Abstract
Context: Myrtenal is monoterpene a constituent of essential oils found mainly in herbs such as mint, pepper, cumin, etc. It exerts admirable pharmacological activities against many diseases including diabetes. Hyperlipidaemia is a secondary complication of diabetes and also a major risk factor for cardiovascular diseases.
Objective: The present study investigated the possible antihyperlipidaemic efficacy of myrtenal on plasma glucose, pancreatic insulin, plasma and tissue lipid levels in streptozotocin (STZ) induced diabetic rats.
Materials and methods: Diabetes was induced in male Wistar rats by a single intraperitoneal injection of STZ (40 mg/kg b.w.). Myrtenal (80 mg/kg) was administered orally to diabetic rats for a period of 28 d. Plasma glucose, pancreatic insulin, TC, TGs, FFAs, PLs, LDL-C, HDL-C, VLDL, atherogenic index, (HMG-CoA) reductase, LPL, LCAT and liver histology were analyzed.
Results: Diabetic rats showed significantly (p < 0.05) increased plasma glucose (273.18 mg/dL), total cholesterol (142 mg/dL), triglycerides (126 mg/dL), free fatty acids (118 mg/dL), phospholipids (153 mg/dL), low-density lipoprotein (88.07 mg/dL), very low-density lipoprotein (25.2 mg/dL), atherogenic index, whereas a decrease in the levels of pancreatic insulin (97.48 ng/mg) and high-density lipoprotein (29.12 mg/dL). In addition, the activity of 3-hydroxy 3-methylglutaryl coenzyme A (HMG-CoA) reductase (0.94 HMG-CoA ratio/(mevalonate) increased significantly in contrast to the activities of lipoprotein lipase (4.87 μmoles of glycerol liberated/h/L) and lecithin cholesterol acyltransferase (54.61 μmoles of cholesterol esterified/h/L) in diabetic rats. Treatment with myrtenal significantly (p < 0.05) improved the levels of plasma glucose, pancreatic insulin and lipid profiles. Moreover, the histopathological analysis of liver was also in agreement with the biochemical findings.
Discussion and conclusions: The present study indicates that myrtenal possess antihyperglycaemic and antihyperlipidemic properties, and could potentially be a useful phytochemical in treating diabetes.
Introduction
Diabetes mellitus (DM) is a disease described by hyperglycaemia and diabetic dyslipidaemia, which are linked to hypoinsulinaemia or insensitivity of target organs like liver, adipose tissue, skeletal muscle to insulin. Insulin resistance lead to an abnormal lipid profile, which is frequently observed in diabetic conditions (Kulkarni et al. Citation2012). DM is strongly linked with a higher degree of heart disease. Macrovascular diseases commonly found in diabetic patients associated with hyperglycaemia and could be responsible for the development of cardiovascular complications (Okutan et al. Citation2005). Patients with DM2 show significant modifications in the plasma/serum lipid profile including TGs, LDL and HDL. Abnormal state of total cholesterol during diabetes also serves as major risk factors for coronary heart diseases (Tan et al. Citation2005; Verges Citation2009).
Several drugs are in use for controlling hyperglycaemia associated with hyperlipidaemia; however, the existing therapy for diabetes is associated with number of side effects. Hence, to achieve better glycaemic control, antidiabetic drug discovery has shifted its focus to natural plant sources having minimal side effects (Moler Citation2001). Interestingly, several studies have reported that monoterpenes exert abundant medicinal values on various diseases including diabetes. A number of monoterpenes are widely used in the agriculture, cosmetic and food production (Aeschbach et al. Citation1994; Lee et al. Citation1997; Manou et al. Citation1998). They contain diverse pharmacological properties with antidiabetic, hypocholesterolaemic, hypotensive, vasorelaxant, antioxidant, anticancer, antifungal, antibacterial and anti-spasmodic (Marcio et al. Citation2011; Murali & Saravanan Citation2012; Ezhumalai et al. Citation2014). Previous reports documented that monoterpenes could be functional phytochemicals for the management of cardiovascular diseases (CVD) (Bastos et al. Citation2010; Peixoto-Neves et al. Citation2010).
Myrtenal is a natural monoterpene found in many medicinal plants such as pepper, cumin, mint and eucalyptus. It has been reported to possess a wide range of beneficial properties such as antioxidant, anticancer, cyclooxygenase-inhibitor and immunostimulant effect (Lindmark et al. Citation2004; Vibha et al. Citation2009; Babu et al. Citation2012). Recently, we have found that myrtenal has antidiabetic and hepatoprotective effects in experimentally induced diabetic rats (Rathinam et al. Citation2014). But, there is insufficient evidence in the literature to make a definitive conclusion about the effects of myrtenal on lipid profile. Considering these facts and lack of information about the pharmacological effect of nyrtenal in diabetes, we have investigated the antidiabetic and lipid lowering efficacy of myrtenal in streptozotocin (STZ)-induced diabetic rats.
Materials and methods
Animals
Male healthy albino rats of Wistar strain (180–200 g) were obtained from the Central Animal House, Department of Experimental Medicine, Rajah Muthiah Medical College and Hospital, Annamalai University, and maintained at a constant temperature (25 ± 1 °C) on a 12 h light/dark cycle with food (standard diet contained 60% starch, 20% protein and 4.38% fat) and water ad libitum. The experimental protocol was approved by the animal ethics committee of Rajah Muthiah Medical College and Hospital (Reg. no. 160/1999/CPCSEA, Proposal number: 1000), Annamalai University.
Chemicals
Myrtenal and streptozotocin were purchased from Sigma-Aldrich (St. Louis, MO). All other chemicals used in this study were of analytical grade and were obtained from Merck and Hi Media, Mumbai, India.
Induction of diabetes in rats
Diabetes mellitus was induced in overnight fasted rats by a single intraperitoneal injection (i.p.) of streptozotocin (STZ-40 mg/kg b.w.) dissolved in 0.1 M citrate buffer at pH 4.5. STZ-injected animals were given 20% glucose solution for 24 h to prevent initial drug-induced hypoglycaemia and associated mortality (Punithavathi et al. Citation2011; Ramachandran & Saravanan Citation2013). STZ-injected animals exhibited hyperglycaemia within a few days and the onset of diabetes was confirmed by measuring the elevated fasting plasma glucose (by glucose oxidase method) 72 h after STZ injection. Rats with fasting blood glucose above 250 mg/dl were considered to be diabetic and used for the experiment.
Treatment schedule
The rats were divided into four groups, each comprising of six rats (6 per group ×4 groups =24 rats). Myrtenal was dissolved in 1 mL of corn oil and administered orally to the rats using an intragastric tube daily for a period of 28 d (Rathinam et al. Citation2014).
Group I: normal control rats.
Group II: normal + myrtenal (myt) (80 mg/kg bw/d).
Group III: diabetic control rats.
Group IV: diabetic + myrtenal (myt) (80 mg/kg bw/d).
At the end of the experimental period, all the animals were fasted overnight, anaesthetized and sacrificed by cervical dislocation. Blood samples were collected in vacutainers containing EDTA. The tubes were centrifuged at 20 min (2000 g at 4 °C) for separation of plasma and used for the determination of lipid parameters. Liver tissue was used for the extraction of lipids. Liver tissue was dissected out, washed in ice-cold saline and stored at −80 °C until used. The tissue was weighed and 10% tissue homogenate was prepared with 0.1 M Tris–HCl buffer, pH 7.4. After centrifugation, the clear supernatant was obtained and used for biochemical assays.
Plasma glucose and pancreatic insulin assay
Fasting plasma glucose was determined using diagnostic kits (Agappe Diagnostic Pvt. Ltd., Kerala, India). Immediately following removal of pancreas, they were homogenized with 20 mL of cold, acidified ethanol solution (75% ethanol, 23.5% water and 1.5% HCl in 1.8 mL volume) per gram of pancreatic tissue. The homogenate was kept at 4 °C overnight and then centrifuged at 600 g for 30 min at 4 °C. The supernatant was diluted 1:10,000 in 0.1 M PBS containing 0.25% BSA and was used for insulin concentration measurement using ELISA Kit (Boeheringer-Manneheim Kit, Manneheim, Germany) according to instructions of the manufacturer.
Extraction and assay of lipid profile
Total lipids were extracted from plasma and liver tissues according to the method of Folch et al. (Citation1957). Total cholesterol (TC), triglycerides (TGs), free fatty acids (FFAs) and phospholipids (PLs) were measured by the method of Zlatkis et al. (Citation1953), Fossati and Lorenzo (Citation1982), Falholt et al. (Citation1973) and Zilversmit and Davis (Citation1950), respectively.
Measurement of plasma lipoproteins
The HDL-C content in plasma was measured using a reagent kit (Qualigens Diagnostics, Mumbai, India). VLDL-C and LDL-C fractions were calculated as VLDL-C = TG/5 and LDL-C = TC − (HDL-C + VLDL-C) and atherogenic index (AI) = LDL-C/HDL-C correspondingly (Friedwald et al. Citation1972; Abbott et al. Citation1988).
Assessment of lipid marker enzymes
The activity of 3-hydroxy-3-methylglutaryl coenzyme A (HMG-CoA reductase) in the liver was assayed by the method of Philipp and Shapiro (Citation1970). Lipoprotein lipase (LPL) activity was assayed by the method of Korn (Citation1955). Lecithin cholesterol acyltransferase (LCAT) activity was assayed by the method of Hitz et al. (Citation1983).
Histological observation
The liver was instantly dissected out, excised and rinsed in ice-cold saline solution. A fraction of liver was fixed in 10% neutral buffered formalin fixative solution. After fixation, tissue was embedded in paraffin; solid sections of 4–5 μm thickness were made by using a rotary microtome. The sections were stained with haematoxylin–eosin and histological observations were made under a light microscope (400×). To measure the liver damage (semi-quantitative measurements) for better comprehension we used software measure IT-Olympus Soft Imaging Solution GmbH (version-5.2, Olympus, Tokyo, Japan).
Statistical analysis
Data were presented as means ± S.D. and were subjected to one way analysis of variance (ANOVA) using SPSS Version 17.0 (SPSS, Cary, NC) and the individual comparisons were obtained by Duncan’s multiple range test (DMRT). Values were considered statistically significant at p< 0.05.
Results
Myrtenal improves the pancreatic insulin levels
To assess the antihyperglycaemic effect of myrtenal, we analyzed the plasma glucose and pancreatic insulin level in normal control and experimental rats. Indeed, diabetic rats showed hyperglycaemia and a fall in pancreatic insulin level were observed. Myrtenal (80 mg/kg b.w) treatment markedly decreased hyperglycaemia and improved insulin level was observed in diabetic rats ().
Table 1. Effect of myrtenal on plasma glucose and pancreatic insulin levels in normal control and experimental rats.
Myrtenal decreased the plasma lipids
shows the levels of plasma lipids TC, TGs, FFAs and PLs were significantly increased in diabetic rats as compared with normal control rats. On the contrary, treatment with myrtenal (80 mg/kg b.w.) significantly decreased the plasma lipids in diabetic rats as compared with diabetic control rats.
Table 2. Effect of myrtenal on the levels of plasma lipids TC, TGs, FFAs and PLs in normal and experimental rats.
Myrtenal decreased the tissue lipids
The liver tissue lipids TC, TGs, FFAs and PLs levels are shown in . Diabetic rats showed significant elevation of TC, TGs, FFAs and PLs when compared with normal control rats. Myrtenal (80 mg/kg b.w.) treatment significantly decreased the levels of liver tissue lipids TC, TGs, FFAs and PLs in diabetic rats as compared with diabetic control rats.
Table 3. Effect of myrtenal on the levels of liver TC, TGs, FFAs and PLs in normal control and experimental rats.
Myrtenal effect on LDL-C, VLDL-C and HDL-C
Diabetic rats exhibited increased levels of plasma LDL-C, VLDL-C and decreased levels HDL-C as compared with normal control rats. Oral treatment with myrtenal (80 mg/kg b.w.) in diabetic rats significantly improved plasma lipoproteins levels as compared with diabetic control rats ().
Figure 1. Effect of myrtenal on plasma lipoproteins in normal control and experimental rats. Values are represented as means ± SD for six rats in each group. Values are not sharing a common superscript letter (a–c) differ significantly at p < 0.05 (DMRT); NC, normal control; DC: diabetic control. aNC and N + Myt significant as compared to Diabetic and Diabetic + Myt (p < 0.05). bDiabetic significant as compared with NC, N + Myt and diabetic + Myt (p < 0.05). cDiabetic + Myt significant as compared with diabetic, NC and N + Myt (p < 0.05).
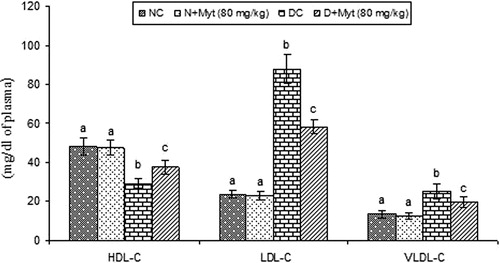
Myrtenal decreased the activities of LPL and LCAT
A significant decrease in the activities of LPL and LCAT was noted in plasma of diabetic rats as compared with normal rats. Treatment with mytenal (80 mg/kg b.w.) in diabetic rats markedly improved the LPL and LCAT enzyme activities as compared with diabetic control rats ().
Figure 2. Effect of myrtenal on the activities of LPL and LCAT in normal control and experimental rats. Values are represented as means ± SD for six rats in each group. Values are not sharing a common superscript letter (a–c) differ significantly at p < 0.05 (DMRT); NC: normal control; DC: diabetic control. aNC and N + Myt significant as compared with diabetic and diabetic + Myt (p < 0.05). bDiabetic significant as compared with NC, N + Myt and diabetic + Myt (p < 0.05). cDiabetic + Myt significant as compared with diabetic, NC and N + Myt (p < 0.05).
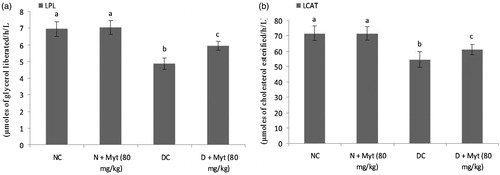
Myrtenal decreased the activity of HMG-CoA reductase
Diabetic rats show significantly increased activity (decreased HMG-CoA/mevalonate ratio) in liver. Oral administration of myrtenal (80 mg/kg b.w.) in diabetic rats significantly decreased the activity as compared with diabetic control rats ().
Figure 3. Effect of myrtenal on the activity of HMG-CoA reductase in the liver of normal control and experimental rats. Values are represented as means ± SD for six rats in each group. Values are not sharing a common superscript letter (a–c) differ significantly at p < 0.05 (DMRT); NC: normal control; DC: diabetic control. aNC and N + Myt significant as compared with diabetic and diabetic + Myt (p < 0.05). bDiabetic significant as compared with NC, N + Myt and diabetic + Myt (p < 0.05). cDiabetic + Myt significant as compared with diabetic, NC and N + Myt (p < 0.05).
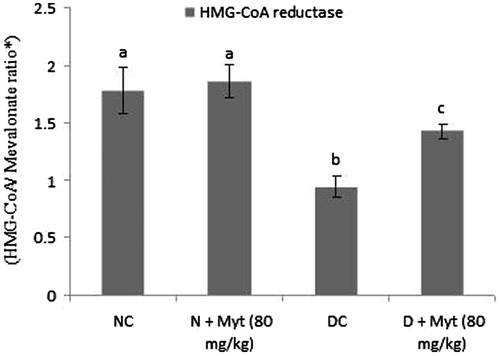
Histology of liver
shows the histology of rat liver stained with H&E. (a and b) shows orderly arranged hepatocyte morphology. Diabetic rat liver showed the microvesicular steatosis (c). Diabetic + myrtenal (80 mg/kg b.w.) treated rat liver revealed few micro-fat deposition and mild degeneration hepatocyte (d).
Figure 4. (a) Effect of myrtenal on liver fat accumulation in experimental diabetic rats (H&E, 40×). Histology of liver in experimental rats after 28 d of myrtenal treatment (A) normal control and (B) normal control + myrtenal (80 mg/kg b.w) alone treated rat liver shown orderly arranged hepatocyte morphology, (C) diabetic rat liver shows the microvesicular steatosis, (D) diabetic + myrtenal (80 mg/kg b.w) treated rat liver shows few micro-fat deposition and mild degeneration hepatocyte. (b) Semi-quantitative analysis of liver histology. aNC and N + Myt significant as compared with diabetic and diabetic + Myt (p < 0.05). bDiabetic significant as compared with NC, N + Myt and diabetic + Myt (p < 0.05). cDiabetic + Myt significant as compared with diabetic, NC and N + Myt (p < 0.05).
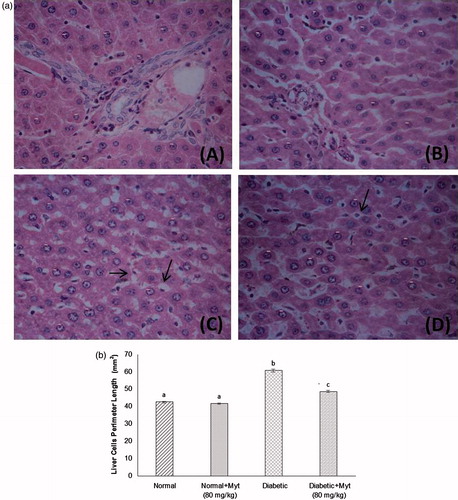
Histological score
shows liver histological changes and tissue injury score significantly increased in diabetic rats as compared with normal control rats. Treatment with myrtenal (80 mg/kg b.w.) significantly decreased the tissue injury level in diabetic rats.
Discussion
DM2 is caused by increased blood glucose, insulin resistance and/or insulin deficiency. It is increasingly acknowledged that insulin resistance contributes to the dyslipidemia associated with type 2 DM (Adiels et al. Citation2006). The characteristic features of dyslipidemia are augmented concentration of plasma TGs, low HDL-C concentration and improved concentration of small dense LDL-C particles. The lipid changes associated with DM2 are attributed to increased free fatty acid flux secondary to insulin resistance (Mooradian Citation2009).
Insulin is a potent inhibitor of lipolysis by inhibiting the activity of the lipases and holds back the release of FFAs from the adipose tissue (Al-Shamaony et al. Citation1994). An elevated activity of this enzyme observed under diabetic condition that enhances the lipolysis and releases FFAs into the circulation which in turn increases the β-oxidation of fatty acids. The resultant increase in circulating FFAs contributes to an increase in hepatic TGs. In the present study, diabetic rats showed increased the level of FFAs in plasma and liver.
Diabetic patients are frequently monitored the hypertriglyceridaemia and is involved in the progression of vascular complications (Kudchodkar et al. Citation1988). Increased TGs level in diabetes might be due to elevated flux of FAs and impaired mobilization of VLDL from circulation. TGs enhance the regulation of lipoprotein interaction to sustain usual lipid metabolism. Insulin-dependent activity of LPL enzyme in peripheral tissues interacts with TGs-rich lipoprotein in endothelial cell where it hydrolyzes triglyceride into FFAs. The deficiency of insulin reduces the LPL activity and produces hypertriglyceridaemia (Taskinen Citation1987). In our study, diabetic rats exhibited significant elevation of TGs with a decrease in LPL activity. Administration of myrtenal in diabetic rats decreased the TGs level in plasma and tissue. This could be due to the improved insulin secretion which in turn increases LPL activity.
Cellular cholesterol homeostasis is vital for the prevention of cardiovascular disease. It has been established that accumulation of lipids in hepatic tissue due to the insulin deficiency that leads to a series of derangements in metabolic and regulatory processes in DM. Hypercholesterolaemia is the most frequently observed lipid abnormality in diabetes. The degree of hypercholesterolaemia is directly proportional to severity in diabetes (Shepherd Citation2005). The coronary heart diseases developed by the accumulation of total cholesterol which increases the incidence of atherosclerosis in diabetes (Nielsen et al. Citation2002). Cholesterol levels were increased due to augmented cholesterol biosynthesis in DM (Kim et al. Citation2008). HMG-CoA reductase catalyzes the cholesterol metabolism biosynthesis and its activity was found to be significantly increased in the liver of diabetic rats (Al-Shamaony et al. Citation1994). In the present study, cholesterol levels were increased in diabetic rats which could be due to increased cholesterogenesis. Diabetic rats treated with myrtenal reduced the cholesterol level and decreased the HMG-CoA reductase activity.
PLs are essential for the bile synthesis and lipoprotein secretion is important for cellular membrane integrity (Vance & Vance Citation2005). It acts as an interface with together hydrophilic region and hydrophobic lipoprotein of lipoprotein core (Cohn & Roth Citation1996). In a previous study, STZ-diabetic rats showed increased PLs level due to elevated levels of FFAs (Frayn Citation1993) which can promote PLs synthesis. In the present study, PLs are increased in the plasma and liver of diabetic rats. However, oral treatment of myrtenal reduced the level of PLs in plasma and liver of diabetic rats.
According to the literature, the cause of improvement in cholesterol level in the blood depends on recovery of liver function, which is a key organ for lipid metabolism. Thus, it is important that antidiabetic agents should improve hepatic function to maintain normal level of glucose and insulin in blood stream. In our study, STZ-induced diabetic rats showed aberrant levels of FFAs, TGs, TC and PLs in plasma and liver. Myrtenal-treated diabetic rats improved the levels of FFAs, TGs, TC and PLs. Our previous findings clearly pointed out that myrtenal improved the glucose and insulin levels in diabetic rats (Rathinam et al. Citation2014), which offer the recovery of hepatic function and normalize the altered level of FFAs, TGs, TC and PLs in diabetic rats. Similar findings were observed with linalool, a plant-derived monoterpene, in a model of experimentally induced diabetic nephropathy (Deepa & Anuradha Citation2011).
LDL-C is involved in the transport of cholesterol from liver to peripheral tissue. This could be regarded as possible cause in the development of atherogenesis. Recent studies demonstrate that LDL-C and apolipoprotein-B secretion were increased in type 2 DM. The increased LDL-C in diabetic patients follow abnormal metabolism and is associated with an increase in VLDL-C secretion and impaired VLDL-C catabolism (Duvillard et al. Citation2000). In addition, VLDL-C and chylomicrons play a vital role in terms of contributing exterior apoproteins and lipids to HDL-C during hydrolysis by LPL. Increased LDL-C may arise on glycosylation of the lysyl residue of apoprotein-B as well as from decreasing affinity for the LDL receptor (Golay et al. Citation1986). Oral administration of myrtenal normalized these effects, possibly by controlling the hydrolysis of lipoproteins.
Liver is an important organ for the cholesterol metabolism where HDL-C brings cholesterol from the peripheral tissues to the liver thereby cholesterol gets metabolized into bile acids (Singh et al. Citation2007). Indeed, this pathway plays a vital role in reducing cholesterol levels in the blood and peripheral tissues (Garvey et al. Citation2003). The decreased plasma HDL level in diabetic rats may be due to the reduced production of HDL by intestine and liver and/or due to the glycation of HDL or its apoproteins by high glucose rendering it more pro-atherogenic.
LCAT is synthesized in the liver and circulates in the blood. LCAT is the key enzyme in plasma which is involved in the esterification of free cholesterol to cholesteryl esters in plasma and also responsible for HDL-C metabolism (Borggreve et al. Citation2003). Hyperglycaemia leads to non-enzymatic glycation of proteins including Apo A-I. HDL gets glycation on serine side chains in its most abundant protein moiety (Apo A-I) causing conformational alterations eventually resulting in reduced LCAT activity. The decreased activity of LCAT could affect the reverse cholesterol transportation of HDL-C and decreases the levels of mature HDL-C particles, which may eventually lead to severe impairment of HDL-C synthesis and metabolism in diabetes (Kripa et al. Citation2011). Treatment with myrtenal in diabetic rats enhances the insulin secretion and averts the accumulation of cholesterol by stimulating the activity of the enzyme LCAT. This could be indicative of an enhanced capacity for HDL maturation and the shift towards improved HDL level was seen with myrtenal treatment. Our finding is consistent with previous study which reported that treatment with ursolic acid, a terpenoid, increases LCAT activity (Celio et al. Citation2010).
Conclusion
In conclusion, myrtenal positively modulates lipid metabolism in diabetic rats. Moreover, our study reveals the antihyperglycaemic and antihyperlipidaemic effect of myrtenal in STZ-induced diabetic rats. However, further studies are in progress to pin-point the mechanism underlying the pharmacological action of myrtenal against STZ-induced diabetic rats.
Funding information
The authors thank the University Grants Commission (UGC), New Delhi, India, for the financial support in the form of research fellow under Research Fellowship in Science for Meritorious Students (RFSMS) Scheme (F.25-1/2013-14(BSR)/7/10/2007) to Mr. Ayyasamy Rathinam.
Disclosure statement
The author report that they have no conflicts of interest.
References
- Abbott RD, Wilson PW, Kannel WB, Castelli WP. 1988. High density lipoprotein cholesterol, total cholesterol screening, and myocardial infarction. The Framingham Study. Arteriosclerosis. 8:207–211.
- Adiels M, Olofsson SO, Taskinen MR, Borén J. 2006. Diabetic dyslipidaemia. Curr Opin Lipidol. 17:238–246.
- Aeschbach R, Loliger J, Scott BC, Murcia A, Butler J, Halliwell B, Aruoma OI. 1994. Antioxidant actions of thymol, carvacrol, 6-gingerol, zingerone and hydroxytyrosol. Food Chem Toxicol. 32:31–36.
- Al-Shamaony L, Al-Khazraji SM, Twaij HA. 1994. Hypoglycaemic effect of Artemisia herba alba. II. Effect of a valuable extract on some blood parameters in diabetic animals. J Ethnopharmacol. 43:167–171.
- Babu LH, Perumal S, Balasubramanian MP. 2012. Myrtenal, a natural monoterpene, down-regulates TNF-α expression and suppresses carcinogen-induced hepatocellular carcinoma in rats. Mol Cell Biochem. 369:183–193.
- Bastos JF, Moreira IJ, Ribeiro TP, Medeiros IA, Antoniolli AR, De Sousa DP, Santos MR. 2010. Hypotensive and vasorelaxant effects of citronellol, a monoterpene alcohol in rats. Basic Clin Pharmacol Toxicol. 106:331–337.
- Borggreve SE, DeVries R, Dullaart RPF. 2003. Alterations in high-density lipoprotein metabolism and reverse cholesterol transport in insulin resistance and type 2 diabetes mellitus: role of lipolytic enzymes, lecithin: cholesterol acyltransferase and lipid transfer proteins. Eur J Clin Invest. 33:1051–1069.
- Celio L, Meloa D, Maria R, Queiroza G, Said Fonsecaa GC, Ayla Bizerrab MC, Telma Lemosb LG, Tiago Meloc S, Flavia Santosc A, Vietla Rao S. 2010. Oleanolic acid, a natural triterpenoid improves blood glucose tolerance in normal mice and ameliorates visceral obesity in mice fed a high-fat diet. Chem Biol Interact. 185:18559–18565.
- Cohn RM, Roth KS. 1996. Lipid and lipoprotein metabolism. Biochemistry and disease. Baltimore: Williams and Wilkins Publishers.
- Deepa B, Anuradha CV. 2011. Linalool, a plant derived monoterpene alcohol, rescues kidney from diabetes induced nephropathic changes via blood glucose reduction. Diabetol Croat. 40–44.
- Duvillard L, Pont F, Florentin E, Galland-Jos C, Gambert P, Vergès B. 2000. Metabolic abnormalities of apolipoprotein B-containing lipoproteins in noninsulin-dependent diabetes: a stable isotope kinetic study. Eur J Clin Invest. 30:685–694.
- Ezhumalai M, Radhiga T, Pugalendi KV. 2014. Antihyperglycemic effect of carvacrol in combination with rosiglitazone in high-fat diet-induced type 2 diabetic C57BL/6J mice. Mol Cell Biochem. 385:23–31.
- Falholt K, Falholt W, Lund B. 1973. An easy colorimetric micro method for routine determination of free fatty acids in plasma. Clin Chim Acta. 46:105–111.
- Folch J, Lees M, Solane SGH. 1957. A simple method for isolation and purification of total lipids from animal tissues. J Biol Chem. 26:497–509.
- Fossati P, Lorenzo P. 1982. Serum triglycerides determined colorimetrically with an enzyme that produces hydrogen peroxide. Clin Chem. 28:2077–2080.
- Frayn KN. 1993. Insulin resistance and lipid metabolism. Curr Opin Lipidol. 4:197–204.
- Friedwald WT, Levy RI, Fredrickson DS. 1972. Estimation of the concentration of LDL-C in plasma without use of the preparative ultracentrifuge. Clin Chem. 18:449–502.
- Garvey WT, Kwon S, Zheng D, Shaughnessy S, Wallace P, Hutto A, Pugh K, Jenkins AJ, Klein RL, Liao Y. 2003. Effects of insulin resistance and type 2 diabetes on lipoprotein subclass particle size and concentration determined by nuclear magnetic resonance. Diabetes. 52:453–462.
- Golay A, Chen YD, Reaven GM. 1986. Effect of differences in glucose tolerance on insulin stability regulate carbohydrate and free fatty acid metabolism in obese individuals. J Clin Endocrinol Metabol. 86:1081–1088.
- Hitz J, Steinmetz J, Siest G. 1983. Plasma lecithin: cholesterol acyltransferase-reference values and effects of xenobiotics. Clin Chim Acta. 133:85–96.
- Kim H, Jeong D, Jung H, Jung YJ, Yokozawa T, Choi JS. 2008. Hypolipidemic effects of sophora flavescens and its constituents in poloxamer407-induced hyperlipidemic and cholesterol-fed rats. Biol Pharm Bull. 31:73–78.
- Korn ED. 1955. Clearing factor, a heparin-activated lipoprotein lipase. I. Isolation and characterization of the enzyme from normal rat heart. J Biol Chem. 215:1–26.
- Kripa K, Vijayalakshmi NR, Helen A. 2011. Methanolic extract of costusigneus (N.E.Br.) alleviates dyslipidemia in diabetic rats. Asian J Pharm Clin Res. 4:154–157.
- Kudchodkar BJ, Lee JC, Lee SM, DiMarco NM, Lacko AG. 1988. Effect of dietary protein on cholesterol homeostasis in diabetic rats. J Lipid Res. 29:1272–1287.
- Kulkarni CR, Joglekar MM, Patil SB, Arvindekar AU. 2012. Antihyperglycemic and antihyperlipidemic effect of Santalumalbum in streptozotocin induced diabetic rats. Pharm Biol. 50:360–365.
- Lee S, Tsao R, Peterson C, Coats JR. 1997. Insecticidal activity of monoterpenoids to western corn rootworm (Coleoptera: Chrysomelidae) two-spotted spider mite (Acari: Tetranychidae), and house fly (Diptera: Muscidae). J Econ Entomol. 90:883–892.
- Manou L, Bouillard L, Devleeschouwer MJ, Barel AO. 1998. Evaluation of the preservative properties of Thymus vulgaris essential oil in topically applied formulations under a challenge test. J Appl Microbiol. 84:368–376.
- Marcio RVS, Flávia VM, Byanka PF, Damiao PDS, Leonardo RB, Lucindo JQJ. 2011. Cardiovascular effects of monoterpenes: a review. Rev Bras Farmacogn Braz J Pharmacogn. 21:764–771.
- Lindmark-Henriksson M, Isaksson D, Vanek T, Valterová I, Högberg HE, Sjödin K. 2004. Transformation of terpenes using a Picea abies suspension culture. J Biotechnol. 107:173–184.
- Moler DE. 2001. New drug targets for type 2 diabetes and metabolic syndrome. Nature. 414:821–827.
- Mooradian AD. 2009. Dyslipidemia in type 2 diabetes mellitus. Nat Clin Pract Endocrinol Metab. 5:150–159.
- Murali R, Saravanan R. 2012. Antidiabetic effect of d-limonene, a monoterpene in streptozotocin-induced diabetic rats. Biomed Prev Nutr. 2:269–275.
- Nielsen LB, Bartels ED, Bollana E. 2002. Over expression of apolipoprotein B in the heart impedes cardiac triglyceride accumulation and development of cardiac dysfunction in diabetic mice. J Biol Chem. 277:14–20.
- Okutan H, Ozcelik N, Yilmaz HR, Uz E. 2005. Effects of caffeic acid phenethyl ester on lipid peroxidation and antioxidant enzymes in diabetic rat heart. Clin Biochem. 38:191–196.
- Peixoto-Neves D, Silva-Alves KS, Gomes MD, Lima FC, Lahlou S, Magalhães PJ, Ceccatto VM, Coelho-de-Souza AN, Leal-Cardoso JH. 2010. Vasorelaxant effects of the monoterpenic phenol isomers, carvacrol and thymol, on rat isolated aorta. Fundam Clin Pharmacol. 24:341–350.
- Philipp B, Shapiro DJ. 1970. Improved methods for the assay and activation of 3-hydroxy-3-methylglutaryl coenzyme A reductase. J Lipid Res. 20:588–593.
- Punithavathi VR, Prince PS, Kumar R, Selvakumari J. 2011. Antihyperglycaemic, antilipid peroxidative and antioxidant effects of gallic acid on streptozotocin induced diabetic Wistar rats. Eur J Pharmacol. 650:465–471.
- Ramachandran V, Saravanan R. 2013. Efficacy of asiatic acid, a pentacyclic triterpene on attenuating the key enzymes activities of carbohydrate metabolism in streptozotocin-induced diabetic rats. Phytomedicine. 20:230–236.
- Rathinam A, Pari L, Chandramohan R, Sheikh BA. 2014. Histopathological findings of the pancreas, liver, and carbohydrate metabolizing enzymes in STZ-induced diabetic rats improved by administration of myrtenal. J Physiol Biochem. 70:935–946.
- Shepherd J. 2005. Does statin monotherapy address the multiple lipid abnormalities in type 2 diabetes? Atheroscler Suppl. 6:15–19.
- Singh IM, Shishehbor MH, Ansell BJ. 2007. High-density lipoprotein as a therapeutic target: a systematic review. J Am Med Assoc. 298:786–798.
- Tan BK, Tan CH, Pushparaj PN. 2005. Anti-diabetic activity of the semi-purified fractions of Averrhoa bilimbi in high fat diet fed-streptozotocin-induced diabetic rats. Life Sci. 76:2827–2839.
- Taskinen MR. 1987. Lipoprotein lipase in diabetes. Diabetes Metab Rev. 3:551–570.
- Vance JE, Vance DE. 2005. Metabolic insights into phospholipid function using gene targeted mice. J Biol Chem. 280:10877–10880.
- Verges B. 2009. Lipid modification in type 2 diabetes: the role of LDL and HDL. Fundam Clin Pharmacol. 23:681–685.
- Vibha JB, Choudhary K, Mangal S, Rathore MS, Shekhawat NS. 2009. A study on pharmacokinetics and therapeutic efficacy of Glycyrrhiza glabra. A miracle medicinal herb. Bot Res Int. 2:157–163.
- Zilversmit BB, Davis AK. 1950. Microdetermination of plasma phospholipids by trichloroacetic acid precipitation. J Lab Clin Med. 35:155–160.
- Zlatkis A, Zak B, Boyle GJ. 1953. A simple method for determination of serum cholesterol. J Clin Med Res. 41:486–492.