Abstract
Context: The increased incidence of non-albicans Candida (NAC) resistant to fluconazole (FLZ) makes it necessary to use new therapeutic alternatives. Acca sellowiana (O.berg) Burret (Myrtaceae) is a guava with several proven biological activities. The interaction with fluconazole can be a feasible alternative to overcome this resistance.
Objective: This study evaluates the in vitro antifungal activity of fractions obtained from the lyophilized aqueous extract of the leaves of A. sellowiana against resistant strains of NAC.
Materials and methods: The antifungal activity of the fractions was evaluated at 500 μg/mL by microdilution method. Checkerboard assay was performed to determine the effect of the combination of the F2 fraction and antifungal at concentrations: MIC/4, MIC/2, MIC, MIC × 2 and MIC × 4.
Results: Candida glabrata showed the lowest MIC values (500–3.90 μg/mL) and the F2 active fraction was the most effective. The association of F2 with FLZ showed a strong synergistic effect (FICI ≤ 0.5) against 100% of C. glabrata resistant isolates. Moreover, the F2 active fraction has demonstrated that probably acts in the cell wall of these yeasts. There was no observed acute dermal toxicity of lyophilized aqueous extract of leaves of A. sellowiana on pig ear skin cells.
Discussion and conclusion: The interaction between substances present in the F2 active fraction is possibly responsible for the antifungal activity presented by this fraction. This study is unprecedented and suggests that the combination of F2 active fraction and FLZ might be used as an alternative treatment for mucocutaneus infections caused by C. glabrata resistant.
Introduction
Fungal infections caused by Candida spp. have risen considerably in recent years and represent a crescent global health problem (Gácser et al. Citation2014). The increase of infections like mucosal and systemic candidiasis can be explained by a high number of patients at risk, like as the possibility of these yeasts to invade unharmed tissues (Beshey et al. Citation2013). Patients are receiving chemotherapy; transplant recipients and diabetics are more susceptible to fungal infections because they are with their impaired immunity (Kathiravan et al. Citation2012).
Candida albicans, C. glabrata, C. parapsilosis, C. tropicalis and C. krusei represent 95–97% of all invasive fungal infections caused by yeast of this genus. Species of Candida are the most significant cause of opportunistic fungal infections in worldwide (Barros et al. Citation2013). In their study, Pfaller et al. (Citation2014) found that the emergence of candidemias caused by non-albicans species exceeds C. albicans in most tertiary care medical centres in North America. Antifungal resistance is more prevalent in non-albicans Candida (NAC) species than compared with C. albicans. In part, this may be occurring due to intrinsic resistance of the C. krusei to fluconazole (FLZ) and a greater propensity for development of resistance in species such as C. glabrata (Pfaller et al. Citation2014). The C. glabrata infections are more prevalent in the USA (21%) than elsewhere (8–13%), but globally have a high mortality rate in immunocompromised patients (Rodrigues et al. Citation2014). Some authors relate the use of fluconazole with the selection of resistant isolates of C. glabrata, especially in cancer centers (Quindós Citation2014). A total of 30% of the candidemia reported in Brazilian hospitals due to the abusive use of FLZ at these centres which are related with C. glabrata (Da Costa et al. Citation2014).
Azoles antifungals, such as FLZ, are widely used in the treatment of Candida infections. However, treatment failure due to antifungal resistance has been increasingly reported, particularly in HIV-positive individuals (Ahmad et al. Citation2013). Candida species may develop resistance by increasing the drug efflux, phenotypic changes in site of action of this drug and genomic mutations that minimize the toxic effect of the drug (Srinivasan et al. Citation2014). In reason of the surrounding context, natural compounds derived from plants have been drawing attention from researchers, for use in the formulation like new antifungal (Sardi et al. Citation2013). Recent studies have associated antifungal drugs with natural compounds for obtaining synergistic action against resistant isolates (Liu et al. Citation2014).
Acca sellowiana (O.berg) Burret (Myrtaceae) is a plant native to South America, in regions of subtropical climate as the state of Rio Grande do Sul in Brazil (Ruberto & Tringali Citation2004). Some of its components, such as terpenoids and phenolic compounds, have antifungal activity when evaluated in isolation (Ramos et al. Citation2010). Fresh fruit of A. sellowiana (common names: Feijoa, Pineapple Guava and Guavasteen) is appreciated for its characteristic taste, which is similar to the guava. In addition, there is a wide variety of manufactured products of the fruit, particularly in the region of Australia, in the form of jellies, syrup, liquor and candied fruit (Ruberto & Tringali Citation2004). Previous studies have found that its fruits have lots of terpenes, tannins, saponins and flavonoids (Lapcik et al. Citation2005). Furthermore, acetone extract of the fruits of A. sellowiana possess antibacterial, antioxidant, and anti-cancer activities in vitro (Vuotto et al. Citation2000). Although there are studies assessing the composition and biological activities of fruits of A. sellowiana, little is known about the other parts of the plant such as the leaves.
This study investigates the in vitro antifungal activity of active fractions obtained from the lyophilized aqueous extract of the leaves of A. sellowiana, against isolates of NAC (C. famata, C. glabrata, C. tropicalis, C. parapsilosis and C. krusei) resistant to antifungal agents traditionally used in clinical therapy.
Materials and methods
Plant material
Leaves of Acca sellowiana were collected in Rio Grande do Sul, Brazil, in July 2012. The plant was identified by Dr. Ronaldo A. Wasum (UCS, RS). Voucher specimen was deposited in the herbarium of University of Caxias do Sul (ICN).
Obtaining the active fractions
Initially, the aqueous extract was obtained from the infusion of Acca sellowiana leaves employing boiling water as the solvent. During the infusion, the plant drug and the solvent were kept in contact for 15 min. The lyophilized extract fractionation was performed in normal-phase column chromatography using 230–400 mesh silica gel (Macherey – Nagel, Düren, North Rhine-Westphalia, Germany) with solvents acetone, ethanol (Sigma, St. Louis, MO) and distilled water. The solvent gradient followed in this way: Fraction F1) acetone; Fraction F2) acetone:ethanol (50:50); Fraction F3) ethanol; Fraction F4) ethanol:ethanol and water (70:30); Fraction F5) ethanol and water (70:30); Fraction F6) ethanol and water (70:30):ethanol and water (50:50), and Fraction F7) ethanol and water (50:50). The obtained fractions were taken residue in a rotary evaporator (Fisatom), maintaining the temperature of 45° ± 10 °C. The residues of the fractions were solubilized in RPMI 1640 broth (Gibco, Grand Island, NB) to reach the concentrations 1 mg/mL and 6 mg/mL. After this, aliquots were prepared which were maintained at −15 °C and protected from light.
Analysis of the fractions extracted from the freeze-dried aqueous extract of A. sellowiana by thin-layer chromatography (TLC)
The following reference substances (RS) were used: pyrogallol, gallic acid, catechin, quercetin, vitexin, caffeic acid, kaempferol, myricetin, luteolin, rutin and chlorogenic acid (Sigma, St. Louis, MO). As stationary phase, silica gel G/UV254 TLC plate was used (Macherey – Nagel, Düren, North Rhine-Westphalia, Germany). The mobile phase was toluene, ethyl acetate and formic acid (7:10:3 v–v–v) and 2: ethyl acetate, formic acid and distilled water (18:1:1 v-v-v). All solvents have brand Vetec (Xerém, Rio de Janeiro, Brazil). After migration, the plates were developed with Reagent Natural A (difenilborato amino ethanol 1% in ethanol) and polietineloglicol solution, with subsequent visualization lamp chamber with ultra-violet. After the elution, the spots visualized under UV light and after sprayed 1% sulphuric vanillin–H2SO4 reagent followed by heating at 100 °C (Wagner & Bladt Citation1996).
Analysis of the fractions extracted from the lyophilized aqueous extract of A. sellowiana by high-performance liquid chromatography (HPLC)
For the analysis of the fractions, it consisted of a solvent delivery pump (Model LC-10 ADvp), a variable wavelength UV/VIS detector (Model SPD 10 AVP), a manual injection valve (Rheodyne, Rheodyne, Cotati, CA) with a 20 μL loop and degasser (DGU 14A). Data collection and analyses were performed using CLASS-VPTM System Software (Shimadzu Inc., Tokyo, Japan). A gradient elution was performed on a Thermo Scientific Hypersil C18 column (250 × 4.0 mm i.d., 5 μm particle size) (Thermo Scientific, Waltham, MA). The mobile phase consisted of two different solutions, solution A and solution B. Both solutions consisted of tetrahydrofuran (THF) and sodium dihydrogen phosphate buffer (15.6 g/L) adjusted to pH 3.0 with phosphoric acid, where the proportion of THF and buffer was 5:95 for solution A and 40:60 for solution B. All solutions were degassed and filtered through a 0.45 μm pore size filter (Millipore, Temecula, CA). Separations were effected by a gradient elution program as follows: from 0 to 10 min, solution B followed a linear change from 50 to 100%; from 10 to 15 min, B was isocratic at 100%; from 15 to 16 min, B linearly changed from 100 to 50% and from 16 to 20 min, B was isocratic at 50%. The mobile phase flow rate was 1 mL/min and the injection volume was 20 μL. UV detection was performed at 356 nm.
Chromatographic conditions
The identification and the determination of catechin content in the active fractions of A. sellowiana were carried out using an HPLC system (Shimadzu, Canby, OR) which consisted of a solvent delivery pump model CBA – 20A, a autosampler SIL – 20A, with a 20 μL loop, coupled with photodiode array detector (PDA) SPD. A gradient elution was performed on Phenomenex C18 column 5 μ 100A (250 × 4.6 mm) and Ultra C18 4.6 mm precolumn (Thermo Scientific, Waltham, MA). The mobile phase consisted of two different solutions, 0.05% phosphoric acid (solution A) and acetonitrile (solution B). The phosphoric acid and acetonitrile HPLC grade were obtained from Tedia (Fairfield, CA). Separations were effected by a gradient elution program as follows: 95% A and 5% B in 10 min; 88% A and 12% B in 25 min; 80% A and 20% B in 40 min; 70% A and 30% B in 50 min; 80% A and 20% B in 55 min and finally 95% A and 5% B in 58 min. All solutions were filtered through a 0.45 μm pore size filter and degassed. The mobile phase flow rate was 0.7 mL/min and the injection volume was 20 μL. UV detection was performed at 280 nm. The ambient temperature was maintained at 20° ± 2 °C.
Preparation of reference substances and active fractions
To perform the analysis of the active fractions from A. sellowiana and preliminary identification of the compounds present in the samples, the following RS were employed: gallic acid, catechin, epigallocatechin, epigalocatequinagalato, pyrogallol, caffeic acid, chlorogenic acid, rutin and vitexin. The RS used are Sigma brand (St. Louis, MO). Initially, each of RS and active fractions (F1, F2 and F3) were weighed as 2.5 mg and 25 mg and transferred to 10 mL volumetric flask. The samples were dissolved in a 30:70 mixture (acetonitrile:purified water) and then diluted to a final concentration of 25 μg/mL for SR and 250 μg/mL for active fractions. The samples were filtered through syringe filters Chromafil Xtra – PA 45–25–0.4 μm (Sigma Inc., St. Louis, MO) and placed in vials. For the RS and the peaks detected in the samples, absorption spectrum using the photodiode array was obtained.
Standard curve preparation and samples
A catechin standard curve was prepared at concentrations of 10.0, 20.0, 25.0, 30.0, 40.0 and 50.0 μg/mL, in triplicate. The active fractions were evaluated in A. sellowiana concentration: 1000 μg/mL in triplicate. The samples were solubilized in a mixture 30:70 (acetonitrile:purified water) and analyzed by HPLC using the chromatographic conditions as listed above.
Fungal strains
A total of 19 isolates of NAC were tested in this study: Candida famata (CF RL23), Candida glabrata (CG RL02, CG RL03, CG RL12, CG RL34, CG RL37, CG RL12m, CG RL22, CG RL24, CG RL34m and CG RL37m) C. krusei (CK01), C. parapsilosis (CP RL11m, CP RL13m and CP RL27m), C. tropicalis (CT 17A, CT 57A and CT 94P) and as control strain C. glabrata ATCC 40039. The Candida isolates were analyzed phenotypically by Vitek Yeast Biochemical Card (BioMerieux Vitek – Hazelwood, MO). All isolates are from the mycology collection of yeasts of the Federal University of Rio Grande do Sul, Brazil.
Antifungal compounds
The antifungal agents used were FLZ, amphotericin B (AMB) (Crisália, Itapira, São Paulo, Brazil) and anidulafungin (AND) (Pfizer, New York, NY). The stock solution of FLZ was prepared using sterile distilled water at a concentration of 1024 μg/mL. The AMB stock solution was prepared in DMSO (Sigma, St. Louis, MO) at a concentration of 12.8 mg/mL and the AND stock solution was also prepared in DMSO at a concentration of 3.3 mg/mL. For the experiments, antifungal drugs were diluted with RPMI 1640.
Antifungal susceptibility testing
First antifungal screening was carried out to investigate the antifungal activity of the seven fractions from the lyophilized aqueous extract of the leaves of A. sellowiana in the concentration of 500 μg/mL. Fractions that showed antifungal activity in the screening were submitted to the broth microdilution assay to determine the minimum inhibitory concentration (MIC) value with the reading at 100% inhibition. The broth microdilution assay was performed as standardized by the CLSI document M27-A3 (CLSI Citation2008). The MIC value was also determined for drugs antifungals and the results were interpreted through the document M27-S4 (CLSI Citation2012).
Checkerboard assay
The most active fraction from the lyophilized aqueous extract of the leaves of A. sellowiana was used for this assay. The interaction of synthetic antifungal agents (FLZ and AND) with the more active fraction was evaluated in quadruplicate using the checkerboard assay (Johnson et al. Citation2004), with some modifications. This assay was performed in 96-well microplates. The concentrations used for AND, FLZ and more active fraction were selected based on MIC values previously determined. To perform the checkerboard assay, four times lower concentrations and four times greater than the MIC value was tested of each substance for each isolate specifically (MIC/4, MIC/2, MIC, MIC × 2 and MIC × 4). The microplates were incubated at 35 °C for 24 h and 48 h for reading of AND and FLZ, respectively.
After visual reading of the plates, MTT-vital dye tetrazolium salt [3-(4,5-dimethylthiazol-2-yl)-2,5-diphenyltetrazolium bromide] (Sigma, St. Louis, MO) was added, at the concentration 0.5 mg/mL. The plates were incubated for 3 h at 35 °C. Next, the supernatant was removed and the purple formazan crystals formed were extracted of the fungal cells with isopropyl alcohol (Vetec, Xerém, Rio de Janeiro, Brazil). The evaluation of cell damage was measured by reading the absorbance at two wavelengths: 570 and 690 nm in microplate reader Spectramax – M2 (Molecular Devices, Silicon Valley, CA) (Chiou et al. Citation2001). The fractional inhibitory concentration index (FICI) was calculated for each combination. The synergistic action was defined when FICI ≤ 0.5, indifference 0.5 < FICI < 4.0 and antagonism FICI ≥ 4.0 (Odds Citation2003).
Protection of sorbitol
The analysis of the action of the active fractions from A. sellowiana on the integrity of the fungal cell wall was performed for the Sorbitol Protection Assay. In this assay, five isolates of C. glabrata (CG RL02, CG RL03, CG RL12, CG RL12m and CG RL34) and ATCC 40039 strain were used. The determinations of MIC values were conducted simultaneously with and without the addition of sorbitol (Sigma, St. Louis, MO) 1.6 mol/L, an osmotic protection preventing cell lysis. AND was used as a positive control of the test (Escalante et al. Citation2008).
Assay exogenous ergosterol
Exogenous ergosterol (Sigma, St. Louis, MO) in concentrations of 500–100 mg/mL was added to the culture medium. The most active fraction was evaluated to analyze the possible interaction with ergosterol present in the fungal membrane. The assay was carried out for Candida glabrata strains (CG RL12, CG RL34m, CG RL37m and ATCC 40039). The concentration range tested for the active fraction was 500–0.976 μg/mL. AMB (64–0.031 μg/mL) was used as a positive control of the test (Escalante et al. Citation2008).
Scanning electron microscopy
To analyze possible irregularities caused by a more active fraction in the cell walls of yeast, the scanning electron microscope JEOL 6060 (Joel Inc., Peabody, MA) was used. The analyses were performed at the Electron Microscopy Center of the Universidade Federal do Rio Grande do Sul. The microdilution assay was realized and after 48 h, 10 μL of sub-inhibitory concentration (the concentration that inhibits 50% of fungal growth) was withdrawn and incubated at 35 °C overnight. Afterwards, the cells were maintained in a buffer solution 0.1 M sodium cacodylate (pH 7.2) containing 2% glutaraldehyde and 2% paraformaldehyde at 4 °C. The samples were dehydrated in a series of 30–100% acetone solution, dried in a critical point of CO2 (Baltec CPD 030) and coated with gold sputter coater (Moraes et al. Citation2015). This methodology was realized for 10 C. glabrata strains and the same yeast without treatment was also observed.
Histopathological evaluation
Tissue samples from adult male pigs, freshly slaughtered at the Institute Federal of Santa Catarina were used to evaluate the formation of tissue damage due to the action of the lyophilized aqueous extract of leaves of A. sellowiana. Pigs were slaughtered according to the rules of the Brazilian Ministry of Agriculture, respecting animal welfare (Brazil Citation2013). The ear skin of pigs was used in this study. The tissues were removed within a period of 5 min after the slaughter. The hairs were carefully removed by an electric trimmer and transported to the laboratory in ice-cold Krebs–Hepes buffer. The skin samples were mounted in Franz diffusion cells (Logan Instrument Corp., Somerset, NJ) with the diffusion area of approximately 2.25 cm2. The epidermal side of the skin was exposed to a PBS buffer solution pH 7.0 (Sigma, St. Louis, MO) (negative control) for 24 h.
The lyophilized aqueous extract of leaves of A. sellowiana was resuspended in a PBS buffer pH 7.0 at a concentration of 1 mg/mL. The epidermal side of the skin then was subjected to extract contact with this solution for 24 h. Fragments of these tissues were harvested, fixed in 10% neutral-buffered formalin, processed routinely and stained with haematoxylin and eosin (HE), and examined under light microscopy. The experiments were carried out in triplicate.
Results
The chromatographic peak of the RS catechin was eluted at 19.7 min. The analysis of F1, F2 and F3 active fraction by HPLC-PDA elucidated the presence of catechin in the three fractions. In the peak of catechin, two major peaks at most active fraction F1 were found. The highest peak found with a retention time of 25.4 min and the second major peak with a retention time of 22.9 min. In the active fraction F2, a major peak probably was observed in 33.3 min which was present in higher concentration compared with the chromatographic peak of catechin in 19.0 min. Beyond of the presence of catechin, the active fraction also contains other F3 majority chromatographic peaks, for example to 35.3 min. Observed results are shown in .
Figure 1. Chromatogram and absorption spectrum of RS catechin and F1, F2 and F3 active fractions in 1000 μg/mL. (a) RS catechin. Retention time: 19.744. (b) F1 active fraction (major peaks 1, 2 and 3) with retention time of peak catechin: 19.699. (c) F2 active fraction (major peaks 1 and 2) with retention time of peak catechin: 19.073. (d) F3 active fraction (major peaks 1 and 2) with retention time of peak catechin: 19.764. Analyzed by high performance liquid chromatography coupled to photodiode array detector (PDA). Wavelength: 280 nm. Ultra C18 pre-column 4.6 mm; 5 μ C18 100A column (250 × 4.6 mm). Mobile phase 0.05% phosphoric acid (solution A) and acetonitrile (solution B). Flow rate: 0.7 mL/min. Injection volume: 20 μL.
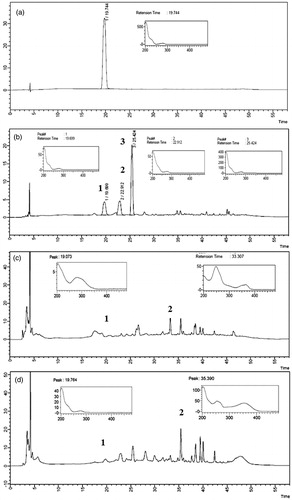
The concentration of catechins present in the active fractions F1, F2 and F3 was determined by a standard curve to obtain the straight-line equation, as shown in .
At 1000 μg/mL, F1 active fraction showed a higher concentration of catechins (7.82 mg/mL), followed by the F2 active fraction (1.38 mg/mL) and F3 active fraction (1.30 mg/mL), as can be seen in .
Table 1. Catechin concentrations present at the F1, F2 and F3 fractions.
The antifungal screening assay demonstrated that the F1, F2, F3, F4 and F6 fractions of A. sellowiana were active for opportunistic yeast.
The MIC determination was carried out for F1, F2, F3, F4 and F6 active fractions against 11 strains of NAC resistant to fluconazole (MIC ≥ 64 μg/mL to C. glabrata and MIC ≥ 8 μg/mL to C. parapsilosis and C. tropicalis). There was no inhibition of fungal growth for the three isolates of C. tropicalis (CT 17A, CT 57A and CT 94P) tested. To C. parapsilosis species, the CP RL11m strain was not sensitive to any of the active fractions and the CP RL13m strain was only sensitive to active fraction F1. Candida glabrata was the most sensitive species to fractions showing the lower MIC values (500–3.90 μg/mL). The F1, F2 and F3 fractions were the most efficient among the active fractions. Results are shown in .
Table 2. Minimum inhibitory concentration (MIC) values expressed in μg/mL of active fractions of A. sellowiana front the strains of non-albicans Candida.
Due to these previous results, our research evaluated the F1, F2 and F3 active fractions against C. glabrata strains: five sensitive strains (CG RL02, CG RL03, CG RL12S, CG RL34S and CG RL37) and five resistant strains (CG RL12m, CG RL22, CG RL24, CG RL34m and CG RL37m) to FLZ. The MIC values were verified at 24 and 48 h. Except CG RL22 strain, the other strains were sensitive to at least two fractions tested, with MIC values between 500 and 3.9 μg/mL. Among the three fractions, the smaller MIC values were found for the F2 active fraction in most strains. F2 active fraction was the most effective against C. glabrata strains, hence this fraction was chosen to implement the proposed methodology ().
Table 3. Minimum inhibitory concentration (MIC) values in 24 and 48 h to F1, F2 and F3 active fractions expressed in μg/mL front of Candida glabrata strains sensitive and resistant to FLZ.
Through the checkerboard assay, the interaction between F2 active fraction with FLZ and AND was rated. Eight out of 10 strains tested showed a synergistic interaction in response to the combination of F2 active fraction with FLZ. The CG RL37m strain showed the highest percentage of cell damage (95%) compared with other strains, reducing four times the MIC values to FLZ and F2 active fraction, and the CG RL22 strain showed the most efficient combination with the lowest IFIC value (0.25), indicating strong synergistic interaction. The FLZ MIC value for CG RL34m strain was 64 μg/mL. When this antifungal was associated with F2 active fraction, the MIC value was reduced to 16 μg/mL, reversing the resistance, as shown in .
Table 4. Evaluation of the interaction between the F2 active fraction and the FLZ against Candida glabrata strains.
The combination of the F2 active fraction with AND showed no synergistic interaction for all the strains that were tested (data not shown).
The percentage of cell damage in the CG RL03, CG RL22 and CG RL37m strains, relating to the four most effective combinations of F2 active fraction and FLZ at different concentrations, is shown in .
Figure 3. (a) CG RL03. (b) CG RL22. (c) CG RL37m. Percentage of cellular damage found in the three most effective combinations resulting from the interaction between the F2 active fraction and fluconazole (F2 + FLZ). Horizontal axis: percentage of cellular damage obtained when the F2 active fraction was evaluated separately in two minimum inhibitory concentrations different. Depth axis: percentage of cellular damage obtained when the fluconazole (FLZ) was assessed separately in two minimum inhibitory concentrations different. Vertical axis: percentage of cellular damage resulting from the interaction between the F2 active fraction with fluconazole (F2 + FLZ) in different minimum inhibitory concentrations.
F2 active fraction

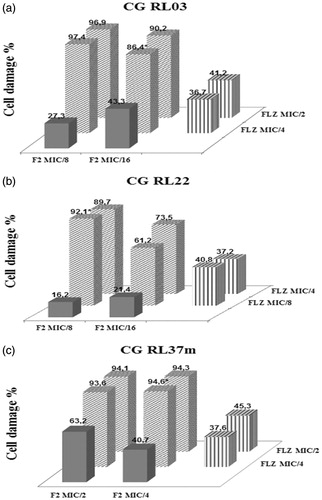
In the exogenous ergosterol test, no differences were observed between the MIC values of the F2 active fraction both in the absence and in the presence of exogenous ergosterol, in all concentrations that were tested (250–50 mg/mL). Thus, the F2 active fraction does not interact with the ergosterol. This fact may indicate that probably the fraction does not act in the membrane of yeast by this mechanism.
An increase in the MIC values of F2 active fraction to all strains tested was observed in the sorbitol protection assay, as well as AND (positive control). The results show that the F2 active fraction F2 possibly act in the cell wall of yeasts.
Supplementary material
The analysis in a scanning electron microscope found that the F2 active fraction exerts its action on the cell wall. Among the six C. glabrata strains tested, all showed deformations in their cell walls, forming cavities on its surface and changes in their morphology. This result corroborates what was observed in our study through the sorbitol protection assay and are shown in .
Figure 4. Morphological representation of C. glabrata strains susceptible to the effect of F2 active fraction through scanning electronic microscopy. (a) CG RL02S (5000 times magnification). (b) CG RL02S (10,000 times magnification). (c) CG RL34m (15,000 times magnification). (d) CG RL12S (10,000 times magnification). (e) CG RL37m (10,000 times magnification). (f) ATCC 40039 in RPMI 1640 (10,000 times magnification). Electric current: 5 kV.
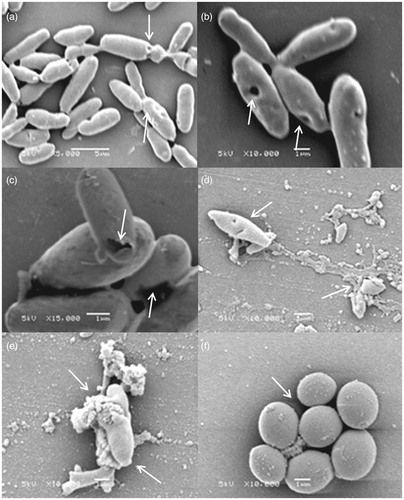
There was observed no acute dermal toxicity acute dermal toxicity of lyophilized aqueous extract of leaves of A. sellowiana on pig ear skin cells by histopathological evaluation () when compared with skin treated with PBS buffer (sample control).
Figure 5. Histopathological evaluation of porcine cells treated with lyophilized aqueous extract of leaves of A. sellowiana and negative control at 100 and 400 times magnification. (1a) Swine epidermal cells treated with lyophilized aqueous extract of leaves of A. sellowiana at 1 mg/mL (100 times magnification). (1b) Swine epidermal cells treated with PBS buffer solution pH 7.0 (100 times magnification). (2a) Swine epidermal cells treated with lyophilized aqueous extract of leaves of A. sellowiana at 1 mg/mL (400 times magnification). (2b) Swine epidermal cells treated with lyophilized aqueous extract of leaves of A. sellowiana at 1 mg/mL (400 times magnification).
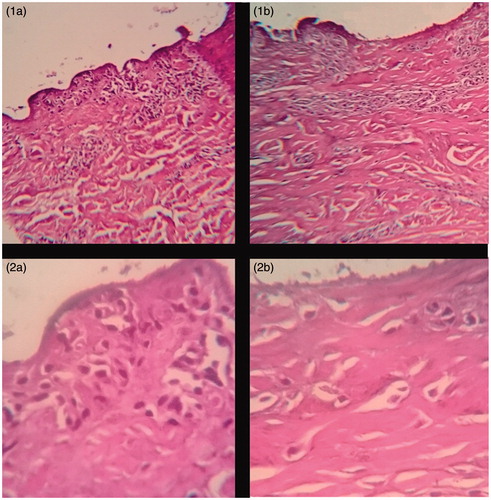
Discussion
Phenolic compounds are secondary metabolites of plants that play a key role in the sensory and nutritional quality of the fruits, vegetables and other plants (Moreno-Montoro et al. Citation2015). Moreover, they consist of a large group of bioactive chemicals having diverse biological functions (Ignat et al. Citation2011). In plants, the phenolic compounds comprise simple phenols, coumarins, lignins, lignans, phenolic acids, flavonoids and condensed and hydrolyzable tannins (Khoddami et al. Citation2013). In recent years, flavonoids have attracted considerable interest due to their potential beneficial effects on human health, such as antiviral, antimicrobial, anti-inflammatory and antioxidant activities, as well as protection against cardiovascular disease and some cancers (Tripoli et al. Citation2007; González-Molina et al. Citation2010; Ahmed et al. Citation2012).
Analyzing the absorption spectrum of the majoritarian chromatographic peaks presents in the F1, F2 and F3 active fractions, we suggest the characteristic of flavonoids and phenolic compounds. The majority chromatographic peak in F2 active fraction (33.3 min) is probably indicative of flavonoids because its UV absorption spectrum presents a characteristic profile of these compounds. The possible presence of flavonoids combined with catechin could explain the higher efficacy of F2 active fraction, when compared with other fractions. The effectiveness of active fractions against the strains tested was not proportional to the concentration of catechin found. The F1 active fraction, which contains the highest concentration of catechin, was less effective in comparison with the F2 active fraction. In the F1 active fraction, other two major peaks are present (peaks 2 and 3) and they were not detected in the F2 active fraction. These substances were not elucidated in this study, but their UV absorption spectrums are characteristic for phenolic compounds derived from catechin. These compounds can probably interact with catechin reducing their potential biological effect. To a lesser extent, compared with the active fraction F2, flavonoid may also be present in the other peaks in the active fraction F3 (peak 3). This peak shows two absorption bands in the UV, the first at approximately 255 nm and the second at about 360 nm. According to Gaitén et al. (Citation2010) flavonoids, especially flavonols and flavones, have two absorption bands in the UV/VIS: band 1 represents the ring A and these compounds are observed in a range of 320–385 nm and band 2 corresponds to ring B, viewed in the 250–285 nm.
According to Saito et al. (Citation2013), catechin inhibited dimorphism of Candida albicans strains by inhibiting the expression of specific genes for the formation of hyphae, reducing the expression levels of the mRNA of the hyphae.
Since the emergence of FLZ during the 1990 decade and its introduction as an antifungal treatment for candidiasis, antifungal therapy has been conducted even with the recognition of the small sensitivity to FLZ and cross-resistance with other azoles. This fact occurs mainly in C. glabrata and C. krusei strains (Pfaller Citation2012). The increased use of antifungal agents for the treatment of mucosal infections and systemic caused by Candida spp. resulted in the selection or emergence of resistant strains (Tobudic et al. Citation2012). Thus, our study searched for to find a new source alternative therapy to combat this antifungal resistance.
The present study showed the unprecedented action of active fractions from the lyophilized aqueous extract of leaves of A. sellowiana against strains of C. parapsilosis, C. famata, C. glabrata and C. krusei. Among these species, C. glabrata was the most sensitive to the fractions, with the lower MIC values. Basile et al. (Citation2010) observed antifungal activity against C. albicans strains (MIC value= 250 μg/mL) and filamentous fungi, however, with the acetone extract of the fruits of A. sellowiana. In a study conducted by Park et al. (Citation2006), it was verified that the epigallocatechin gallate (EGCg), derived from catechins, has lower MIC values that of FLZ and AND for seven Candida species. The C. glabrata species was the most susceptible to EGCg, demonstrating MIC values smaller than FLZ. In our study, C. glabrata was also the most susceptible species to the active fractions containing catechin. Probably the antifungal activity of active fractions obtained from the lyophilized aqueous extract of leaves of A. sellowiana is due to catechin and other compounds that have not been elucidated in these fractions. The F2 active fraction obtained the best antifungal activity possibly due to the interaction between catechin and flavonoids.
Natural products are currently considered an attractive alternative for controlling resistant yeasts. They are effective, most secure and low cost offered to the population (Hirasawa & Takada Citation2004; Rosato et al. Citation2008; Shokri et al. Citation2011; Pippi et al. Citation2015). Research involving synergism with herbal medicines has been established as a fundamental activity in recent years. Synergistic effects of two substances can be produced if the substances affect different targets (Wagner & Ulrich-Merzenich Citation2009).
The evaluation of cellular damage showed that 80% of C. glabrata strains showed synergistic activity to the interaction of the F2 active fraction with FLZ. Moreover, all resistant isolates (CG RL12m, CG RL22, CG RL24, CG RL34m and CG RL37m) demonstrated to be susceptible to this interaction. The combination was also able to reverse the antifungal resistance in four of the five resistant strains to FLZ, except CG RL22 strain. These results show that the combination of F2 active fraction with the FLZ was most effective than the substances separately, since the MIC values of the F2 active fraction and FLZ were lower when measured in the interaction. Thus, antifungals drugs, such as FLZ, can show flaws in their activity when tested alone, but can enhance its action in combination with other substances. A synergistic effect was also observed by Pippi et al. (Citation2015) when the FLZ was associated with Brazilian red propolis against resistant isolates of C. glabrata and C. parapsilosis. Moreover, to Hirasawa and Takada (Citation2004), the combination of epigallocatechin gallate (EGCg) and FLZ has synergistic effect even against resistant strains of C. albicans to FLZ.
For Baddley and Pappas (Citation2005), interactions between substances can result in synergistic effects in three ways: (a) the substance that acts on the cell membrane can increase the penetration of substances acting on the DNA or protein synthesis – AMB combined with azole or flucytosine (5-FC); (b) the interaction of agents that inhibit different steps in the biochemical pathway – azoles and terbinafine; (c) substance with action in the cell wall in association with substance with action on the membrane – echinocandins with azoles or AMB. To Da Silva et al. (Citation2014), the synergistic interaction between flavonoids and FLZ was observed against C. tropicalis strains resistant to FLZ, promoting DNA damage and apoptosis. This information agrees with our study, which the combination of the F2 active fraction with FLZ also reversed the antifungal resistance of C. glabrata strains.
In the interaction between the F2 active fraction and AND, there was no synergistic effect for all isolates tested. An interaction occurs when two compounds used are capable of attacking the yeast cell into more than two different targets (Pfaller et al. Citation2014). The reported indifference between the interaction of the F2 active fraction and the AND probably occurred as both have mechanisms of action similar and, therefore, a mechanism possibly hinders the effect of the other.
Used as a positive control in sorbitol protection assay, the AND inhibits the action of the enzyme β-1,3-d-glucan. This enzyme handles biosynthesis of β-glycan, an essential component in the fungal cell wall, leading to cell death. With the addition of sorbitol to the culture medium, an increase in the MIC values occurs (compared with values without sorbitol) in substances that act on the cell wall. The MIC values of F2 active fraction in the presence of sorbitol increased up to four times. Thus, probably this fraction acts in the cell wall of the strains tested. We can suggest that the active fraction F2, when combined with FLZ, facilitates penetration of the fungal cell membrane, generating a synergistic effect and combat the resistance of these isolates.
Several studies report the antifungal activity of fractions and plant extracts, however, few can observe the action of this effect on cell morphology of yeast. To De Groot et al. (Citation2008), the cell wall of C. glabrata contains 6% protein (glucans and mannans) and 1.3% of chitin having the same basic constituents of the cell wall of C. albicans and Saccharomyces cerevisiae, but in different amounts. The cell wall of C. glabrata is 50% thicker than C. albicans and S. cerevisiae. This difference could hinder the intracellular penetration of antifungal drugs. By scanning electron microscopy, it was also possible to suggest the action of the F2 active fraction on the cell wall of the yeast C. glabrata. These mechanisms are just assumptions because the exact mechanism of action of the F2 active fraction in the cell wall of the strains of C. glabrata is unknown.
The absence of histopathological abnormalities in tissues treated with the lyophilized aqueous extract of leaves of A. sellowiana was expected, due to the popular use of A. sellowiana plant in Australia for the treatment of skin lesions. Different levels of cytotoxicity may be related to biochemical parameters of the cells involved, as the plasma membrane composition and metabolic activity, time of exposure to the toxic agent, toxicity assay used, the medium used to apply the extracts, and also the degree of purification of the extracts (Papo & Shai Citation2005; Maher & McClean Citation2006).
In conclusion, the results presented in this study are very promising. The fractions obtained from the lyophilized aqueous extract of leaves of A. sellowiana showed antifungal activity to NAC yeasts. Strong synergistic activity was observed between the F2 active fraction and FLZ. This combination can be used as an adjunct treatment for eradicate fungal infections caused by C. glabrata resistant. For this, future studies evaluating other pharmacological parameters are required.
Funding information
This study received financial support from the National Council of Technological and Scientific Development (CNPq), Brazil
Acknowledgements
The authors would like to acknowledge the assistance of Gregory Svetlichny for SEM images.
Disclosure statement
All authors declare to have no conflict of interest.
References
- Ahmad A, Khan A, Manzoor N. 2013. Reversal of efflux mediated antifungal resistance underlies synergistic activity of two monoterpenes with fluconazole. Eur J Pharm Sci. 48:80–86.
- Ahmed AS, Elgorashi EE, Moodley N, McGaw LJ, Naidoo V, Eloff JN. 2012. The antimicrobial, antioxidative, anti-inflammatory activity and cytotoxicity of different fractions of four south African Bauhinia species used traditionally to treat diarrhoea. J Ethnopharmacol. 143:826–839.
- Baddley JW, Pappas PG. 2005. Antifungal combination therapy: clinical potential. Drugs. 65:1461–1480.
- Barros FMC, Pippi B, Dresch RR, Daubera B, Lucianob SC, Apela MA, Fuentefriab AM, von Pose GL. 2013. Antifungal and antichemotactic activities and quantification of phenolic compounds in lipophilic extracts of Hypericum spp. native to South Brazil. Ind Crop Prod. 44:294–249.
- Basile A, Conte B, Rigano D, Senatore F, Sorbo S. 2010. Antibacterial and antifungal properties of acetonic extract of Feijoa sellowiana fruits and its effect on Helicobacter pylori growth. J Med Food. 13:189–195.
- Beshey BN, Okasha AS, Eldin MEN. 2013. Fluconazole and selective digestive decontamination for prevention of Candida infection in high risk critically ill patients. Alex. J Med. 50:93–98.
- Brasil. Ministério da Agricultura. 2013. Instrução Normativa n° 3/2000. Aprova Regulamento Técnico de Métodos de Insensibilização para Abate Humanitário de Animais de Açougue.
- Chiou CC, Mavrogiorgos N, Tillem E, Hector R, Walsh TJ. 2001. Synergy, pharmacodynamics, and time-sequenced ultrastructural changes of the interaction between nikkomycin Z and the echinocandin FK463 against Aspergillus fumigatus. Antimicrob Agents Chemother. 45:3310–3321.
- CLSI M27-A3. 2008. Reference method for broth dilution antifungal susceptibility testing of yeasts: third informational supplement. Wayne [PA]: Clinical and Laboratory Standards Institute.
- CLSI M27-S4. 2012. Reference method for broth dilution antifungal susceptibility testing of yeasts: fourth informational supplement. Wayne [PA]: Clinical and Laboratory Standards Institute.
- Da Costa VG, Quesada RMB, Abe ATS, Furlaneto-Maia L, Furlaneto MC. 2014. Nosocomial bloodstream Candida infections in a tertiary-care hospital in South Brazil: a 4-year survey. Mycopathologia. 178:243–250.
- Da Silva CR, Neto JBA, Campos RS, Figueiredo NS, Sampaio LS, Magalhães HI, Cavalcanti BC, Gaspar DM, de Andrade GM, Lima IS, et al. 2014. Synergistic effect of the flavonoid catechin, quercetin, or epigallocatechin gallate with fluconazole induces apoptosis in Candida tropicalis resistant to fluconazole. Antimicrob Agents Chemother. 58:1468–1478.
- De Groot PWJ, Kraneveld EA, Yin QY, Dekker HL, Gross U, Crielaard W, de Koster CG, Bader O, Klis FM, Weig M. 2008. The cell wall of the human pathogen Candida glabrata: Differential incorporation of novel adhesin-like wall proteins. Eukaryotic Cell. 7:1951–1964.
- Escalante A, Gattuso M, Perez P, Zacchino S, Seprényi G, Mándi Y. 2008. Evidence for the mechanism of action of the antifungal phytolaccoside B isolated from Phytolacca tetramera Hauman. J Nat Prod. 71:1720–1725.
- Gácser A, Tiszlavicz Z, Németh T, Seprényi G, Mándi Y. 2014. Induction of human defensins by intestinal Caco-2 cells after interactions with opportunistic Candida species. Microbes Infect. 16:80–85.
- Gaitén YIG, Martínez MM, Henriques AT, Alonso GDB. 2010. Flavonoids analysis of a butanol fraction obtained from Phyllanthus orbicularis HBK. Rev Cub Farm. 44:367–373.
- González-Molina E, Domínguez-Perles R, Moreno DA, García-Viguera C. 2010. Natural bioactive compounds of Citrus limon for food and health. J Pharm Biomed Anal. 51:327–345.
- Hirasawa M, Takada K. 2004. Multiple effects of green tea catechin on the antifungal activity of antimycotics against Candida albicans. J Antimicrob Chemother. 53:225–229.
- Ignat I, Volf I, Popa VI. 2011. A critical review of methods for characterisation of polyphenolic compounds in fruits and vegetables. Food Chem. 126:1821–1835.
- Johnson M, Macdougall C, Ostrosky-Zeichner L, Perfect JR, Rex JH. 2004. Combination antifungal therapy. Antimicrob Agents Chemother. 48:693–715.
- Kathiravan MK, Salake AB, Chothe AS, Dudhe PB, Watode RP, Mukta MS, Gadhwe S. 2012. The biology and chemistry of antifungal agents: a review. Bioorg Med Chem. 20:5678–5698.
- Khoddami A, Wilkes MA, Roberts TH. 2013. Techniques for analysis of plant phenolic compounds. Molecules. 18:2328–2375.
- Lapcik O, Klejdus B, Kokoska L, Davidovác M, Afandi K, Kubán V, Hampl R. 2005. Identification of isoflavones in Acca sellowiana and two Psidium species (Myrtaceae). Biochem Sys Ecol. 33:983–992.
- Liu S, Hou Y, Chen X, Gao Y, Li H, Sun S. 2014. Combination of fluconazole with non-antifungal agents: a promising approach to cope with resistant Candida albicans infections and insight into new antifungal agent discovery. Int J Antimicrob Agents. 43:395–402.
- Maher S, McClean S. 2006. Investigation of the cytotoxicity of eukaryotic and prokaryotic antimicrobial peptides in intestinal epithelial cells in vitro. Biochem Pharmacol. 71:1289–98.
- Moraes RC, Dalla Lana AJ, Kaiser S, Ortega GG. 2015. Antifungal activity of Uncaria tomentosa (Willd.) D.C. against resistant non-albicans Candida isolates. Ind Crop Prod. 69:7–14.
- Moreno-Montoro M, Olalla-Herrera M, Gimenez-Martinez R, Navarro-Alarcon M, Rufián-Henares JA. 2015. Phenolic compounds and antioxidant activity of Spanish commercial grape juices. J Food Compos Anal. 38:19–26.
- Odds FC. 2003. Synergy, antagonism, and what the chequerboard puts between them. J Antimicrob Chemother. 52:1
- Papo N, Shai Y. 2005. Host defense peptides as new weapons in cancer treatment. Cell Mol Life Sci. 62:784–790.
- Park BJ, Park JC, Taguchi H, Fukushima K, Hyon SH, Takatori K. 2006. Antifungal susceptibility of epigallocatechin 3-O-gallate (EGCg) on clinical isolates of pathogenic yeasts. Biochem Biophys Res Commun. 347:401–405.
- Pfaller MA. 2012. Antifungal drug resistance: mechanisms, epidemiology, and consequences for treatment. Am J Med. 125:3–13.
- Pfaller MA, Andes DR, Diekema DJ, Horn DL, Reboli AC, Rotstein C, Franks B, Azie NE. 2014. Epidemiology and outcomes of invasive candidiasis due to non-albicans species of Candida in 2,496 patients: data from the prospective antifungal therapy (PATH) registry 2004–2008. PLos One. 9:101510.
- Pippi B, Dalla Lana AJ, Moraes RC, Güez CM, Machado M, de Oliveira LF, Lino von Poser G, Fuentefria AM. 2015. In vitro evaluation of the acquisition of resistance, antifungal activity and synergism of Brazilian red propolis with antifungal drugs on Candida spp. J Appl Microbiol. 118:839–50.
- Quindós G. 2014. Epidemiology of candidaemia and invasive candidiasis. A changing face. Rev Iberoam Micol. 31:42–48.
- Ramos DM, Bortoluzzi RLC, Mantovani A. 2010. Plantas medicinais de um remascente de Floresta Ombrófila Mista Altomontana. Rev Bras de Plantas Med. 12:380–397.
- Rodrigues CF, Silva S, Henriques M. 2014. Candida glabrata: a review of its features and resistance. Eur J Clin Microbiol Infect Dis. 33:673–688.
- Rosato A, Vitali C, Gallo D, Balenzano L, Mallamaci R. 2008. The inhibition of Candida species by selected essential oils and their synergism with amphotericin B. Phytomedicine. 15:635–638.
- Ruberto G, Tringali C. 2004. Secondary metabolites from the leaves of Feijoa sellowiana Berg. Phytochemistry. 65:2947–2951.
- Saito H, Tamura M, Imai K, Ishigami T, Ochiai K. 2013. Catechin inhibits Candida albicans dimorphism by disrupting Cek1 phosphorylation and cAMP synthesis. Microb Pathog. 56:16–20.
- Sardi JCO, Scorzoni L, Bernardi T, Fusco-Almeida AM, Mendes Giannini MJ. 2013. Candida species: current epidemiology, pathogenicity, biofilm formation, natural antifungal products and new therapeutic options. J Med Microbiol. 62:10–24.
- Shokri H, Khosravi AR, Yalfani R. 2011. Antifungal efficacy of propolis against fluconazole-resistant Candida glabrata isolates obtained from women with recurrent vulvovaginal candidiasis. Int J Gynaecol Obstet. 114:158–159.
- Srinivasan A, Lopez-Ribot JL, Ramasubramanian AK. 2014. Overcoming antifungal resistance. Drug Discov Today Technol. 11:65–71.
- Tobudic S, Kratzer C, Presterl E. 2012. Azole-resistant Candida spp. – emerging pathogens? Mycoses. 5:24–32.
- Tripoli E, La Guardia M, Giammanco S, Di Majo D, Giammanco M. 2007. Citrus flavonoids: molecular structure, biological activity and nutritional properties: a review. Food Chem. 104:466–479.
- Vuotto ML, Basile A, Moscatiello V, De Sole P, Castaldo-Cobianchi R, Laghi E, Ielpo MT. 2000. Antimicrobial and antioxidant activities of Feijoa sellowiana fruit. Int J Antimicrob Agents. 13:197–201.
- Wagner H, Bladt S. 1996. Plant drug analysis: a thin layer chromatography atlas, 2nd ed. Berlin: Springer. 384 p.
- Wagner H, Ulrich-Merzenich G. 2009. Synergy research: approaching a new generation of phytopharmaceuticals. Phytomedicine. 16:97–110.