Abstract
Context: Albizia species are reported to exhibit many biological activities including antiovulatory properties in female rats and antispermatogenic and antiandrogenic activities in male rats.
Objective: The present study investigates the flavonoids of Albizia amara (Roxb.) B. Boivin (Fabaceae) leaves and evaluates their activity on gene expression of fertility and antioxidant glutathione-S-transferase-related genes of treated female mice in addition to their effect on DNA damage.
Materials and methods: Plant materials were extracted by using 70% methanol for 48 h, the extract was chromatographed on a polyamide 6S column, each isolated compound was purified by using Sephadex LH-20 column; its structure was elucidated by chemical and spectral methods. Both the leaves extract and myricitrin (200, 30 mg/kg bw/d, respectively) were assayed for their effect on DNA damage in female mice after four weeks treatment using Comet assay. Their modulatory activity on gene expression of fertility (aromatase CYP19 and luteinizing hormone LH) and antioxidant glutathione-S-transferase (GST)-related genes of treated female mice were investigated by real-time PCR (qPCR).
Results: Quercetin-3-O-gentiobioside, myricitrin, quercetin-3-O-α-rhamnopyranoside, myricetin, quercetin and kaempferol were isolated and identified from the studied taxa. Myricitrin and the extract induced low rate of DNA damage (4.8% and 5%, respectively), compared with the untreated control (4.2%) and significantly down-regulated the expression of CYP19 and LH genes and up-regulated GST gene.
Discussion and conclusion: Our results highlight the potential effect of the leaves extract of Albizia amara and myricitrin as fertility-regulating phytoconstituents with ability to protect DNA from damage and cells from oxidative stress.
Introduction
The genus Albizia (Fabaceae) comprises approximately 150 species, mostly trees and shrubs native to tropical and subtropical regions of Asia and Africa (Kokila et al. Citation2013). Albizia lebbeck (L.) Benth. seeds, pods and bark methanol extracts were reported to possess antispermatogenic and antiandrogenic activities in male rats (Gupta et al. Citation2005). Recently, Farag et al. (Citation2013) reported that saponins of A. lebbeck seeds exhibited antiovulatory properties in female rats: the seed extract had an antifertility effect on male rats (Singh et al. Citation1991). Previous phytochemical studies on Albizia species reported the isolation of the flavonoids: 4′,7-dihydroxyflavanone, 3′,4′,7-trihydroxyflavone, and 3-O-methylfisetin from stem bark of A. zygia (DC.) J.F. Macbr. and kaempferol, quercetin, sophoflavescenol and kurarinone from A. lebbeck (Abdalla & Laatsch Citation2012). Julibrosides A1–A3, albiziaflavane and 4′,7-dihydroxyflavan-3,4-diol were isolated by Kamga et al. (Citation2014) from A. ferruginea (Guill. & Perr.) Benth. Albizia amara (Roxb.) B. Boivin known as ‘Oil Cake tree’ is an endemic plant in dry areas of India. Macrocyclic alkaloids (budmunchiamines A, B and C) were isolated from its leaves and found to have antiplatelets aggregation and bactericidal activity (Kokila et al. Citation2013). Previous studies on A. amara leaves revealed its potent antioxidant activities and cytotoxicity on human breast cancer cells (Rajkumar et al. Citation2008), in addition to the regulation of Bcl-2, TNF-α and IL6 genes (Gopinath et al. Citation2013).
The present research aimed to investigate the flavonoids of A. amara leaves and to evaluate the potential effects of the leaves extract and its major isolated compounds from their biological point of view. These include the regulation of fertility (aromatase CYP19 and luteinizing hormone LH) and antioxidant (glutathione-S-transferase, GST)-related genes, in addition to study their effects on DNA damage.
Materials and methods
General
NMR experiments were recorded on Jeol EX-500 spectrometer (JOEL Inc., Tokyo, Japan). EI-MS was measured on Thermo Scientific spectrometer (70 eV) (Thermo Fisher Scientific Inc., Waltham, MA). UV spectra were recorded on Shimadzu model-2401 CP spectrophotometer (Shimadzu Inc., Tokyo, Japan). ESI-MS was measured on LCQ Advantage Thermo Finnigan spectrometer (Thermo Fisher Scientific Inc., Waltham, MA). SSQ Column chromatography (CC) was carried out on Polyamide 6S (Riedel-De-Haen AG, Seelze Haen AG, Seelze Hanver, Germany) and Sephadex LH-20 (Pharmazia, Uppsala, Sweden). Paper chromatography (PC, descending) Whatman No. 1 and 3 mm papers, using solvent systems (1) H2O, (2) 15% HOAc (H2O: HOAc, 85:15), (3) BAW (BuOH:HOAc: H2O, 4:1:5, upper layer) and (4) BBPW (C6H6:n-BuOH:pyridine:H2O, 1:5:3:3, upper layer).
Plant material
Albizia amara leaves were collected from Al Nohoud city, West Kordofan, Sudan, in March 2012 and authenticated by Dr. Sameh Reda. A voucher specimen (M128) was deposited in the herbarium of National Research Centre, Cairo, Egypt (CAIRC). The shade-dried powdered leaves of A. amara (500 g) were extracted by percolation in 70% methanol for 48 h, filtered and the solvent was removed under vacuum to yield 100 g residue (HAE).
Extraction and isolation
The aqueous methanolic extract HAE (90 g) was chromatographed on a polyamide column 6S, 125 × 5 cm internal diameter, and eluted with H2O, followed by H2O–MeOH mixtures of decreasing polarities to yield eight fractions (A–H). Fractions A and B were eluted by (MeOH/H2O, 1:5) and each fraction was subjected to repeated Sephadex LH 20 sub-columns (Pharmazia, Uppsala, Sweden) fractionation using H2O and H2O:MeOH of decreasing polarities to afford the pure compounds 1 (7 mg), 2 (80 mg) and 3 (10 mg). Fractions F and G were eluted from the polyamide column by (MeOH/H2O, 3:2) and (MeOH/H2O, 4:1), respectively. Each fraction was rechromatographed on Sephadex LH 20 column (Pharmazia, Uppsala, Sweden) using MeOH/C6H6/H2O (60:38:2). The subfractions were re-applied on Sephadex LH 20 column (Pharmazia, Uppsala, Sweden) using methanol to yield compounds 4 (15 mg), 5 (12 mg) and 6 (10 mg).
Structure elucidation of the isolated compounds
The isolated compounds were characterized as quercetin 3-O-gentiobioside (1), myricetin 3-O-α-rhamnopyranoside (myricitrin) (2), quercetin 3-O-α-rhamnopyranoside (3), myricetin, (4) quercetin (5) and kampferol (6) for the first time from A. amara leaves. Characterization was carried out by Rf values, colour reactions, acid hydrolysis, UV spectrophotometry, ESI spectrometry and spectral analysis (i.e., 1H nuclear magnetic resonance and 13C nuclear magnetic resonance) and comparing with published data (Agrawal Citation1989; Fossen et al. Citation1999; Kawashty et al. Citation2012).
Quercetin 3-O-gentiobioside 1)
Yellow amorphous powder Rf values: 0.45 (HOAc), 0.34 (BAW); UV λmax,: nm (MeOH): 256, 265sh, 358; (+NaOMe): 268, 327, 403; (+AlCl3): 273, 430; (+AlCl3/HCl): 268, 398; (+NaOAC): 273, 323, 387; (+NaOAC/H3BO3): 262, 377; (CoPC with authentic sample); normal acid hydrolysis gave glucose and quercetin (5) while enzymatic hydrolysis (β-glucosidase) yielded an intermediate: quercetin-3-O-glucoside (CoPC); 1H NMR (500 MHz, DMSOd6): δ 7.7 (1H, d, J = 2.5 Hz, H-2′), 7.4 (1H, dd, J = 2.5, 8.5 Hz, H-6′), 6.78 (1H, d, J = 8.5 Hz, H-5′), 6.3 (1H, d, J = 2.5 Hz, H-8), 6.1 (1H, d, J = 2.5 Hz, H-6), 5.6 (1H, d, J = 8 Hz, H-1″), 4.6 (1H, (d, J = 8 Hz, H-1‴) and 3.2–3.9 (gentiobioside protons overlapped with hydroxyl and water protons).
Myricetin 3-O-α-rhamnopyranoside (myricitrin) (2)
Yellow amorphous powder; Rf values: 0.43 (HOAc), 0.66 (BAW); UV λmax, nm (MeOH): 258, 303, 350; (+NaOMe):267, 395; (+AlCl3): 272, 313, 433; (+AlCl3/HCl): 272, 308sh, 353, 401; (+NaOAC): 275, 315, 375; (+NaOAC/H3BO3): 262, 310, 376; normal acid hydrolysis gave rhamnose and myricetin (4); 1H NMR (500 MHz, DMSOd6): δ 6.9 (2H, s, H-2′, H-6′), 6.3 (1H, d, J = 2.5 Hz, H-8), 6.1 (1H, d, J = 2.5 Hz, H-6), 3.1–4.0 (m, sugar protons) and 0.77 (3H, d, J = 6 Hz, CH3 rhamnose). 13C NMR (125 MHz, DMSO-d6, ppm): 178.1 (C-4), 165.6 (C-7), 161.7 (C-5), 157.8 (C-9), 157.0 (C-2), 146.3 (C-3′, C-5′), 137.0 (C-4′), 134.7 (C-3), 120.1 (C-1´), 108.4 (C-2′, C-6′), 104.2 (C-10), 102.4 (C-1′′), 99.4 (C-6), 94.1 (C-8), 71.8 (C-2′′), 71.0 (C-4′′), 70.9 (C-3′′), 70.4 (C-5′′), 18.0 (C-6′′). ESI/MS [M]− at m/z = 463.
Quercetin 3-O-α-rhamnopyranoside (3)
Yellow amorphous powder; Rf values: 0.39 (HOAc), 0.69 (BAW); UV λmax, nm (MeOH): 259, 304, 354; (+NaOMe): 269, 397; (+AlCl3): 274, 315, 436; (+AlCl3/HCl): 274, 310sh, 355, 404; (+NaOAC): 276, 317, 377; (+NaOAC/H3BO3): 264, 313, 378; 1H-NMR (500 MHz, DMSOd6): δ 7.6 (1H, d, J = 2.5 Hz, H-2′), 7.5 (1H, dd, J = 2.5, 8.5 Hz, H-6′), 6.8 (1H, d, J = 8.5 Hz, H-5′), 6.3 (1H, d, J = 2.5 Hz, H-8), 6.1 (1H, d, J = 2.5 Hz, H-6), 5.7(1H, d, J = 8 Hz, H-1′′), 3.1–3.7 (rhamnopyranoside protons overlapped with hydroxyl and water protons) and 0.8 (3H, d, J = 6 Hz, CH3 rhamnose).
Myricetin (4)
Yellow amorphous powder; Rf values: 0.26 (HOAc), 0.43 (BAW); UV λmax, nm (MeOH): 265, 300sh, 361; (+NaOMe): 269, 328sh, 408; (+AlCl3): 272, 310, 427; (+AlCl3/HCl): 217, 306, 362, 411; (+NaOAC): 270, 401; (+NaOAC/H3BO3): 266, 391; ESI/MS [M]− at m/z = 317.13; 1H NMR (500 MHz, DMSOd6): δ 7.2 (2H, s, H-2′, H-6′), 6.3 (1H, d, J = 2.5 Hz, H-8), 6.1 (1H, d, J = 2.5 Hz, H-6).
Quercetin (5)
Yellow amorphous powder; Rf values: 0.25 (HOAc), 0.80 (BAW); UV λmax, nm (MeOH): 258, 267sh, 298sh, 360; (+NaOMe): 274, 324, 409; (+AlCl3): 275, 304sh, 333sh, 429; (+AlCl3/HCl): 269, 300sh, 358sh, 403; (+NaOAC): 269, 323, 373; (+NaOAC/H3BO3): 261, 301sh, 379; 1H NMR (500 MHz, DMSOd6): δ 7.7 (1H, d, J = 2.5 Hz, H-2′), 7.5 (1H, dd, J = 2.5, 8.5 Hz, H-6′), 6.8 (1H, d, J = 8.5 Hz, H-5′), 6.3 (1H, d, J = 2.5 Hz, H-8), 6.1 (1H, d, J = 2.5 Hz, H-6).
Kaempferol (6)
Yellow amorphous powder, Rf = 0.76 (BAW). UV spectral data, λmax, nm (MeOH): 264, 289sh, 315sh, 363; (+NaOMe), 273, 3216sh, 408; (+AlCl3) 267, 301, 351, 421; (+AlCl3/HCl) 268, 301, 351, 422; (+NaOAc) 271, 304, 376; (+NaOAC/H3BO3) 263, 292, 317, 367.1H NMR (500 MHz, DMSOd6): 8.0 (2H, d, J = 8.5 Hz, H-2′, 6′); 6.9 (2H, d, J = 8.5 Hz, H-3′, 5′); 6.3 (1H, d, J = 2.0 Hz, H-8); 6.1(1H, d, J = 2.0 Hz, H-6).
Biological investigation
Active constituent and plant material administration
Myricitrin (major compound) was administrated orally at a dose of 30 mg/kg bw/d (1/10 of LD50 300 mg) (Pereira et al. Citation2011). Its chemical purity was > 95%, determined by HPLC. The extract HAE was administrated orally at a dose of 200 mg/kg bw/d (1/10 of LD50 2000 mg/kg bw) (Khan et al. Citation2010). The sample doses were dissolved in Tween 80 followed by double-distilled water.
Experimental animals
Thirty female mice weighing 25–30 g were obtained from the Animal House Colony of the National Research Centre, Cairo, Egypt. The animals were kept individually in wire bottomed cages at room temperature (25 ± 2 °C) under 12 h dark–light cycles. They were maintained on standard laboratory diet and water ad libitum. The animals were allowed to acclimatize their new conditions for 1 week before commencing experiment, and then they were allocated into three groups (5 mice/group). All animals received human care in compliance with the Ethics Committee of the National Research Centre, approval no. 13163 and followed the recommendations of the National Institutes of Health Guide for Care and Use of Laboratory Animals No. 85-23 (NIH Citation1985).
Experimental design
The animals were classified as the following for 4-weeks period treatments: group (1) untreated control group (Control); group (2) was treated with myricitrin (30 mg/kg bw/d) and group (3) was treated with HAE (200 mg/kg bw/d).
Sample preparation
At the end of the experimental period, blood samples were withdrawn from the retro-orbital venous plexus under diethyl ether anesthesia. Blood samples were received in EDTA containing tubes and centrifuged at 1800 g for 10 min at 4 °C where plasma was separated and stored at −20 °C for biochemical analyses. After blood collection, the animals were rapidly sacrificed and both the liver and ovaries of each animal were isolated and dried on filter paper, then dissected thoroughly, washed with isotonic saline, and weighed. Immediately, each individual liver and ovaries were stored in liquid nitrogen and stored at −80 °C for gene expression analysis of genes related to fertility and antioxidant effects.
Comet assay
Peripheral blood lymphocytes from female mice were isolated by centrifugation (15 min, 280 g) in a density gradient of Gradisol L (Aqua Medica, Lodz, Poland). The concentration of the cells was adjusted to (1 − 3) × 105 cells/mL by adding RPMI 1640 without glutamine to the single cell suspension. A freshly prepared suspension of cells in 0.75% low-melting point Agarose (Sigma Chemicals, St. Louis, MO) dissolved in phosphate buffer saline (PBS; Sigma Chemicals, St. Louis, MO) was cast onto microscope slides precoated with 0.5% normal melting Agarose. The cells were then lysed for 1 h at 4 °C in a buffer consisting of 2.5M NaCl, 100 m MEDTA, 1% Triton X-100, 10 mM Tris, pH 10. After the lysis, DNA was allowed to unwind for 40 min in electrophoretic solution consisting of 300 mM NaOH, 1 mM EDTA, pH > 13. Electrophoresis was conducted at 4 °C for 30 min at electric field strength 0.73 V/cm (30 mA). The slides were then neutralized with 0.4 M Tris, pH 7.5, stained with 2 μg/mL ethidium bromide (Sigma Chemicals, St. Louis, MO) and covered with cover slips. The slides were examined at 200 × magnification fluorescence microscope (Nikon, Tokyo, Japan) to a COHU 4910 video camera (Cohu Inc., San Diego, CA) equipped with a UV filter block consist an excitation filter (359 nm) and barrier filter (461 nm) and connected to a personal computer-based image analysis system, Lucia-Comet v.4.51 (Laboratory Imaging, Praha, Czech Republic). Fifty images were randomly selected from each sample and the comet tail DNA was measured (Blasiak et al. Citation2004). Endogenous DNA damage measured as the mean comet tail DNA of peripheral blood lymphocytes of two mice groups (5 mice each). The number of cells scored for each animal was 100 () (Blasiak et al. Citation2004).
Table 1. Visual score of DNA damage in female mice treated with of Albizia amara leaves extract and myricitrin using comet assay.
Gene expression analysis
Isolation of total RNA
TRIzol® Reagent (Invitrogen, Karlsruhe, Germany) was used to extract total RNA from liver and ovarian tissues of female mice according to the instructions of the manufacturer. Isolated total RNA was treated with one unit of RQ1 RNAse-free DNAse (Invitrogen, Karlsruhe, Germany) to digest DNA residues, re-suspended in DEPC-treated water and quantified photospectrometrically at 260 nm. Purity of total RNA was assessed by the 260/280 nm ratio which was between 1.8 and 2.1. Additionally, integrity was assured with ethidium bromide-stain analysis of 28S and 18S bands by formaldehyde-containing Agarose gel electrophoresis (data not shown). Aliquots were used immediately for reverse transcription (RT), otherwise they were stored at −80 °C.
Reverse transcription (RT) reaction
Complete poly (A)+ RNA isolated from liver and ovarian tissues was reverse transcribed into cDNA in a total volume of 20 μL using RevertAidTM First Strand cDNA Synthesis Kit (Fermentas, Taufkirchen, Germany). An amount of total RNA (5 μg) was used with a master mix. The master mix was consisted of 50 mM MgCl2, 10× RT buffer (50 mM KCl; 10 mM Tris-HCl; pH 8.3), 10 mM of each dNTP, 50 μM oligo-dT primer, 20 IU ribonuclease inhibitor (50 kDa recombinant enzyme to inhibit RNase activity) and 50 IU Mu LV reverse transcriptase. The mixture of each sample was centrifuged for 30 s at 1000 g and transferred to the thermo cycler. The RT reaction was carried out at 25 °C for 10 min, followed by 1 h at 42 °C, and finished with a denaturation step at 99 °C for 5 min. Afterwards, the reaction tubes containing RT preparations were flash-cooled in an ice chamber until being used for cDNA amplification through quantitative real time-polymerase chain reaction (qRT-PCR).
qRT-PCR
QIAGEN's real-time PCR cycler (Rotor-Gene Q, QIAGEN Inc., Valencia, CA) was used to determine the liver cDNA copy number. PCR reactions were set up in 25 μL reaction mixtures containing 12.5 μL 1 × SYBR® Premix Ex TaqTM (TaKaRa, Biotech. Co. Ltd., Dalian, China), 0.5 μL 0.2 μM sense primer, 0.5 μL 0.2 μM antisense primer, 6.5 μL distilled water and 5 μL of cDNA template. The reaction program was allocated to three steps. First step was at 95.0 °C for 3 min. Second step consisted of 40 cycles in which each cycle is divided into three steps: (a) at 95.0 °C for 15 s, (b) at 55.0 °C for 30 s and (c) at 72.0 °C for 30 s. The third step consisted of 71 cycles which started at 60.0 °C and then increased about 0.5 °C every 10 s up to 95.0 °C. At the end of each sqRT-PCR, a melting curve analysis was performed at 95.0 °C to check the quality of the used primers. Each experiment included a distilled water control.
The semi-quantitative values of RT-PCR (sqRT-PCR) of aromatase CYP19, luteinizing hormone LH and glutathione-S-transferase GST genes were normalized on the bases of β-actin expression () (Girgis et al. Citation2012). At the end of each sqRT-PCR, a melting curve analysis was performed at 95.0 °C to check the quality of the used primers.
Table 2. Primers sequences used.
Calculation of gene expression
First the amplification efficiency (Ef) was calculated from the slope of the standard curve using the following formula found in the manufacturer’s instruction pamphlet:
The relative quantification of the target to the reference was determined by using the 2−ΔΔCT method if Ef for the target (CYP19, LH and GST) and the reference primers (β-actin) as follows:
The relative expression was calculated by 2−ΔΔCT.
Results
Isolation of flavonoids
From the methanolic extract of A. amara leaves (HAE) (MeOH/H2O, 7:3), six flavonoids were isolated, using chromatographic techniques as elaborated in the Supplementary file (page 2). The isolated compounds were identified as quercetin 3-O-gentiobioside (1), myricetin 3-O-α-rhamnopyranoside (myricitrin) (2), quercetin 3-O-α-rhamnopyranoside (3), myricetin (4) quercetin (5) and kaempferol (6) (). Their structure elucidation was carried out through chemical investigation (enzymatic and complete acid hydrolysis) and physical investigation (UV, NMR and MS) (Mabry et al. Citation1970; Agrawal Citation1989; Fossen et al. Citation1999; Markham & Geiger Citation1994; Kawashty et al. Citation2012).
Comet assay
DNA damage of treated female mice with isolated myricitrin (30 mg/kg bw/d) and HAE (200 mg/kg bw/d) was detected by comet assay (). Myricitrin and HAE induced DNA damage rates of 4.8% and 5%, respectively, compared with that of untreated control group 4.2% ().
Gene expression analysis of fertility and antioxidant-related genes
The results obtained from qRT-PCR analysis of CYP19, LH and GST genes isolated from ovarian tissues of treated female mice [myricitrin (30 mg/kg bw/d) and HAE (200 mg/kg bw/d)] are presented in and . Fertility-related genes aromatase CYP19 and luteinizing hormone LH were significantly down-expressed in treated groups with myricitrin and HAE compared with the untreated control group. The expression of antioxidant related gene (glutathione-S-transferase GST) was found to be up-regulated in myricitrin and HAE-treated groups compared with the untreated control group ( and ).
Figure 2. Effect of myricitrin (30 mg/kg bw/d) and Albizia amara hydroalcholic leaves extract (HAE) (200 mg/kg bw/d) on gene expression of luteinizing hormone (LH) and aromatase (CYP19), fertility-related genes isolated from ovarian tissues and glutathione transferase (GST), antioxidant-related gene isolated from liver tissues, of treated female mice. Note: Gene expression analysis was carried out using quantitative real-time PCR analysis. All results were expressed as a,bMeans with different letters, within tissue. One-way analysis of variance (ANOVA) using the Statistical Package for the Social Sciences (SPSS Inc., Chicago, IL) program, version 11 followed by least significant difference (LSD) was used to compare significance between groups. Difference was considered significant when p ≤ 0.05.
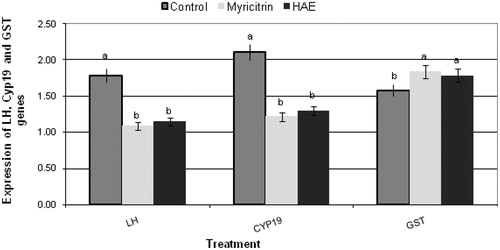
Discussion
In the present study, the isolated flavonoids were separated for the first time from A. amara leaves. Myricitrin (myricetin 3-O-α-rhamnopyranoside) (2) is isolated as a major constituent.
Comet assay revealed that myricitrin and HAE induced low rates of DNA damage, which were relatively similar to that of untreated control group (). However, Gopinath et al. (Citation2013) reported that A. amara effect in the inhibition of DNA damage could be attributed to its potential on p53 and TNF-α genes regulation.
The fertility-related genes aromatase CYP19 and LH were significantly down-regulated in treated female mice by A. amara extract HAE and myricitrin compared with the untreated control group. Regulation of CYP19 and LH genes expression play an important role in male and female reproduction. The aromatase is present in both Leydig cells and seminiferous tubules, predominantly in spermatids in male as well as in the granulosa cells of the oocytes of female. Leydig cell aromatase activity is under luteinizing hormone (LH) control and that paracrine factors of Sertoli cell origin are also involved (Carreau & Levallet Citation2000; Genissel et al. Citation2001). In addition, Gupta et al. (Citation2005) reported that A. lebbeck inhibited the fertility of male rats which suppressed the function of Leydig cells and Sertoli cells and inhibited sperm production. Whereas Paoletta et al. (Citation2008) noted that the aglycone myricetin inhibits the aromatase enzyme. Also, Lu et al. (Citation2012) reported that myricetin inhibited oestrogen biosynthesis by 20%, although it worked via suppressing aromatase transcription rather than inhibiting the enzyme. Further investigation will be necessary to study the structure activity relationship between myricetin, its glycoside (myricitrin) and other derivatives.
Therefore, we can suggest that myricitrin and A. amara leaves could have the ability to modulate the male and female mice fertility due to alteration of aromatase CYP19 and luteinizing hormone LH genes expression.
The expression of antioxidant-related gene (glutathione-S-transferase GST) was found to be up-regulated in myricitrin and HAE-treated groups compared with the untreated control group. This result is in accordance with the reported study of Hayder et al. (Citation2008) on myricitrin anti-mutagenic activity and potential to modulate the expression patterns of other cellular genes involved in oxidative stress and in DNA damaging repair. Consequently, our observations on the protective role of myricitrin and HAE on DNA could be attributed to their potential effect on gene expression and/or its antioxidant activity. Moreover, Pereira et al. (Citation2011) reported that myricitrin is a nitric oxide and protein kinase C inhibitor that exerts anti-psychotic and anti-anxiolytic-like effects. Thus, further deep research needs to be conducted to introduce the bioactive myricitrin and A. amara leaves to the market as non-convential source of fertility regulating herbal drug with less deleterious side effects.
Conclusion
The present study is the first report of the potential of the naturally isolated myricitrin and A. amara leaves extract to induce significant down-regulation of CYP19 and LH genes and up-regulation of GST gene. The study highlights their potential effects as possible fertility-regulating phytoconstituents with the ability to protect DNA from damage and cells from oxidative stress.
Funding information
This work was financially supported by the National Research Center, Egypt, Project no. ‘10010002’.
Disclosure statement
The authors report no declarations of interest.
References
- Abdalla AM, Laatsch H. 2012. Flavonoids from sudanese Albizia zygia (Leguminosae, subfamily Mimosoideae), a plant with antimalarial potency. Afr J Tradit Complement Altern Med. 9:56–58.
- Agrawal PK. 1989. Carbon-13 NMR of flavonoids. New York, Tokyo, Amsterdam, Oxford: Elsevier.
- Blasiak J, Arabski M, Krupa R, Wozniak K, Zadrozny M, Kasznicki J, Zurawska M, Drzewoski J. 2004. DNA damage and repair in type 2 diabetes mellitus. Mutat Res. 554:297–304.
- Carreau S, Levallet J. 2000. Testicular estrogens and male reproduction. News Physiol Sci. 15:195–198.
- Farag M, El Gamal A, Kalil A, Al-Rehaily A, El Mirghany O, El Tahir K. 2013. Evaluation of some biological activities of Albizia lebbeck flowers. Pharmacol Pharm. 4:473–447.
- Fossen T, Larsen A, Kiremire BT, Andersen M. 1999. Flavonoids from blue flowers of Nymphaèa caerulea. Phytochemistry. 51:1133–1137.
- Genissel C, Levallet J, Carreau S. 2001. Regulation of cytochrome P450 aromatase gene expression in adult rat Leydig cells: comparison with estradiol production. J Endocrinol. 168:95–105.
- Girgis E, Khalil WKB, Emam AN, Mohamed MB, Rao KV. 2012. Nanotoxicity of gold and gold-cobalt nanoalloy. Chem Res Toxicol. 25:1086–1098.
- Gopinath P, Sundara VD, Kamatchiammal S, Saroja V. 2013. Anti-cancerous activity of Albizia amara (Roxb.) B. Boivin using human breast cancer cells (MCF-7) by in vitro methods. Int J Pharm Res Rev. 2:23–32.
- Gupta RS, Chaudhary R, Yadav RK, Verma SK, Dobhal MP. 2005. Effect of saponins of Albizia lebbeck (L.) Benth. bark on the reproductive system of male albino rats. J Ethnopharmacol. 96:31–36.
- Hayder N, Bouhlel I, Skandrani I, Kadri M, Steiman R, Guiraud P, Mariotte AM, Ghedira K, Dijoux-Franca MG, Chekir-Ghedira L. 2008. In vitro antioxidant and antigenotoxic potentials of myricetin 3-O-galactoside and myricetin 3-O-rhamnoside from Myrtus communis: modulation of expression of genes involved in cell defense system using cDNA microarray. Toxicol In Vitro. 22:567–581.
- Kamga J, Sandjo LP, Böke-Sarikahya N, Kirmizigül S, Kuete V, Ngadjui BT. 2014. Albiziaflavane A: a new flavane from Albizia ferruginea (Mimosoideae). Nat Prod Res. 28:1574–1578.
- Kawashty SA, Hussein SR, Marzouk MM, Ibrahim LF, Helal MMI, El Negomy SIM. 2012. Flavonoid constituents from Morettia philaena (Del.) DC and their antimicrobial activity. J Appl Sci Res. 8:1484–1489.
- Khan A, Shah RD, Pallewar S. 2010. Evaluation of anti-inflammatory and analgesic activity of ethanolic extracts of Inula racemosa and Albizia amara. Int J Pharmacogn Phytochem Res. 2:322–327.
- Kokila K, Priyadharashini SD, Sujathal V. 2013. Phytopharmacological properties of Albizia species: a review. Int J Pharmacol Pharm Sci. 5:7370–7372.
- Lu DF, Yang LJ, Wang F, Zhang GL. 2012. Inhibitory effect of luteolin on estrogen biosynthesis in human ovarian granulosa cells by suppression of aromatase (CYP19). J Agric Food Chem. 60:8411–8418.
- Mabry TJ, Markham KR, Thomas MB. 1970. The systematic identification of flavonoids. Berlin: Springer.
- Markham KR, Geiger H. 1994. 1H-NMR of flavonoids and their glycosides in DMSO-d6. In: Harborne JB, editor. The flavonoids: advances in research since 1986. London: Chapman & Hall.
- NIH. 1985. Guide for the Care and Use of Laboratory Animals NIH Publication No.85-23, Revised.
- Paoletta S, Steventon GB, Wildeboer D, Ehrman TM, Hylands PJ, Barlow DJ. 2008. Screening of herbal constituents for aromatase inhibitory activity. Bioorg Med Chem. 16:8466–8470.
- Pereira M, Siba IP, Chioca LR, Correia D, Vital MA, Pizzolatti MG, Santos AR, Andreatini R. 2011. Myricitrin, a nitric oxide and protein kinase C inhibitor, exerts antipsychotic-like effects in animal models. Prog Neuropsychopharmacol Biol Psychiatry. 35:1636–1644.
- Rajkumar T, Satheesh Kumar E, Sinha BN. 2008. Evaluation of antioxidant properties of Albizia amara leave. Afr J Biotechnol. 7:1826–1828.
- Singh YN, Bisht H, Panday D. 1991. Effect of dry seed extract of a medicinal plant Albizzia lebbeck on testicular and epididymal protein profiles of rat. Himalayan. J Environ Zool. 5:94–108.