria of Xanthomonas spp. J Am Oil Chem Soc. 87:1341–1349.
- Bulbul IJ, Nahar L, Alam Ripa F, Haque O. 2011. Antibacterial, cytotoxic and antioxidant activity of chloroform, n-hexane and ethyl acetate extracts of plant Amaranthus spinosus. Int J Pharm Tech Res. 3:1675–1680.
- De León L, Beltrán B, Moujir L. 2005. Antimicrobial activity of 6-oxophenolic triterpenoids. Mode of action against Bacillus subtilis. Planta Med. 71:313–319.
- Dzoyem J, Pieme C, Penlap V. 2010. In vitro antibacterial activity and acute toxicity studies of aqueous-methanol extract of Sida rhombifolia Linn. (Malvaceae). BMC Complement Altern Med. 10:40
- Harsha VS. 2011. In vitro antibacterial activity of Amaranthus spinosus root extracts. Pharmacophore. 2:266–270.
- Kaushik P, Goyal P. 2008. In vitro evaluation of Datura innoxia (thorn-apple) for potential antibacterial activity. Indian J Microbiol. 48:353–357.
- Kirtikar KR, Basu BD. 2001. Indian medicinal plants, 2nd ed., vol.I. New Connaught Place, Dehradun, Uttranchal: Oriental Enterprises.
- Maiyo ZC, Ngure RM, Matasyoh JC, Chepkorir R. 2010. Phytochemical constituents and antimicrobial activity of leaf extracts of three Amaranthus plant species. Afr J Biotechnol. 9:3178–3182.
- Mondal A, Guria T, Maity TK. 2015. A new ester of fatty acid from a methanol extract of the whole plant of Amaranthus spinosus and its α-glucosidase inhibitory activity. Pharm Biol. 53:600–604.
- Nostro A, Germano M, D’angelo V, Marino A, Cannatelli M. 2000. Extraction methods and bioautography for evaluation of medicinal plant antimicrobial activity. Lett Appl Microbiol. 30:379–384.
- Rai PK, Jindal S, Gupta N, Rana R. 2014. An inside review of Amaranthus spinosus Linn: a potential medicinal plant of India. IJRPC. 4:643–653.
- Soberón J, Sgariglia M, Sampietro D, Quiroga E, Sierra M, Vattuone M. 2010. Purification and identification of antibacterial phenolics from Tripodanthus acutifolius leaves. J Appl Microbiol. 108:1757–1768.