Abstract
Context: Ethnobotanical claims of medicinal plants used in northern Maputaland are limited.
Objectives To establish scientific validity for a selection of the plants used in Maputaland to treat skin diseases.
Materials and methods: Aqueous and dichloromethane–methanol extracts were prepared from 37 plant species which were collected from four rural communities in Maputaland. Antimicrobial screening was performed on extracts against 12 dermatological relevant pathogens using the micro-titre plate dilution assay. Their combined effect was evaluated by determining the sum of the fractional inhibitory concentrations (ΣFICs). Chemical analysis was undertaken using reverse-phase high-performance liquid chromatography (RP-HPLC) and investigated in vitro across excised intact porcine skin using the ILC07 automated system.
Results: The organic extract of Garcinia livingstonei T. Anderson (Clusiaceae) was found to be the most antimicrobially active displaying an average broad-spectrum MIC value of 270 μg/mL. The combination of Sclerocarya birrea (A. Rich.) Hotsch. (Anacardaceae) with Syzygium cordatum Hochst. ex C. Krauss (Myrtaceae) displayed synergistic effects. The four antimicrobially active organic extracts were found to possess mainly anthraquinones, flavonoids, tannins and saponins. The organic extracts of Kigelia africana (Lam.) Benth. (Bignoniaceae) and S. cordatum were found to have more compounds capable of permeating intact skin after 10 min of exposure.
Conclusion: More than 80% of the organic extracts tested displayed a correlation between the antimicrobial efficacy and the reported traditional uses of the plants. Furthermore, the traditional use of topically applied plant preparations is validated as some compounds from the active plants are capable of permeating the skin in vitro.
Introduction
Skin infections are common worldwide and most are self-limiting with low mortality rates. However, more recently, these infections have been responsible for increased morbidity due to their association with diseases such as the human immunodeficiency virus and the acquired immune deficiency syndrome (HIV/AIDS), causing reduced immunity. Consequently, skin and mucosal infections affect more than 90% of HIV-infected individuals (Steenkamp et al. Citation2007). Resistant microbial strains have also played a role in higher morbidity (Quave et al. Citation2008). In developing countries, the prevalence of bacterial and fungal infections ranges from 20 to 80%. Dermatophytes alone affect more than 25% of the population, especially in African countries (Al-Hoqail Citation2012).
The study area, northern Maputaland, is one of the poorest regions in South Africa with 47% of the population not having a formal income (De Wet et al. Citation2013). The majority of the people in this remote area live together in confined dwellings where infectious diseases may be easily spread (York et al. Citation2011). Skin infections are problematic, due to incidences of high HIV infection rates (15.2%) making people more susceptible to a wide range of skin afflictions (Statistic South Africa: Community Survey Citation2007). Lack of proper infrastructure, humidity, heat and unsanitary living conditions facilitate bacterial and fungal skin infections. The majority of the population in northern Maputaland also depend on wood fires for cooking which increases the risks of burns while other wounds are inflicted during wood collection and field cultivation (De Wet et al. Citation2013). In an ethnobotanical study by De Wet et al. (Citation2013) in four rural communities (Mabibi, Mbazwana, Mseleni and Tshongwe) of northern Maputaland, lay people were interviewed regarding the choice of plants used to treat skin diseases. The focus was on plants that grow in and around the immediate vicinity of the homesteads. Forty-seven plant species were documented for 11 skin ailments (abscesses, boils, burns, incisions, pimples, rash, ringworms, shingles, sores, warts and wounds). Eight plant species i.e., Acacia burkei Benth. (Fabaceae), Brachylaena discolor DC. (Asteraceae), Ozoroa engleri R.Fern. & A.Fern (Anacardaceae), Parinari capensis subsp. capensis Harv. (Chrysobalanaceae), Portulacaria afra Jacq. (Portulacaceae), Sida pseudocordifolia Hochr. (Malvaceae), Solanum rigescens Jacq. (Solanaceae) and Drimia delagoensis Baker (Asparagaceae) were documented for the first time in northern Maputaland, as a treatment for skin infections (De Wet et al. Citation2013). The anti-infective role of these eight plant species has not been previously explored. Furthermore, very limited attention has been given to investigate plants used for skin infections against fastidious pathogens such as Propionibacterium acnes, Microsporum acnes and Trichophyton mentagrophytes (Mabona et al. Citation2013), and these aspects have been considered in this study.
In most traditional medicine systems of the world, including African traditional medicine, different plant species are often combined for better efficacy (van Vuuren & Viljoen Citation2011). Several in vitro antimicrobial reports in the South African context have shown the role of synergism where certain plants are used in combination to treat various infectious diseases (van Vuuren & Viljoen Citation2008; Suliman et al. Citation2010; York et al. Citation2012; Zonyane et al. Citation2013). However, combination studies dedicated to validating of South African plant species, used in combination against skin diseases, are limited. In the study by Mabona et al. (Citation2013) on the antimicrobial activity of southern African medicinal plants with dermatological relevance, the selected plant combinations showed antimicrobial activity against a number of skin-associated pathogens. The information on the use of selected plants was, however, limited to that which was found in the readily available literature. In an ethnobotanical survey by De Wet et al. (Citation2013), it was noted that the lay people of Maputaland use different plant combinations for six types of skin diseases (sores, shingles, burns, boils, acnes and ringworm). This study reports on the in vitro efficacy of the interactive profiles of these plant combinations to determine if there is any antimicrobial benefit when combined.
Another aspect to be considered in the scientific evaluation of plants applied topically for skin infections is the preliminary phytochemical investigation of antimicrobially active extracts and whether compounds present in the crude extracts are capable of permeating the skin. This will provide insight on the intended therapeutic effect of such topically applied preparations. Some studies have evaluated the in vitro permeability of certain pharmacological active compounds present in plant extracts (Heard et al. Citation2006; Boonen et al. Citation2010; Park et al. Citation2012). About 48% of the plant species documented by De Wet et al. (Citation2013) are applied topically to treat both superficial and deep skin infections. None of the chemical profiles have been previously investigated prior to and after in vitro exposure to intact porcine skin. This study examines the ability of chemical compounds to permeate intact skin from the most antimicrobial active topically applied plant extracts used by the lay people in Maputaland.
Materials and methods
Plant collection, identification and extraction
Plant samples were collected from northern Maputaland, KwaZulu-Natal, South Africa during June–July 2012. Thirty-seven plants () were considered for the present study based on the availability of the plant material during the ethnobotanical study (De Wet et al. Citation2013). Plant parts used, such as leaves, bark, roots and fruits from the identified plants, were collected from around the vicinity of the homesteads during the interview process with the lay people. Voucher specimens were prepared on site and are housed in the herbarium of the Department of Botany at the University of Zululand, South Africa. The plants were identified and authenticated by Dr. T. C. H. Morstert from the University of Zululand as well as Mkhipheni Ngwenya from South African National Biodiversity Institute (SANBI), Durban. Collected plant materials were separated according to the parts used and dried at room temperature. The dried plants were then ground into a fine powder using a Scientec RSA Hammer mill (Quadro Alloys And Trading Enterprises, Springs, South Africa).
Table 1. Plants from northern Maputuland used for dermatological conditions.
Two types of extracts (aqueous and organic) were prepared from the ground plant material. Organic extracts were prepared by submerging a known quantity (approximately 30 g) of dried, powdered plant material in approximately 100 mL of a 1:1 mixture of dichloromethane and methanol (CH2Cl2: MeOH) (Merck, Darmstadt, Germany). The mixture was agitated to thoroughly mix plant material, left in an incubator at 37 °C for 24 h and filtered thereafter. Extraction was repeated twice to re-extract the remaining plant material by adding a fresh solvent and incubating for another 24 h. Both resulting filtrates were then combined in one vessel and air-dried to evaporate the solvent. Aqueous extracts were prepared by submerging the known amount of powdered plant material (approximately 30 g) in approximately 100 mL warm, sterile distilled water. These were then left at ambient temperature overnight, filtered and frozen at −80 °C before lyophilization. After lyophilization, the extracts were subjected to ultraviolet light overnight to eliminate possible contaminants. All samples were kept in sealed bottles at room temperature until further use.
Antimicrobial assays
Culture preparation
This was performed according to the National Committee for Clinical and Laboratory Standards guidelines (CLSI Citation2012). American Type Culture Collection (ATCC) bacterial and fungal strains associated with skin infections were selected for analysis. The bacterial strains included the Gram-positive Staphylococcus aureus ATCC 25925, methicillin-resistant Staphylococcus aureus (MRSA) ATCC 43300, gentamycin and methicillin-resistant S. aureus (GMRSA) ATCC 33592, S. epidermidis ATCC 2223, P. acnes ATCC 11827, Brevibacterium agri ATCC 51663 and B. linens DSM 20425. Gram-negative strains included Pseudomonas aeruginosa ATCC 27853 and Escherichia coli ATCC 25922. Two dermatophytes (T. mentagrophytes ATCC 9533, M. canis ATCC 36299), and the yeast Candida albicans (ATCC 10231) were included as fungal pathogens. All ATCC strains were obtained from Davies Diagnostics (Johannesburg, South Africa) and the DSM (Deutsche Sammlung von Mikroorganismen) strain was obtained from German Collection of Micro-organisms. Bacterial cultures were grown in Tryptone Soya broth (TSB) (Oxoid, Hampshire, UK) followed by incubation at 37 °C for 24 h. Propionibacterium acnes, a fastidious anaerobe, was grown in Thioglycolate broth and incubated at 37 °C for 7 d under anaerobic conditions using a candle jar. The yeast, C. albicans, was grown in TSB and incubated at 37 °C for 48 h. Dermatophytes, T. mentagrophytes and M. canis were cultured on Sabouraud’s Dextrose agar (Oxoid, Hampshire, UK) and incubated at 35 °C for 7 d under 100% relative humidity.
Minimum inhibitory concentration (MIC) assays
The micro-dilution technique was used to determine the MIC values of the plant extracts. Microtitre plates were aseptically prepared by adding 100 μL of sterile broth into each well. Plant extracts at the starting concentration of 32 mg/mL were transferred to the first row of the microtitre plate. Serial dilutions were performed on plant extracts. To each well, 100 μL of culture was added. Before use, the culture was first diluted in a broth to just turbid (0.5 McFarland standard) and adjusted to 1:100 ratio to give a density of approximately 1 × 106 colony forming units/mL (CFU/mL). Ciprofloxacin at a starting concentration of 10 μg/mL and amphotericin B at a starting concentration of 100 μg/mL were used as positive controls for the bacteria and fungi, respectively. These were included in each assay to confirm antimicrobial susceptibility. Negative controls (sterile media, antimicrobial sensitivity of solvents) were also included in all assays. Plates were sealed with a sterile adhesive sealer and incubated in appropriate conditions. After incubation, 40 μL of the indicator (0.4 mg/mL) iodonitrotetrazolium chloride (INT) was added to each well. The plates were left at room temperature and assessed for the presence of microbial growth after the development of red colour in the culture control columns which appeared after approximately 1–6 h depending on the test pathogen. For Candida albicans, the plates were left at room temperature and the results were read after 24 h. For the dermatophytes, an indicator was added before incubation and results were read after a 4–7 d incubation period. The MIC value was reported as the lowest concentration of the extract that resulted in inhibition of microbial growth, represented by a clear colour in the wells. All assays were undertaken in duplicate, and where variation was observed, a third repeat was undertaken (Eloff Citation1998; CLSI Citation2012).
Combination studies
The antimicrobial interaction of plants used in combination was determined by calculating the sum of the fractional inhibitory concentrations (ΣFIC). For the ΣFIC determination, the plant extracts at a starting concentration of 32 mg/mL were mixed in equal ratios. The MIC values were determined for the plant combinations and the FIC was calculated for each combination. The ΣFIC was calculated by dividing the MIC value of the combination by the MIC value of a particular plant extract singularly. The ΣFIC was then calculated by adding together the two FIC values from each plant extract within the combination. Where more than two plants were combined, the ΣFIC was calculated taking into consideration each plant within the combination. The ΣFIC value was used to determine if the combined plants are synergistic (ΣFIC ≤ 0.5), additive (ΣFIC > 0.5–1.0), non-interactive (ΣFIC >1.0– ≥4.0) or antagonistic (ΣFIC >4.0) (van Vuuren & Viljoen Citation2011).
Varied ratio studies undertaken on selected combinations which showed interesting results were then combined in nine ratios (9:1, 8:2, 7:3, 6:4, 5:5, 4:6, 3:7, 2:8 and 1:9). The MIC value was determined for all combinations and the values were plotted against independent plant MIC values on an isobologram using GraphPad Prism® version 5 software (GraphPad Software Inc., La Jolla, CA). This expressed the interactions of various ratio combinations on an isobole graph. The isobole graph was interpreted by examining the data points of the ratios falling within or on either three of the adjoining lines (the 0.5:0.5 line, the 1.0:1.0 line and the 4.0:4.0 line) of the two axes. Synergistic activity was noted for points falling below or on the 0.5:0.5 line on the isobologram. An additive effect was expressed when ratio points fell between the 0.5:0.5 and the 1.0:1.0 line. Points falling between the 1.0:1.0 and the 4.0:4.0 line expressed the non-interactive interaction, while antagonism was noted for points falling above the 4.0:4.0 line (van Vuuren & Viljoen Citation2011). Positive and negative controls were included in each assay and all assays were undertaken at least in duplicate.
Further analysis of the most antimicrobially active organic extracts of topically applied plants
Preliminary phytochemical analysis
Phytochemical screening was performed on organic extracts of the four antimicrobially active topically applied plants namely G. livingstonei, K. africana, S. birrea and S. cordatum. The chemical tests were conducted using standard qualitative methods as described by Ahumuza and Kirimuhuzya (Citation2011), as well as Bibi et al. (Citation2012) in order to ascertain the presence of alkaloids, anthraquinones, coumarins, flavonoids, tannins, saponins and sterols.
Chemical profiling
The chemical profiling of the crude organic extracts performed for identification purposes utilized reverse-phase high-performance liquid chromatography (RP-HPLC). The analyses were performed utilizing a Flexar Perkin Elmer HPLC system containing a Perkin Elmer binary pump (PerkinElmer Health Sciences, Inc., Shelton, CT), a Flexar LC autosampler and a Flexar LC UV-Vis detector. Analyses were performed prior to and after in vitro permeation experiments across excised porcine skin. The column (Brownlee Spheri-5 RP-18, 5 μ, 100 × 4.6 mm, PerkinElmer Health Sciences, Inc., Shelton, CT) temperature was held constant at 25 °C. The detection wavelength for all the extracts was 260 nm. Plant extracts (32 mg/mL in PBS) were initially filtered through a 0.45 μm filter before chemical analysis. An aliquot (10 μL of the extract) was injected into the chromatographic system and the resultant chromatograms were used to indicate the peak spectrum of chemical compounds. PBS was injected as a solvent control. Analysis was performed using a linear gradient of A (HPLC grade methanol) and B (0.1% (v/v) formic acid in water) at a flow rate of 1 mL/min. The gradient consisted of the following steps: 0–10 min, 1–5% A; 10–15 min, 5–15 A; 15–20 min, 15–20% A; and 20–40 min, 20–60% A. An equilibration time of ∼5 min (1% A) preceded the next run.
Preliminary studies on the in vitro permeation (diffusion) across excised intact porcine skin
The rate of in vitro diffusion of compounds present in the organic plant extracts across excised intact porcine skin was determined using a PermeGear flow-through diffusion system with seven in-line flow-through cells (PermeGear Inc., Hellertown, PA). The excised porcine skin used was obtained from pigs euthanized for other purposes. Tissue strips (4.0 × 1.0 cm) were snap frozen in liquid nitrogen and stored at −196 °C. An ethics waiver for the approval of the use of porcine skin was obtained from the University of the Witwatersrand School of animal, plant and environmental sciences as well as Human Research Ethics Committee. Prior to each experiment, liquid nitrogen frozen skin tissue strips were thawed in PBS for 5 min at room temperature. The thawed skin tissue strips were cut into seven 1 × 1 cm diameter sections. The skin sections were mounted into the donor compartments of a flow-through diffusion system with the stratum corneum facing up in the direction of the donor compartments. Each compartment was then held together with a clamp wedging the skin between the donor and the receiver compartment. The exposed surface area of the skin for diffusion in each flow cell was 0.039 cm2. All air bubbles under the skin were removed and 500 μL of the plant extract with a concentration of 32 mg/mL in PBS was topically applied on the exposed skin surface in the donor compartment. The donor compartments were then covered with parafilm to prevent any evaporation and to keep a constant temperature within the chambers throughout the experiment. The temperature of the system was kept at 37 ± 2 °C using a thermostatic water bath (Haake SC100). Each experiment was run for a total period of 2 h with liquid (containing the diffused compounds from the extracts) collected every 10 min in the ISCO Retriever IV fraction collector. The collected liquid was analyzed with RP-HPLC to determine the number of chemical compounds that were capable of diffusing from the extract across the skin. Phosphate Buffered Saline (pH 7.4) was used as an elution medium. All experiments were performed in sextuple for each extract.
Results and discussion
Antimicrobial activity of individual plants
The antimicrobial efficacies of the organic and aqueous extracts investigated against 12 dermatological relevant pathogens are presented in . As in many other plant-based antimicrobial studies, organic extracts proved to have higher inhibitory activity compared with the aqueous extracts. Even so, of the 41 aqueous extracts investigated, 61.4% demonstrated noticeable activity (<1000 μg/mL) against one or more of the 12 test pathogens, while all the organic extracts were found to be active against at least one or more pathogens. Most (> 80%) of the organic extracts showed a correlation between the antimicrobial efficacy and their reported traditional uses with some plant species displaying broad-spectrum activity with average MIC values <1000 μg/mL.
Table 2. The average MIC values (μg/mL) of aqueous and organic dichloromethane: methanol extracts against 12 selected dermatological relevant pathogens.
The organic extract of G. livingstonei (only mentioned once by the interviewees) exhibited the lowest average MIC value of 270 μg/mL when tested against the 12 test pathogens. Some compounds (amentoflavone and 4′-monomethoxy amentoflavone) isolated from G. livingstonei acetone leaf extract have also been found to display notable antimicrobial activity against E. coli, S. aureus, and P. aeruginosa with MIC values ranging from 8 to 100 μg/mL (Kaikabo et al. Citation2009). Annona senegalensis (Pers., Annonaceae), also cited once, was reported to treat sores by the lay people of rural Maputaland (De Wet et al. Citation2013). Sores can be infected by various bacteria including S. aureus, GMRSA, MRSA, S. epidermidis, P. aeruginosa and sometimes by E. coli. The organic extract of this plant exhibited a broad-spectrum antimicrobial activity with an average MIC value of 330 μg/mL. The plant was effective against MRSA (MIC value of 500 μg/mL), S. epidermidis (MIC value of 250 μg/mL), E. coli (MIC of 500 μg/mL) and P. aeruginosa (MIC of 250 μg/mL), the pathogens most likely to infect sores. Noticeable antimicrobial properties were also observed against B. agri, B. linens, P. acnes, C. albicans, M. canis and T. mentagrophytes (MIC values between 60 and 500 μg/mL). Annona senegalensis has been reported to treat various skin diseases including leprosy (Abbiw Citation1990), wounds (Hutchings et al. Citation1996), dermatitis and other unspecified skin infections (Kisangau et al. Citation2007). Kaurenoic acid isolated from A. senegalensis exhibited some activity against S. aureus (MIC value of 150 μg/mL) while the lipophilic fraction gave noticeable activity against P. aeruginosa with an average MIC value of 40 μg/mL (Okoye et al. Citation2012).
The bark and fruits of K. africana were reported to treat incisions and ringworm (De Wet et al. Citation2013). In addition to being effective against the relevant pathogens M. canis (MIC values of 340 μg/mL and 380 μg/mL, respectively) and T. mentagrophytes (MIC value of 60 μg/mL), the bark and fruit organic extracts were also effective against other pathogens (B. agri, S. epidermidis, P. acnes, P. aeruginosa and C. albicans) displaying MIC values ranging from 10 to 380 μg/mL. These findings may imply that the similarity of activity could be attributed to the presence of similar compounds in both bark and fruits of K. africana. Previous chemical studies indicated the presence of an iridiod, vermonoside which is common in bark and fruits of this plant species (Gabriel & Olubunmi Citation2009; van Wyk et al. Citation2009). Other studies (Mabona et al. Citation2013; Naidoo et al. Citation2013) have reported on the weak antimicrobial activity of K. africana against pathogens associated with skin and sexually transmitted infections.
Even though the bark of S. brachypetala Sond. (Fabaceae) was only mentioned once as a combined treatment with S. birrea for sores, the organic extract of this plant independently, demonstrated noticeable activities against eight pathogens (an average MIC of 650 μg/mL). Hutchings et al. (Citation1996) documented S. brachypetala as a treatment of acne; however, to the best of our knowledge, no other studies have investigated this plant against the acne-associated pathogen P. acnes. Previous studies on S. brachypetala have focused on diarrhoeal pathogens (Mathabe et al. Citation2006; van Vuuren et al. Citation2015). With the exception of S. aureus, no other studies were found that investigated the effectiveness of S. brachypetala against skin pathogens.
Plant species such as A. burkei, B. discolor, O. engleri, P. capensis subsp. capensis, P. afra and S. rigescens which were investigated in this study have only been recently highlighted as a treatment for skin diseases (De Wet et al. Citation2013). The organic extracts of O. engleri and P. capensis subsp. capensis displayed broad-spectrum antimicrobial activities. The leaves and bark of O. engleri were reported to treat sores. Noticeable activity was observed from the organic bark extract against S. aureus (MIC value of 750 μg/mL), S. epidermidis (MIC value of 50 μg/mL), E. coli (MIC value of 190 μg/mL) and P. aeruginosa (MIC value of 380 μg/mL) which are the pathogens associated with sores, thus supporting the traditional use. Previous studies on P. capensis subsp. capensis have shown efficacy against S. aureus (York et al. Citation2012) and has been found to have promising antifungal activity against Cladosporium cucumerinum (Garo et al. Citation1997). Promising antimalarial activity for this species was also observed (Uys et al. Citation2002). Reports on the dermatophytic activity as observed in this study are new findings. Other plant species namely T. elegans Stapf. (Apocynaceae), S. serratuloides DC. (Asteraceae) and S. birrea were the most frequently mentioned treatments for skin ailments by the lay people of northern Maputaland. The antimicrobial properties of S. birrea have been studied previously and the bark extracts have been found to possess antimicrobial activity against various pathogens (McGaw et al. Citation2000; Eloff Citation2001; York et al. Citation2012; Naidoo et al. Citation2013; van Vuuren et al. Citation2015). This plant was reported to treat burns, boils and sores independently as well as used in combination with other plants (De Wet et al. Citation2013). The organic extract displayed noticeable activity against 10 of the 12 tested pathogens (broad-spectrum activity with an average MIC value 370 μg/mL).
Senecio serratuloides was the most cited plant (cited 17 times) by the interviewees as a treatment of skin diseases (De Wet et al. Citation2013). The plant is also well documented for the treatment of various skin diseases including abscesses, boils (van Wyk & Gericke Citation2000), burns, cuts (Pooley Citation2003), skin eruptions due to syphilis (Taylor et al. Citation2003) and STI sores (De Wet et al. Citation2012). Senecio serratuloides, however, displayed mostly poor antimicrobial activity when tested against the skin pathogens. This may imply that this plant may act by inducing other healing properties in dermatological conditions, i.e., it may act as an anti-inflammatory or for pain relief. Similar incidences of poor activity for frequently used plants in the same geographical area have been found in previous studies (York et al. Citation2012; Naidoo et al. Citation2013).
Even though water was the solvent used traditionally by the lay people of northern Maputaland, many of the aqueous extracts exhibited moderate to poor activity. Certain plant species, however, exhibited some activity. Acacia burkei was reported to treat ringworm (De Wet et al. Citation2013) and the aqueous extract showed noticeable activity against the relevant pathogen, T. mentagrophytes (MIC value of 500 μg/mL). The aqueous extract of E. tirucalli L. (Eurphobiaceae) which was reported to treat sores also gave notable activity against GMRSA and MRSA (MIC values of 500 and 250 μg/mL, respectively), which are the pathogens mostly associated with sores, skin and soft tissue infections, hence validating its traditional use. Ozoroa engleri bark also displayed noticeable activity against four pathogens, B. agri, B. linens, P. acnes and T. mentagrophytes giving MIC values of 500, 250, 380 and 250 μg/mL, respectively.
Antimicrobial activity of combined plants
The ΣFIC values for aqueous and organic (dichloromethane: methanol) extract combinations are depicted in . In cases, where no end point for the MIC determination was found either for a combination or for individual plant(s) in a combination, the ΣFIC value could not be calculated. In these cases, tentative interpretations (synergism, additive, non-interactive or antagonistic effect) were given based on the increase or decrease in MIC value for a combination when compared with the MIC values of the individual plants.
Table 3. The ΣFIC of aqueous and organic plant extracts used in combinations to treat skin diseases.
The aqueous and organic extracts of the poly-herbal combination B. discolor, E. tirucalli, S. serratuloides, O. engleri and Hypoxis hemerocallidea Fisch., C.A. Mey. & Avelall. (Hypoxidaceae) interacted synergistically when tested against S. aureus (based on the tentative interpretation). When considering the MIC data of the individual plants brought into this combination (), the antimicrobial activity was mostly moderate towards S. aureus (MIC values ranging from 1000 to >8000 μg/mL) except O. engleri organic extract which displayed 750 μg/mL. Thus, the synergistic effects observed indicated that the activity was enhanced when the plants were combined which supports the use of this combination for the treatment of sores.
The combination of H. hemerocallidea and S. rigescens (organic extract) exhibited the most prominent synergistic effect (ΣFIC value of 0.04) against S. epidermidis when combined in equal ratios. When investigated independently, the organic extract of H. hemerocallidea showed moderate activity (2000 μg/mL) against S. epidermidis while S. rigescens displayed noticeable activity (150 μg/mL). Thus, S. rigescens contributed strongly to the enhanced activity of the combination. H. hemerocallidea is well documented for its use against skin diseases (Hutchings et al. Citation1996; Elgorashi et al. Citation2003; Taylor et al. Citation2003; Drewes et al. Citation2008; van Wyk et al. Citation2009; De Wet et al. Citation2012), while our earlier study (De Wet et al. Citation2013) found that S. rigescens was only recently noted for the first time as treatment of skin diseases. The fact that this plant species demonstrates such good antimicrobial activity, both independently and in combination brings into consideration the importance of validating newly discovered medicinal uses for plant species. When this combination was analyzed further in various ratios against the two pathogens demonstrating synergistic interactions, the isobologram displayed a synergistic profile (all ratio points fell below the 0.5 line) for all organic combinations irrespective of the ratio at which the two plants were combined ().
Figure 1. Isobologram representation of H. hemerocallidea in combination with S. rigescens against S. aureus and S. epidermidis. Where ▪ = organic extracts combinations, ▴ = aqueous extracts combinations, ★ = 1:1 ratio of organic extracts, • = 1:1 ratio of aqueous extracts.
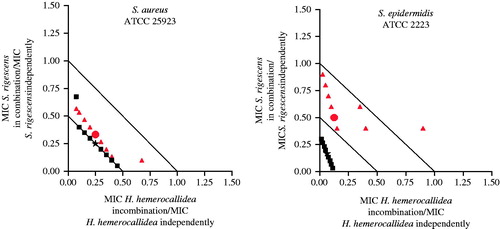
Traditionally, S. birrea is combined with S. cordatum to treat sores (De Wet et al. Citation2013). Mostly, synergistic interactions were evident with the ΣFIC values of 0.13 (aqueous extract combination), 0.34 (organic extract combination) and 0.19 (aqueous extract combination) against S. aureus, S. epidermidis, and P. aeruginosa, respectively. When examined in more depth, i.e., the examination of various ratios (), additive interactions were predominant. Synergistic interactions were observed mostly for the aqueous extract combinations when tested against S. aureus. This is the first report for the evaluation of this combination against skin pathogens. A combination evaluation against pathogens associated with sexually transmitted infections did, however, exhibit noticeable interaction (aqueous extract combination) with the ΣFIC value of 0.42 against the pathogen Oligella ureolytica (Naidoo et al. Citation2013). These findings support the use of this combination in treatment of infectious diseases including skin infections, as claimed by the lay people of northern Maputaland.
Figure 2. Isobologram representation of S. birrea in combination with S. cordatum against S. aureus, S. epidermidis and P. aeruginosa. Where: ▪ = organic extracts combinations, ▴ = aqueous extracts combinations, ★ = 1:1 ratio of organic extracts, • = 1:1 ratio of aqueous. extracts.
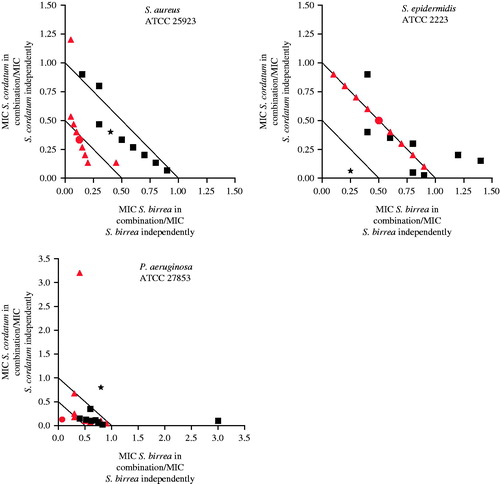
The combined antimicrobial effect of S. brachypetala and S. birrea showed a synergistic interaction against S. epidermidis (organic extract with a ΣFIC value of 0.34) and P. aeruginosa (aqueous extract – tentative interpretation). When examined in various ratios, the interactive profiles of the organic and aqueous extracts of S. brachypetala combined with S. birrea mainly exhibited additive patterns against the tested pathogens (). Even though the initial synergistic interaction was based on a tentative interpretation in the 1:1 combination against P. aeruginosa, the more in-depth analysis shows that where S. brachypetala was in higher concentrations (9:1, 8:2, 7:3 and 6:4), a synergistic effect was observed.
Figure 3. Isobologram representation of S. brachypetala in combination with S. birrea against S. aureus and P. aeruginosa. Where: ▪ = organic extracts combinations, ▴ = aqueous extracts combinations, ★ = 1:1 ratio of organic extracts, • = 1:1 ratio of aqueous extracts.
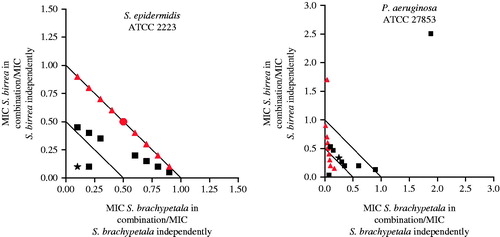
When the combination of Strychnos madagascariensis Poir (Strychnaceae) and Strychnos spinosa Lam. (Strychnaceae) was investigated against S. aureus, S. epidermidis and P. aeruginosa, synergistic interactions were observed for the organic extracts against S. aureus (ΣFIC value of 0.38) and S. epidermidis (ΣFIC value of 0.39). When varying concentrations of the organic extracts of these plants were combined and tested against S. aureus (), most of the ratio points were found in the synergistic region of the isobologram. Since all the ratio points where the concentration of S. madagascariensis was higher fell in a synergy region, it can be deduced that this plant greatly contributed to the observed synergistic effect. When investigated against S. epidermidis, some ratio points (1:9; 3:7 and 4:6 where S. spinosa was higher in concentration) displayed a synergistic effect. One ratio point (9:1) where S. madagascariensis was in higher concentration gave a synergistic effect. Strychnos spinosa has been found to have poor activity when investigated against C. albicans, E. coli, S. aureus and P. aeruginosa (Kubmarawa et al. Citation2007). Strychnos madagascariensis has only been documented for its use against inflammation and wounds (Norscia & Borgognini-Tarli Citation2006) and has only been evaluated against S. aureus and E. coli in a diarrhoeal study by van Vuuren et al. (Citation2015). Studies that have evaluated the antimicrobial efficacy in combination with S. spinosa against skin-associated pathogens are not readily available.
Preliminary phytochemical analysis of the most antimicrobially active organic extracts of topically applied plants
Preliminary qualitative phytochemical screening () revealed the predominant presence of flavonoids, saponins, tannins and anthraquinones in all tested extracts of the selected plants. Flavonoids have been used as main active ingredients in medical preparations (Saravanakumar et al. Citation2009). As a result, flavonoids are the subject of anti-infective research because of their antibacterial, antifungal and antiviral properties. This class of phytochemical contains various compounds that may differ in their modes of action when targeting the microbial cell. For instance, some flavonoid compounds may interfere with nucleic acid synthesis, while others may inhibit the cytoplasmic membrane function and energy metabolism of the microbial cell (Cushnie & Lamb Citation2005).
Table 4. Preliminary phytochemical screening results of the most antimicrobially active topically applied plant extracts (organic).
Saponins are known to protect plants from microbial attack and usually act by disrupting the cell membrane resulting in irreversible damage of the lipid bilayer which account for their antimicrobial activity. This class of phytochemical also exhibits anti-inflammatory properties where they inhibit vascular permeability, the first stage of inflammation (Sparg et al. Citation2004). Anthraquinones isolated from plants also possess antibacterial and antifungal properties (De Barros et al. Citation2011). Tannin containing plants usually possess anti-inflammatory, anti-ulcer and antiparasitic properties. Tannins are also known to inhibit microbial growth by precipitating digestive proteins and thus hindering the availability of nutrients (Prasad et al. Citation2008). These compounds act in the same manner when exposed to burn wounds. They precipitate proteins of the exposed tissue in burn wounds and form granulation tissue.
These four classes of compounds (flavonoids, saponins, tannins and anthraquinones) were all present in the tested extracts and may account for their broad-spectrum antimicrobial activity, as observed in . In addition to the four common classes of compounds found above, G. livingstonei also indicated the presence of alkaloids and flavones, while K. africana indicated the presence of coumarins, flavones and sterols (). Sclerocarya birrea indicated the presence of sterols while S. cordatum indicated the additional presence of alkaloids (). The results obtained in the present study correspond mostly with previous studies on K. africana leaves and fruits (Priya et al. Citation2012; Agyare et al. Citation2013; Priya et al. Citation2013; Arkhipov et al. Citation2014; Solomon et al. Citation2014); S. cordatum bark, leaf and fruit extracts (Pallant & Steenkamp Citation2008; Wanyama et al. Citation2011; Cordier et al. Citation2013; Deliwe & Amabeoku Citation2013; Sidney et al. Citation2015); S. birrea leaf and bark extracts (Ojewole et al. Citation2010; Dabai et al. Citation2013; Baba et al. Citation2014) and G. livingstonei root and stem extracts (Magadula & Suleimani Citation2010; Magadula & Tewtrakul Citation2010).
Chemical profiles of the most antimicrobially active organic extracts of topically applied plants
The HPLC chemical profiles can be utilized to indicate the major peaks present in the various organic extracts for identification purposes as each profile is unique. The profiles of all the extracts were very complex () indicating the presence of a large number of chemical compounds in the extracts. As can be seen from , a large number of relatively hydrophilic compounds as well as a few more lipophilic compounds were present in the K. africana organic extract. Syzygium cordatum organic extract (), however, indicated compounds that were much more lipophilic than those found in the K. africana organic extract. The organic extract of G. livingstonei () similarly indicated a large number of predominantly lipophilic compounds while the organic extract of S. birrea contained various chemical compounds ranging from relatively hydrophilic to more lipophilic compounds (). In the combined organic extract of S. birrea and S. cordatum, a large number of both relatively hydrophilic and lipophilic compounds were observed ().
Figure 5. HPLC chromatograms of K. africana organic extract (32 mg/mL PBS) at 260 nm prior to (A) and after the in vitro permeability experiment at different time intervals (B: 10 min and C: 120 min) after exposure to excised porcine skin.
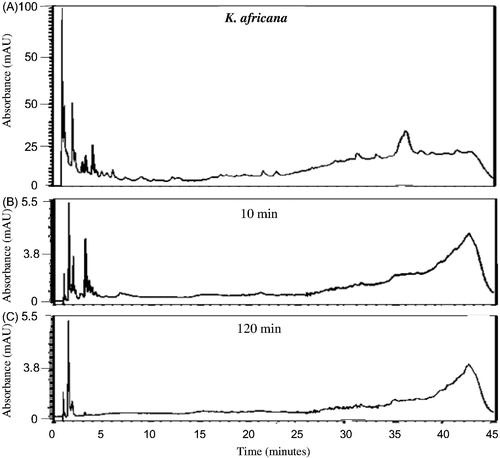
Figure 6. HPLC chromatograms of S. cordatum organic extract (32 mg/mL PBS) at 260 nm prior to (A) and after the in vitro permeability experiment at different time intervals (B: 10 min and C: 120 min) after exposure to excised porcine skin.
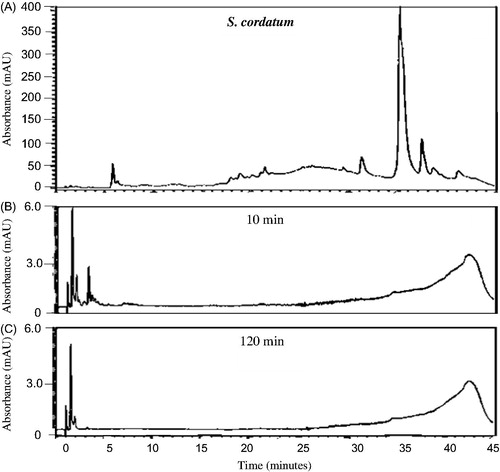
Figure 7. HPLC chromatograms of G. livingstonei organic extract (32 mg/mL PBS) at 260 nm prior to (A) and after the in vitro permeability experiment at different time intervals (B: 10 min and C: 120 min) after exposure to excised porcine skin.
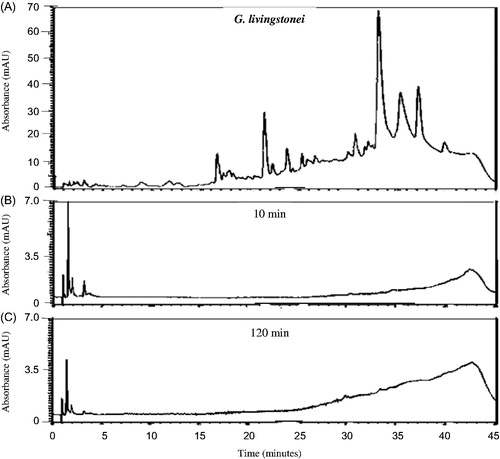
Preliminary studies on the in vitro diffusion of compounds from the most antimicrobially active organic extracts of topically applied plants across excised intact porcine skin
Some plant extracts are topically applied for the treatment of various skin diseases e.g., dermatitis, psoriasis, burns, inflammation and infection. The skin in these diseased states usually contains a compromised barrier function resulting in water loss and altered permeation of topically applied agents (Tsai et al. Citation2001). The active compounds present in topically applied dermatological drugs act on the skin surface mostly for superficial skin infections. They may also act on the epidermal and dermal layers where they penetrate the skin without systemic absorption for ailments affecting epidermal and dermal layers or on the transdermal layer for systemic absorption (Baert et al. Citation2007). These active compounds are, however, usually affected by the permeability of the stratum corneum (Baskar et al. Citation2012; Andrews et al. Citation2013). Thus, in most in vitro studies, the use of biological membrane systems which represent the stratum corneum are employed to study the diffusion profile of the active compounds from topical formulations. This allows the objective comparison of the mode of action to determine whether they serve to act locally, dermally or transdermally on the skin. The same in vitro model (biological membrane system model) was used in this study to evaluate the diffusion of compounds present within the most antimicrobially active organic crude extracts through intact skin to provide a preliminary indication of the movement of these compounds through an intact skin barrier. Since the organic extracts were more active than the aqueous extracts, they were selected for further analysis.
Previous in vitro studies have investigated the permeation behaviour of some known compounds present in topical formulations after certain time intervals with the mean flux values plotted as the standard curves (Heard et al. Citation2006; Boonen et al. Citation2010; Park et al. Citation2012). In the present study, the results were expressed as the preliminary HPLC chemical profile (chemical fingerprint) of each crude extract after in vitro permeation through intact porcine skin. All the investigated organic plant extracts and the combined organic extract of S. birrea and S. cordatum displayed a similar pattern in their penetration behaviour of compounds across the intact skin (). Only relatively hydrophilic compounds eluting within 5 min from the RP-HPLC column were detected after a 10 min exposure time of the intact porcine skin to the various organic extracts. These compounds are assumed to be relatively small. Both K. africana and S. cordatum extracts were found to have slightly more compounds capable of permeating rapidly through the intact porcine skin. Nine major peaks were detected after 10 min of exposure of the intact skin to the extracts, and then decreased in number as the duration of exposure increased to 120 min ( and ). The RP-HPLC chromatograms for the G. livingstonei and S. birrea extracts looked similar to the other two extracts mentioned, however, fewer major compounds diffused across the skin ( and ). In the combined extract of S. birrea and S. cordatum, as expected, a similar pattern of compounds could be detected ().
The permeation behaviour of the compounds from all the extracts indicated that they most probably have a balance between hydrophilicity and lipophilicity and were able to diffuse rapidly through the intact stratum corneum. The predominantly lipophilic compounds observed within the crude extracts ( and ) most probably accumulated within the intact skin due to their size as well as their affinity for the lipophilic stratum corneum. Usually, lipophilicity is among the physicochemical properties that determine the diffusion rate of drug molecules across the skin (Tsai et al. Citation2001). Since the stratum corneum is lipophilic, drug molecules have to be lipophilic to be able to partition within the lipid lamellae of the corneocytes (Naik et al. Citation2000). Permeation through intact skin will depend on a balance between the hydrophilicity and the lipophilicity of the compound diffusing through the skin to give maximum skin penetration. The rate of diffusion of compounds through intact skin also decreases with increasing molecular weight (>500 Da) or size (Naik et al. Citation2000; Jakasa et al. Citation2007).
In intact skin, the stratum corneum is the main barrier to hydrophilic compounds and facilitates the movement of lipophilic compounds. Because these crude extracts are used under conditions where a compromised skin barrier is present, e.g., dermatitis, psoriasis, burns, infections and inflammation, permeation behaviour of compounds will be altered (Davies et al. Citation2015). Previous studies have indicated that diffusion of hydrophilic and amphipathic compounds increases through a compromised barrier of the skin, e.g., where there are abnormalities in the lipids of the stratum corneum, as is the case with psoriasis, dermatitis and UV irradiation (Tsai et al. Citation2001). The epidermal and dermal layers of the skin are hydrophilic and it was shown by a previous study that the viable epidermis also provides a rate limiting step to the diffusion of compounds in the event that the stratum corneum becomes diseased, damaged or absent, as is the case with burn wounds (Andrews et al. Citation2013). When the barrier function of the skin is compromised, the rate of diffusion of most molecules will increase, including compounds of larger molecular size, however, depending on their physicochemical properties, e.g., their lipophilicity. In the case of a compromised barrier function, it can thus be assumed that the compounds that were found diffusing through the intact skin within 10 min from these extracts would diffuse at a much faster rate. The larger and presumably quite lipophilic compounds present in these extracts that did not diffuse through intact skin even after 120 min would be expected to penetrate compromised skin to a far larger extent. In intact skin however, surrounding areas where the skin barrier function is compromised, e.g., burn wounds, could possibly act as a reservoir where active compounds accumulated after topical application to the area, thus extending and enhancing the pharmacological effect, e.g., an antimicrobial effect at the compromised site.
From the previous preliminary phytochemical analyses done in this study where flavonoids, anthraquinones, saponins and tannins were found to be predominantly present in all four of the crude organic extracts, it would largely depend on the physicochemical properties of these groups of compounds to determine their in vitro diffusion across intact skin. Flavonoids possess both hydrophilic and lipophilic properties and contain numerous hydroxyl groups (Rice-Evans & Packer Citation2003; Danihelova et al. Citation2012). In addition, the hydroxyl groups in flavonoids are highly ionizable (Herrero-Martinez et al. Citation2005) and the ionization state will depend on the pH of the surrounding medium. Ionization state of a drug molecule also determines its permeation rate through the skin (Kou et al. Citation1993). In the current study, the permeability experiments were conducted at a physiological pH of 7.4. Flavonoids are weak acids and most flavonoids have pKa values above 7.0 (Rahman Citation2012), since they possess phenyl hydroxyl groups (Liang et al. Citation1997). It has been reported that flavonoids with vicinal-trihydroxyl functional groups are unstable at pH 7.4 (Yao et al. Citation2013), while the 3′,4′-hydroxyflavonoids are negatively charged at pH 7 (Jovanovic et al. Citation1994). Furthermore, the presence of keratin in the stratum corneum presents both negatively and positively charged groups that provide an effective barrier against charged molecules (Bartosova & Bajgar Citation2012). In addition, flavonoids have a high affinity for the stratum corneum, thus, it might be concluded that the flavonoids may not be among the major groups of compounds that permeated through skin; however, these compounds may accumulate within the intact skin exerting a local effect (Rahman Citation2012). Other studies have also found flavonoids to permeate the skin at a very low rate with their permeability usually improved using penetration enhancers (Bonina et al. Citation1996; Saija et al. Citation1998; Arct et al. Citation2002; Vicentini et al. Citation2008). When the skin barrier function, however, becomes compromised, the penetration of flavonoids is expected to be much greater and at a faster rate because the stratum corneum, which is the main barrier to compounds in intact skin, in some cases may be absent (e.g., burn wounds). In the case of skin infection being present, these agents could act on the micro-organisms much more rapidly resulting in an antimicrobial effect.
Saponins contain both lipophilic (sapogenin) and hydrophilic (sugar) properties (Thakur et al. Citation2011). It has been reported that a good candidate drug for topical application must possess both lipophilic and hydrophilic properties to be adequately soluble in both lipophilic (stratum corneum) and hydrophilic (epidermis and dermis) layers of the skin. Because of their ampipathic nature, it can possibly be speculated that saponins might have been among the major compounds that permeated the skin as they were also found to be predominant in all the extracts. Pino et al. (Citation2012) investigated the transdermal permeation of the macromolecules avicins which belong to the family of saponins where high permeability was observed. It was then concluded that both the hydrophilic and hydrophobic portions act synergistically in facilitating the penetration of saponins molecules across the skin lipids. In the case of a compromised skin barrier, these agents are expected to diffuse at a higher rate into the diseased skin tissue exerting a much more rapid pharmacological effect.
The basic chemical structure of all anthraquinones is the merger of three benzene rings (Gessler et al. Citation2013). Drugs with benzene rings in their structures are usually lipophilic and diffuse easily through the stratum corneum of the skin. Thus, anthraquinones were also assumed to be present as the major compounds that were capable of permeating more rapidly across intact skin. The anthraquinone derivatives, danthron and dianthrone, were also found to be capable of permeating the skin in vitro, even though the permeation rate was higher in diseased skin than in normal skin (Wang et al. Citation1987).
Tannins are polyphenolic compounds with a molecular mass extending from 300 to 3000 (Khanbabaee & van Ree Citation2001; Ashok & Upadhyaya Citation2012). These molecules contain a large number of hydroxyl groups rendering them water soluble. Tannins have astringent properties, thus dehydrate the skin and bind as well as precipitates proteins (Ashok & Upadhyaya Citation2012). These compounds are known to form a protective layer over compromised skin and thus as such are not expected to penetrate through intact skin, but rather to accumulate within the skin layers (Ashok & Upadhyaya Citation2012). It would thus be assumed that tannins will penetrate further within compromised skin tissue. However, due to the astringent properties and the ability to bind proteins, tannins would have a strong local effect due to the accumulation within compromised skin layers. The presence of alkaloids, coumarins, flavones and sterols was also indicated in some of the crude extracts. Alkaloids are a diverse group of chemicals found in plants classified as organic nitrogenous bases (Fester Citation2010) of which many show good skin penetration (Chan Citation2012). Alkaloids present in some of the tested extracts are thus also assumed to have diffused rapidly through intact skin. Coumarin is a fragrant compound that occurs naturally in some plants and can be used as an anti-inflammatory or anticancer agent. It penetrates the skin well and is thus also expected to be one of the compounds that penetrated rapidly across intact skin (Beckley-Kartey et al. Citation1997). Plant sterols are similar to cholesterol in structure and when consumed orally can eventually transfer to the skin where they form part of the surface lipids. These compounds are thus not expected to permeate across the skin but rather to accumulate within the skin layers, hydrating the skin and causing anti-inflammatory effects (Gustav Parmentier GmbH Citation2014). Flavones form part of the flavonoids which were described above. As mentioned before, all these compounds would also have penetrated much more rapidly through compromised skin resulting in a more rapid therapeutic effect.
The HPLC profiles of these four organic extracts after permeation through intact skin indicated that there are compounds diffusing across the skin very rapidly within 10 min while the majority of the compounds were not detected even after 120 min exposure to the intact skin. These extracts are mostly used for the treatment of either superficial skin infections, sores, burns or deeper skin infections and the permeability results, to some extent, validate the used of the extracts for these skin inflictions.
Conclusions
In this study, it was observed that the organic extracts displayed higher antimicrobial inhibitory effects than the aqueous extracts with plant species such as A. senegalensis, G. livingstonei, K. africana, O. engleri, P. capensis subsp. capensis, S. brachypetala, S. birrea, S. cordatum as well as E. elephantina (Burch.) Skeels (Fabaceae), T. elegans Stapf. (Apocynaceae) and Z. capense (Thunb.) Harv. (Rutaceae) displaying broad-spectrum antimicrobial effects (average MIC values <1000 μg/mL). Six of the nine plant species (A. burkei, B. discolor, O. engleri, P. capensis subsp. capensis, P. afra and S. rigescenes) documented for the first time in Maputaland as a treatment for skin inflictions also demonstrated positive antimicrobial effects giving some validation to their ethnobotanical uses. This study also demonstrated that the commonly used plants such as S. serratuloides do not necessarily give higher inhibitory effects. This warrants further investigations as the pharmacological activity could be induced by other healing properties.
For the combinations, the results provided some evidence of phytosynergy, thus supporting the traditional method of preparing these combined remedies. The lack of antagonism observed where varied ratios were investigated once again gives credibility for their combined use for therapeutic purposes.
The rapid in vitro diffusion of some chemical compounds from the most antimicrobially active organic extracts of the topically applied plants provided some preliminary scientific insight on the pharmacokinetics of compounds present within these plant extracts intended for topical application through an intact skin barrier. Further studies, however, should be performed on compromised skin, e.g., tape-stripped skin for comparative purposes. The permeated compounds also have to be identified as well as those that possibly accumulated within the skin.
Funding information
The authors would like to thank the University of the Witwatersrand Faculty Research Committee, the National Research Foundation, the University Postgraduate Merit Award, National Students Financial Aid Scheme and the DAAD-NRF-In-Country Scholarship for financial support
Acknowledgements
The authors sincerely thank lay people of northern Maputaland for their hospitality and willingness to share their ethnobotanical knowledge.
Disclosure statement
The authors report no conflicts of interest.
References
- Abbiw DK. 1990. Useful plants of Ghana. West African uses of wild and cultivated plants. United Kingdom: Intermediate Technology Publications. London and Royal Botanic Gardens.
- Agyare C, Dwobeng AS, Agyepong N, Boakye YD, Mensah KB, Ayande PG, Adarkwa-Yiadom M. 2013. Antimicrobial, antioxidant, and wound healing properties of Kigelia africana (Lam.) Beneth. and Strophanthus hispidus DC. Adv Pharmacol Sci. 2013:692613. Article ID
- Ahumuza T, Kirimuhuzya C. 2011. Qualitative (phytochemical) analysis and antifungal activity of Pentas decora (De wild), a plant used traditionally to treat skin fungal infections in Western Uganda. Res Pharm Biotechnol. 7:75–84.
- Al-Hoqail IA. 2012. Epidemiological spectrum of common dermatological conditions of patients attending dermatological consultations in Al-Majmaah Region (Kingdom of Saudi Arabia). J Taibah Univ Med Sci. 8:31–7.
- Andrews SN, Jeong E, Prausnitz MR. 2013. Transdermal delivery of molecules is limited by full epidermis, not just stratum corneum. Pharm Res. 30:1099–109.
- Arct J, Oborska A, Mojski M, Binkowska A, Widzikowska BS. 2002. Common cosmetic hydrophilic ingredients as penetration modifiers of flavonoids. Int J Cosmet Sci. 24:357–66.
- Arkhipov A, Sirdaarta J, Rayan P, McDonnell PA, Cock IE. 2014. An examination of the antibacterial, antifungal, anti-Giardial and anticancer properties of Kigelia Africana fruit extracts. Pharm Commun. 4:62–76.
- Ashok PK, Upadhyaya K. 2012. Tannins are astringent. J Pharm Phytochem. 1:45–50.
- Baba G, Adewumi AAJ, Jere SA. 2014. Toxicity study, phytochemical characterization and anti-parasitic efficacy of aqueous and ethanolic extracts of Sclerocarya birrea against Plasmodium berghei. Br J Pharm Toxicol. 5:59–67.
- Baert B, Deconinck E, van Gele M, Slodicka M, Stoppie P, Bode S, Slegers G, van der Heyden Y, et al. 2007. Transdermal penetration behaviour of drugs: CART-clustering, QSPR and selection of model compounds. Bioorg Med Chem. 15:6943–6955.
- Bartosova L, Bajgar J. 2012. Transdermal drug delivery in vitro using diffusion cells. Curr Med Chem. 19:4671–4677.
- Baskar V, Selvakumar K, Madhan R, Srinivasan G, Muralidharan M. 2012. Study on improving bioavailability ratio of anti-inflammatory compound from ginger through nano transdermal delivery. Asian J Pharm Clin Res. 3:241–246.
- Beckley-Kartey SAJ, Hotchkiss SAM, Capel M. 1997. Comparative in vitro skin absorption and metabolism of coumarin (1,2-benzopyrone) in human, rat, and mouse. Toxicol Appl Pharmacol. 145:34–42.
- Bibi Y, Nisa S, Zia M, Waheed A, Ahmed S, Chaudhary MF. 2012. In vitro cytotoxic activity of Aesculus indica against breast adenocarcinoma cell line (MCF-7) and phytochemical analysis. Pak J Pharm Sci. 25:183–187.
- Bonina F, Lanza M, Montenegro L, Claudio PC, Tomaino A, Trombetta D, Castelli F, Saija A. 1996. Flavonoids as potential protective agents against photo-oxidative skin damage. Intern J Pharm. 145:87–94.
- Boonen J, Baert B, Roche N, Burvenich C, De Spiegeleer B. 2010. Transdermal behaviour of the N-alkylamide spilanthol (affinin) from Spilanthes acmella (Compositae) extracts. J Ethnopharmacol. 127:77–84.
- Chan TYK. 2012. Aconite poisoning following the percutaneous absorption of Aconitum alkaloids. Forensic Sci Int. 223:25–27.
- Cordier W, Gulumian M, Cromarty AD, Steenkamp V. 2013. Attenuation of oxidative stress in U937 cells by polyphenolic-rich bark fractions of Burkea africana and Syzygium cordatum. BMC Complement Altern Med. 13:116
- Cushnie TPT, Lamb AJ. 2005. Antimicrobial activity of flavonoids. Int J Antimicrob Agents. 26:343–356.
- CLSI, 2012. Performance Standards for Antimicrobial Susceptibility Testing; Twenty-second Informational Supplement. CLSI document M100-S22. Wayne (PA): Clinical and Laboratory Standards Institute.
- Dabai TJ, Zuru AA., Hassan LG, Itodo AU, Wara SH. 2013. Phytochemical screening of crude extracts of Amblygocarpus and Sclerocarya birrea. Intern J Trad Nat Med. 2:1–8.
- Danihelova M, Viskupicova J, Sturdík E. 2012. Lipophilization of flavonoids for their food, therapeutic and cosmetic applications. Acta Chim Slovaca. 5:59–69.
- Davies DJ, Heylings JR, McCarthy TJ, Correa CM. 2015. Development of an in vitro model for studying the penetration of chemicals through compromised skin. Toxicol In Vitro. 29:176–181.
- De Barros IB, De Souza DJF, Pinto JP, et al. 2011. Phytochemical and antifungal activity of anthraquinones and root and leaf extracts of Coccoloba mollis on phytopathogens. Brazil Archiv Biol Technol. 54:535–541.
- Deliwe M, Amabeoku GJ. 2013. Evaluation of the antidiarrhoeal and antidiabetic activities of the leaf aqueous extract of Syzygium cordatum Hoscht. ex C. Krauss (Mytraceae) in rodents. Intern J Pharmacol. 9:125–133.
- De Barros IB, De Souza DJF, Pinto JP, Rezende MI, Filho RB, Ferreira DT 2013. Medicinal plants used for the treatment of various skin disorders by rural community of northern Maputaland, South Africa. J Ethnobiomed. 9:51–59.
- De Wet H, Zama VN, van Vuuren SF. 2012. Medicinal plants used for the treatment of sexually transmitted infections by lay people in northern Maputaland, Kwa-Zulu Natal Province, South Africa. S Afr J Bot. 178:12–20.
- Drewes SE, Elliot E, Khan EF, Dhlamini JTB, Gcumisa MSS. 2008. Hypoxis hemerocallidea – not merely a cure for benign prostate hyperplasia. J Ethnopharmacol. 119:593–598.
- Eloff JN. 1998. A sensitive and quick microplate method to determine the minimal inhibitory concentration of plant extracts for bacteria. Planta Med. 64:711–713.
- Eloff JN. 2001. Antibacterial activity of Marula (Sclerocarya birrea (A. rich.) Hochst. subsp. caffra (Sond.) Kokwaro) (Anacardiaceae) bark and leaves. J Ethnopharmacol. 76:305–308.
- Elgorashi EE, Taylor JLS, Maes A, van Staden J, De Kimpe N, Verschaeve L. 2003. Screening of medicinal plants used in South African traditional medicine for genotoxic effects. Toxicol Lett. 143:195–207.
- Fester K. 2010. Plant alkaloids. eLS. doi 10.1002/9780470015902.a0001914.pub2.
- Gabriel OA, Olubunmi A. 2009. Comprehensive scientific demystification of Kigelia africana: a review. Afr J Pure Appl Chem. 3:158–164.
- Garo E, Maillard M, Hostettmann K, Stdeckli-Evans H, Mavi S. 1997. Absolute configuration of a diterpene lactone from Parinari capensis. Helvet Chim Acta. 80:538–44.
- Gessler NN, Egorova AS, Belozerskaya TA. 2013. Fungal anthraquinones. Appl Biochem Microbiol. 49:85–99.
- Gustav Parmentier GmbH. 2014. Phytosterols and phytosterol esters in nutrition and cosmetics. Vorlagen: XiAn Healthful: Phytosterole Broschüre:Phytosterols Brochure.indd. [Online]; [cited 2015 Jun 22]. Available at: http://www.parmentier.de/gpfneu/Sterol_Esters.pdf.
- Heard CM, Johnson S, Moss G, Thomas CP. 2006. In vitro transdermal delivery of caffeine, theobromine, theophylline and catechin from extract of Guarana, Paullinia Cupana. Intern J Pharm. 317:26–31.
- Herrero-Martinez JM, Sanmartin M, Roses M, Bosch E, Rafols C. 2005. Determination of dissociation constants of flavonoids by capillary electrophoresis. Electrophoresis. 26:1886–1895.
- Hutchings A, Scott AH, Lewis G, Cunningham A. 1996. Zulu medicinal plants: an inventory. Pietermaritzburg (South Africa): University of Natal Press.
- Jakasa I, Verberk MM, Esposito M, Bos JD, Kezic S. 2007. Altered penetration of polyethylene glycols into uninvolved skin of atopic dermatitis patients. J Invest Dermatol. 127:129–134.
- Jovanovic SV, Steeden S, Tosic M, Marjanovic B, Simicg MG. 1994. Flavonoids as antioxidants. J Am Chem Soc. 116:4846–4851.
- Kaikabo AA, Samuel BB, Eloff JN. 2009. Isolation and activity of two biflavonoids from leaf extracts of Garcinia livingstonei (Clusiaceae). Nat Prod Commun. 4:1–4.
- Khanbabaee K, van Ree T. 2001. Tannins: classification and definition. Nat Prod Rep. 18:641–649.
- Kisangau DP, Herbert VM, Lyaruu HVM, Hosea KM, Joseph CC. 2007. Use of traditional medicines in the management of HIV/AIDS opportunistic infections in Tanzania: a case in the Bukoba rural district. J Ethnobiomed. 29:1746–4269.
- Kou JH, Roy SD, Du J, Fujiki J. 1993. The effect of receiver fluid pH on in vitro skin flux of weakly ionisable drugs. Pharm Res. 7:986–990.
- Kubmarawa D, Ajoku GA, Enwerem MN, Okorie DA. 2007. Preliminary phytochemical and antimicrobial screening of 50 medicinal plants from Nigeria. Afr J Biotechnol. 6:1690–1696.
- Liang HR, Siren H, Jyske P, Reikkola ML. 1997. Characterization of flavonoids in extracts from four species of Epidemium by micellar electrokinetic capillary chromatography with photo-diode array detection. J Chrom Sci. 35:117–125.
- Mabona U, Viljoen A, Shikanga E, Marston A, van Vuuren SF. 2013. Antimicrobial activity of southern African medicinal plants with dermatological relevance: from an ethnopharmacological screening approach, to combination studies and the isolation of a bioactive compound. J Ethnopharmacol. 148:45–55.
- Magadula JJ, Suleimani HO. 2010. Cytotoxic and anti-HIV activities of some Tanzanian Garcinia species. Tan. J Health Res. 12:1–7.
- Magadula JJ, Tewtrakul S. 2010. Anti-HIV-1 protease activities of crude extracts of some Garcinia species growing in Tanzania. Afr J Biotechnol. 12:1848–1852.
- Mathabe MC, Nikolova RV, Lall N, Nyazema NZ. 2006. Antibacterial activities of medicinal plants used for the treatment of diarrhoea in Limpopo Province, South Africa. J Ethnopharmacol. 105:286–293.
- McGaw LJ, Jäger AK, van Staden J. 2000. Antibacterial, anthelmintic and anti-amoebic activity in South African medicinal plants. J Ethnopharmacol. 72:247–263.
- Naidoo D, van Vuuren SF, van Zyl RL, de Wet H, et al. 2013. Plants traditionally used individually and in combination to treat sexually transmitted infections in northern Maputaland, South Africa: antimicrobial activity and cytotoxicity. J Ethnopharmacol. 149:656–667.
- Naik A, Kalia YN, Guy RH. 2000. Transdermal drug delivery: overcoming the skin's barrier function. Pharm Sci Technol Today. 3:318–326.
- Norscia I, Borgognini-Tarli SM. 2006. Ethnobotanical reputation of plant species from two forests of Madagascar: a preliminary investigation. S Afri J Bot. 72:656–660.
- Ojewole JAO, Mawoza T, Chiwororo WDH, Owira PMO. 2010. Sclerocarya birrea (A. Rich) Hochst. ['Marula'] (Anacardiaceae): a review of its phytochemistry, pharmacology and toxicology and its ethnomedicinal uses. Phytother Res. 24:633–639.
- Okoye TC, Akah PA, Ezike AC, Okoye OM, Onyeto CA, Ndukwu F, Ohaegbulam E, Ikele L. 2012. Evaluation of the acute and sub acute toxicity of Annona senegalensis root bark extracts. Asian Pac J Trop Med. 5:277–282.
- Pallant CA, Steenkamp V. 2008. In vitro bioactivity of Venda medicinal plants used in the treatment of respiratory conditions. Hum Exp Toxicol. 27:859–867.
- Park SN, Kim SY, Lim GN, Jo NR, Lee MH. 2012. In vitro skin permeation and cellular protective effects of flavonoids isolated from Suaeda asparagoides extracts. J Ind Eng Chem. 18:680–683.
- Pino CJ, Gutterman JU, Vonwil D, Samir Mitragotri S, Shastri VP. 2012. Glycosylation facilitates transdermal transport of macromolecules. Proc Natl Acad Sci USA. 109:21283–21288.
- Pooley E. 2003. The complete field guide to trees of Natal Zululand and Transkei. Durban (South Africa): Natal Flora Publications Trust.
- Prasad RN, Viswanathan S, Devi JR, Nayak V, Swetha VC, Archana BR, Parathasarathy N, Rajkumar J. 2008. Preliminary phytochemical screening and antimicrobial activity of Samanea saman. J Med Plant Res. 10:268–270.
- Priya B, Gahlot M, Joshi P, Zade S. 2012. Preliminary phytochemical screening and in vitro anthelmintic activity of Kigelia africana (Bignoniaceae). Intern J Chem Sci. 10:1799–1804.
- Priya B, Menkudale A, Gahlot M, Joshi P, Agarwal M, et al. 2013. Pharmacognostical study, phytochemical analysis and phenolic content of Kigelia africana leaves. Intern J Pharmacy Pharm Sci. 5:163–166.
- Quave CL, Pieroni A, Bennett BC. 2008. Dermatological remedies in the traditional pharmacopoeia of Vulture-Alto Bradano, inland southern Italy. J Ethnobiomed. 4:1–10.
- Rahman A. 2012. Studies in natural product chemistry, vol. 36. New York (NY): Elsevier Publishers.
- Rice-Evans CA, Packer L. 2003. Flavonoids in health and disease. 2nd ed. New York (NY): Marcel Dekker Inc.
- Saravanakumar A, Venkateshwaran K, Vanitha J, Ganesh M, Vasudevan M, Sivakumar T. 2009. Evaluation of antibacterial activity, phenol and flavonoid contents of Thespesia populnea flower extracts. Pak J Pharm Sci. 22:282–286.
- Saija A, Tomaino A, Trombetta D, Marcella GM, de Pasquale A, Bonina F., et al. 1998. Influence of different penetration enhancers on in vitro skin permeation and in vivo photoprotective effect of flavonoids. Intern J Pharm. 175:85–94.
- Sidney MT, Siyabonga SJ, Kotze BA. 2015. Evaluation of the antibacterial activity of Syzygium cordatum fruit-pulp and seed extracts against bacterial strains implicated in gastrointestinal tract infections. Afr J Biotechnol. 16:1387–1392.
- Solomon IS, Umoh IN, Kayode D. 2014. Phytochemical screening and free radical scavenging activities of the fruit and leaves of Kigelia africana (Bignoniaciae). ARPN J Sci Tech. 2:123–128.
- Sparg SG, Light ME, van Staden J. 2004. Biological activities and distribution of plant saponins. J Ethnopharmacol. 94:219–243.
- Statistics South Africa: Community Survey 2007: Municipal Data on Household Services. [Online]; [cited 2015 Feb 22]. Available at: http://www.statssa.gov.za/community_new/content.asp.
- Steenkamp V, Fernandes AC, van Rensburg CEJ. 2007. Screening of Venda medicinal plants for antifungal activity against Candida albicans. S Afri J Bot. 73:256–258.
- Suliman S, van Vuuren SF, Viljoen AM. 2010. Validating the in vitro antimicrobial activity of Artemisia afra in polyherbal combinations to treat respiratory infections. S Afri J Bot. 76:655–661.
- Taylor JLS, Esameldin E, Elgorashi EE, Maes A, van Gorp U, De Kimpe N, van Staden J, et al. 2003. Investigating the safety of plants used in South African traditional medicine: testing for genotoxicity in the micronucleus and alkaline comet assays. Environ Mol Mutagen. 42:144–154.
- Thakur M, Melding MF, Fuchs H, Weng A. 2011. Chemistry and pharmacology of saponins: special focus on cytotoxic properties. Bot: Targets Therapy. 1:19–29.
- Tsai J-C, Sheu H-M, Hung P-L, Cheng C-L. 2001. Effect of barrier disruption by acetone treatment on the permeability of compounds with various lipophilicities: implications for the permeability of compromised skin. J Pharm Sci. 90:1242–1254.
- Uys ACU, Malan SF, van Dyk Svan Zyl RL. 2002. Antimalarial compounds from Parinari capensis. Bioorg Med Chem Lett. 12:2167–9.
- Van Vuuren SF, Viljoen AM. 2008. In vitro evidence of phyto-synergy for plant part combinations of Croton gratissimus (Euphorbiaceae) used in African traditional healing. J Ethnopharmacol. 119:700–704.
- Van Vuuren SF, Viljoen AM. 2011. Plant-based antimicrobial studies-methods and approaches to study the interaction between natural products. Planta Med. 77:1168–1182.
- Van Vuuren SF, Nkwanyana MN, De Wet H. 2015. Antimicrobial evaluation of plants used for the treatment of diarrhoea in a rural community in northern Maputaland, KwaZulu-Natal, South Africa. BMC Complement Alt Med. 15:53.
- Van Wyk B, Gericke N. 2000. People’s plants, 1st ed. Pretoria (South Africa): Briza Publications.
- Van Wyk B, van Oudtshoorn B, Gericke N. 2009. Medicinal plants of South Africa, 2nd ed. Pretoria (South Africa): Briza Publications.
- Vicentini FTMC, Simi TTRM, Ciampo JOD, Wolga NO, Pitol DL, Iyomasa MM, Bentley VLB, Fonseca MJV, et al. 2008. Quercetin in w/o microemulsion: In vitro and in vivo skin penetration and efficacy against UVB-induced skin damages evaluated in vivo. Eur J Pharm Biopharm. 69:948–957.
- Wang JC, Patel BG, Ehmann CW, Lowe N. 1987. The release and percutaneous permeation of anthralin products, using clinically involved and uninvolved psoriatic skin. J Am Acad Dermatol. 16:812–821.
- Wanyama PAG, Kiremire BT, Murumu JES, Kamoga O. 2011. Textile dyeing and phytochemical characterization of crude plant extracts derived from selected dye-yielding plants in Uganda. Intern J Nat Prod Res. 2:26–31.
- Yao Y, Lin G, Xie Y, Ma P, Li G, Meng Q, Wu T. 2013. Preformulation studies of myricetin: a natural antioxidant flavonoids. Pharmazie. 69:19–26.
- York T, De Wet H, van Vuuren SF. 2011. Plants used for treating respiratory infections in rural Maputaland, KwaZulu-Natal, South Africa. J Ethnopharmacol. 135:696–710.
- York T, van Vuuren SF, De Wet H. 2012. An antimicrobial evaluation of plants used for the treatment of respiratory infections in rural Maputaland, KwaZulu-Natal, South Africa. J Ethnopharmacol. 144:118–127.
- Zonyane S, Van Vuuren SF, Makunga NP. 2013. Antimicrobial interactions of Khoi-San poly-herbal remedies with emphasis on the combination; Agathosma crenulata, Dodonaea viscosa and Eucalyptus globulus. J Ethnopharmacol. 148:144–151.