Abstract
Context: Triterpenes from Poria cocos Wolf (Polyporaceae) have been used to treat various diseases in traditional Chinese medicine. However, the antiepileptic effects and mechanism are not fully understood.
Objective: The objective of this study is to investigate the antiepileptic properties of total triterpenes (TTP) from the whole P. cocos.
Materials and methods: The ethanol extract TTP was identified by HPLC fingerprint analysis. Male ICR mice were gavaged (i.g.) with TTP (5, 20, 80 or 160 mg/kg) or reference drugs twice a day for 7 d. Antiepileptic activities of TTP were evaluated by maximal electroshock (MES)- and pentylenetetrazole (PTZ)-induced seizures in mice for 30 and 60 min, respectively. Locomotor activity and Rota-rod tests were performed for 60 min and 5 min, respectively. The levels of glutamic acid (Glu), aspartic acid (Asp), γ-aminobutyric acid (GABA) and glycine (Gly) in convulsive mice were estimated. The chronic epileptic model of Wistar rats was built to measure expressions of glutamate decarboxylase 65 (GAD65) and GABAA in rat brain after TTP treatment.
Results: The LC50 of TTP (i.g.) was above 6 g/kg. TTP (5–160 mg/kg) protected mice against MES- and PTZ-induced convulsions at 65.0% and 62.5%, respectively, but have no effect on rota-rod treadmill; TTP (20–160 mg/kg) significantly reduced the locomotor activities, shortened the onset of pentobarbital sodium-induced sleep; TTP decreased Glu and Asp levels in convulsive mice, but increased the GAD65 and GABAA expressions in chronic epileptic rats at doses usage.
Discussion and conclusion: TTP extracted from P. cocos possessed potential antiepileptic properties and is a candidate for further antiepileptic drug development.
Introduction
Epilepsy is one of the most prevalent and serious brain disorders, which can occur during many possible conditions with recurrent spontaneous seizures (Forsgren et al. Citation2005; Duncan et al. Citation2006). Nearly 65 million people worldwide suffer from this disease until now (Moshe et al. Citation2015). Patients with epilepsy are characterized by depression, anxiety, convulsions, and even loss of consciousness (Clancy et al. Citation2014; Maguire et al. Citation2014). Although efficient antiepileptic drugs and surgical procedures have improved during the past decade, these treatments are still unaffordable for most patients due to the high cost (Benamer & Grosset Citation2009; Newton & Garcia Citation2012; Beghi & Hesdorffer Citation2014). Furthermore, nearly all antiepileptic drugs have safety concerns and side effects (Malagon-Valdez Citation2004; Kaufman Citation2011), and an increasing number of patients are drug resistant (Noam et al. Citation2013; Laxer et al. Citation2014). Therefore, it is urgent to find novel therapeutic agents with few adverse effects. Poria cocos Wolf (Polyporaceae) is a saprophytic fungus that grows on the roots of pine trees in China and other Asian countries. Dried sclerotia of P. cocos, called Fuling, are widely used as a valuable medicine in traditional Chinese prescriptions (Song et al. Citation2011; Kang et al. Citation2015; Ueki et al. Citation2015). In the past decades, researchers from China and Japan have isolated the major known compounds from different parts of P. cocos and extensively investigated their biological activities. Previous studies have demonstrated that the surface layer of P. cocos has a remarkable diuretic effect (Zhao et al. Citation2012; Feng et al. Citation2013a), which has been tested for treatment of early renal injury and chronic kidney disease in rats (Zhao et al. Citation2013a–c); Furthermore, dried sclerotia of P. cocos was verified to have anti-inflammatory and hypoglycemic effects in prediabetes mellitus rats (Lee et al. Citation2014). Triterpenes as the major active compounds of P. cocos are mainly derived from a lanostane skeleton (Rios Citation2011), which have been reported to manage psoriasis, rheumatoid arthritis, septic shock, autoimmune uveitis and bronchial asthma (Cuellar et al. Citation1997; Giner et al. Citation2000; Spelman et al. Citation2006). However, the antiepileptic effects of triterpenes as well as underlying mechanisms remain to be determined.
Our study focused on the antiepileptic activities of total triterpenes (TTP) isolated from P. cocos as well as the underlying benefits and molecular mechanisms that involve specific amino acids in the brain. Therefore, our investigation may provide a scientific basis for understanding potential uses of TTP in drug development.
Methods and materials
Plant material
The dried whole plant P. cocos was purchased from the Traditional Chinese Herbs Market in Xi’an, China and identified by Prof. Peng Lei (Department of Traditional Chinese Medicine, Shannxi Provincial People’s Hospital). A voucher specimen (No. 25021) was deposited at our laboratory.
Preparation of the extract
To prepare TTP, the dried whole plant of P. cocos (1 kg) was ground into fine powder by disintegrator and extracted three times with 6 L volumes of 95% ethanol for 30 min by ultrasonic method, the extracts were combined together and filtered with 0.45 μm filter. Then the filtrate was concentrated under reduced pressure and lyophilized by vacuum evaporation to yield dried powder. The solid powder (4.2 g) was diluted in normal saline (0.9%) for further preparation.
High-performance liquid chromatography (HPLC) fingerprint analysis
The chromatographic system we used includes a Shimadzu LC-20AT system (Shimadzu Corporation, Kyoto, Japan) equipped with SPD-M20A UV-diode array detector and Windows LC solution software (Shimadzu Corporation, Kyoto, Japan), column, VP-ODS 5 μm and dimensions (4.6 × 250 mm). Chromatographic conditions include a mobile phase: solvent A, water; solvent B, acetonitrile; flow rate 1 mL/min; and detection UV 242 nm. The HPLC solvent gradients were 0.01 min, solvent B 40%; 1–25 min, solvent B 56%; 26–45 min, solvent B 67%; 46–70 min, solvent B 67%; 76–100 min, solvent B 100%. The column oven temperature was 30 °C.
Drugs and chemicals
Pentylenetetrazole (PTZ) and phenobarbital sodium were purchased from Sigma Chemical Co. (St. Louis, MO) and diazepam (DZP) was obtained from Roche Product Ltd. (Welwyn Garden City, UK). The standard agents of aspartic acid (Asp), γ-aminobutyric acid (GABA), glutamic acid (Glu) and glycine (Gly) were purchased from the National Institutes for Food and Drug Control (Beijing, China). Glutamate decarboxylase 65 (GAD65) and GABAA monoclonal antibodies, goat anti-rabbit/HRP antibody and β-actin antibody were purchased from Abcam Co. (Shanghai, China). All other chemicals were of analytical reagent grade.
Animals
Male ICR mice (18–22 g) and Wistar rats (180–220 g) were obtained from the Animal Center of Shaanxi Provincial People’s Hospital, Xi’an, China. Animals were housed at 25 °C with a 12 h light/dark cycle and provided with standard laboratory food and water ad libitum. The animal experiments were in accordance with the Animal Care and Use Committee of Shaanxi Provincial People’s Hospital and in compliance with the Chinese National Institute of Health Guidelines for Care and Use of Laboratory Animals.
Measurement of locomotor activity
Locomotor activity of mice was assessed according to the method described previously (Chindo et al. Citation2014). Mice were divided into six groups of 20 mice. For mice in the experimental group, the following treatments were administered by gavage (i.g.) twice a day for 7 d: normal saline (20 mL/kg), DZP (1 mg/kg) or TTP at dose of 5, 20, 80 or 160 mg/kg. We used a photoelectric spontaneous locomotor activity apparatus with four identical boxes (60 × 60 × 40 cm). Each mouse was placed individually in the centre of a box for 5 min. Movement of the mouse interrupted the beam of infrared light emitted from the frame of the apparatus. The number of activities was recorded by the apparatus for 60 min.
Rota-rod test in mice
Rota-rod test was used to assess the motor performance in mice with a rotarod apparatus (Rotarod Treadmill 755, Beijing, China), the diameter of revolving rods was 3 cm. Mice were trained for 5 d to remain on a treadmill device with slowly rotate speed at 10 rpm. During this training process, mice that had an unstable variations were eliminated, and the rest of mice were divided into five groups of 20 mice per group and treated as follows: TTP at doses of 5, 20, 80 or 160 mg/kg, and normal saline (20 mL/kg, i.g.), twice a day for 7 d and continued training during drug administration. One hour after the last medicine administration, the mice were placed individually on the rod at the speed of 20 rpm for 5 min. The latency time was recorded as the animal mounted the rod until it fell off.
Pentobarbital sodium-induced sleep in mice
Pentobarbital sodium-induced sleep was performed in six groups of 20 mice. Mice received TTP (5, 20, 80 or 160 mg/kg, i.g.), normal saline (20 mL/kg, i.g.) or DZP (1 mg/kg, i.g.) twice a day for 7 d. Pentobarbital sodium (50 mg/kg, i.p.) was given to all mice 30 min after drug administration. Mice were observed for loss of the “righting reflex” (Wambebe Citation1985), which was indicated by inability to return to the upright position on all four limbs after the animal was placed on its back. The interval between loss and recovery of the righting reflex was recorded as the duration of sleep.
Maximal electroshock (MES)-induced seizures
MES-induced seizures experiment was advanced by using pharmacological and physiological multi-experimental instrument YSD-4G (Zhenghua Company, Banghu, China) in a single stimulation way, two-clip electrodes was impregnated with saline and then used to clamp the tips of mice ear on both sides, an alternating current stimulus (100 V; 2 Hz) was set. Mice was performed in six groups of 20 mice and pretreated with TTP (5, 20, 80 or 160 mg/kg, i.g.), normal saline (20 mL/kg, i.g.) or phenytoin (4 mg/kg, i.g.) twice a day for 7 d. Electroshock was given 30 min after drug administration. The feature of seizures was recorded as animal hind limb tonic extension (HLTE) within 10 s after electroshock delivery, and the criterion for an anticonvulsant effect was the absence of an HLTE and the later tonic-clonic seizures. The number of mortality was observed within 24 h.
Pentylenetetrazole (PTZ) and picrotoxin-induced seizures in mice
The pentylenetetrazole (PTZ) and picrotoxin-induced convulsion test described previously was modified (Ahmadiani et al. Citation2003) and used to induce convulsions in mice. Mice were divided into six groups of 20 mice and pretreated with TTP (5, 20, 80 and 160 mg/kg, i.g.), normal saline (20 mL/kg, i.g.) or DZP (1 mg/kg, i.g.) twice a day for 7 d. After 30 min, PTZ (90 mg/kg) was administered subcutaneously to all groups of mice. Mice were observed within 60 min, and scored according to the five different types of seizure stage described by the Racine scale (Luttjohann et al. Citation2009): (stage I) one or more mouth and facial twitches; (stage II) rhythmic head nodding; (stage III) forelimb clonus; (stage IV) seizures characterized by rearing or hind limb extension; (stage V) seizures with rearing and repeated falling.
Measurement of Asp, GABA, Glu and Gly content in the brain
PTZ-induced convulsions in mice were conducted as described above. Mice were divided into five groups (n = 20 for each group) and pretreated with TTP (5, 20, 80 or 160 mg/kg, i.g.) or normal saline (20 mL/kg, i.g.) twice a day for 7 d. Mice were killed 50 min after administration of PTZ or saline and the whole brain was collected. Brains were removed rapidly, weighed and then homogenized and centrifuged at 12,000 × g for 10 min at 4 °C. Asp, GABA, Glu and Gly content in the brain were detected by Beckman 6300 high performance amino acid analyzer (Beckman Coulter, Brea, CA).
Chronic epileptic rat seizures induced by PTZ and Western blotting
A PTZ kindling model was used to establish chronic epileptic rats according to the method described previously (Ruthrich et al. Citation2001). A total of 40 Wistar rats were divided into five groups (n = 8 for each group): a control group and four TTP experimental groups (5, 20, 80 or 160 mg/kg, i.g.). PTZ (40 mg/kg, i.p.) was administered once a day for 4 weeks. Criteria for establishing the chronic epileptic model by PTZ kindling were “strong jerking and jumping, and shouting” (Yang et al. Citation2013). The chronic epileptic rats were pretreated with normal saline (20 mL/kg, i.g.) and TTP (5, 20, 80 or 160 mg/kg, i.g.) twice a day for 21 d. The rats were then anesthetized, killed and whole brains were collected. Total protein was extracted from brain tissue, and equal amounts of protein (30 μg) were separated by SDS-PAGE and transferred to PVDF. The membranes were blocked with 5% non-fat milk and then incubated with anti-GAD65 and anti-GABAA rabbit monoclonal IgG (1:1000) followed by goat anti-rabbit/HRP. Bands were detected by enhanced chemiluminescence and quantified using Quantity One software (Bio-Rad Laboratories, Hercules, CA). Antibodies against β-actin were used to measure protein loading.
Acute toxicity
Three groups each six mice were treated i.g. with the TTP (at dose of 2, 4 and 6 g/kg) twice a day, then the intraperitoneal median lethal dose (LD50) was determined after 7 d.
Statistical analysis
The Chi-square exact test was used to analyze significance of anticonvulsant effects against MES- and PTZ-induced seizures among groups (inhibition/% and mortality/%). All other data are presented as mean ± SD, and statistical analyses were performed using the two-tailed Student’s t-test with a significance level of p < 0.05.
Results
HPLC fingerprint of triterpene acids of P. cocos
Preliminary phytochemical analysis revealed the HPLC fingerprint of triterpene acids isolated from P. cocos recorded at 242 nm (). The major compounds in the extract were identified as polyporenic acid C (peak 1), pachymic acid (peak 2), dehydrotrametenolic acid (peak 3) and trametenolic acid (peak 4) when compared with the data obtained from ElectroSpray Ionization-Mass Spectrometry (ESI-MS) with those studies published previously: Taken peak 1 compound as the example, it had ESI-MS m/z: 480 [M-H]− and showed a molecular ion at m/z 481, which was in agreement with the molecular formula C31H45O4 and identified as polyporenic acid C (Tai et al. Citation1993; Chen et al. Citation2009; Feng et al. Citation2013b).
Acute toxicity study
Lethal effects were not observed after the i.g. administration of the TTP extract at doses of 2, 4 and 6 g/kg. The i.g. LD50 value of TTP in mice was estimated to be greater than 6 g/kg, indicating that TTP is obviously safe when administered intragastrically to mice.
Locomotor activity
No abnormal appearance among the different groups of mice was observed during the initial 3 d of treatment of different drugs. However, with the process of treatment continuing, we observed that mice in the TTP group (160 mg/kg) and the DZP group gradually had less activities at night, whereas their sleeping time was extensively prolonged. After the administration of different drugs for 7 d, results of the locomotor activity test were recorded as in . As expected, DZP as the positive control drug significantly decreased the total mobile and rearing activities of mice (p < 0.01). In addition, animals in TTP groups also significantly decreased locomotor activities at doses of 20, 80 and 160 mg/kg (p < 0.05, p < 0.01 and p < 0.01, respectively) compared with negative control mice (). Meanwhile, the average activity number of TTP at dose of 160 mg/kg approached to DZP group (1 mg/kg), which indicated the similar sedative effect between TTP (160 mg/kg) and DZP (1 mg/kg).
Figure 2. Effects of TTP on locomotor activity in mice. (A) Total mobile counts. (B) Total rearing counts. DZP was used as a positive control. Mice were divided into six groups (n = 10): control group (normal saline, 20 mL/kg, i.g.), positive group (DZP, 1 mg/kg) and four experimental groups (TTP at dose of 20, 80 and 160 mg/kg, respectively). Values are means ± SD (n = 20), *p < 0.05, **p < 0.01 when compared with the control.
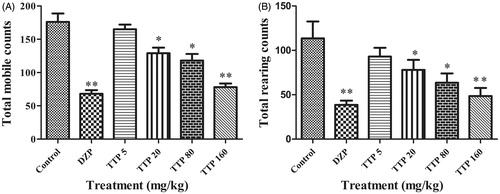
Rota-rod test in mice
This experiment was carried out to measure the motor coordinating and balance of mice on the rotarod. In our preliminary experiment, we found that most mice were easily able to maintain balance on the rotarod for more than 5 min at the speed of 10 rpm. When the speed increased to 20 rpm, as illustrated in , animals received TTP at the doses of 5, 20, 80 or 160 mg/kg had no effect on the body balance and motor coordination when compared with the control group. The average time of the latency to fall off was about 160.7 s for each group during 5-min observation.
Figure 3. Effects of TTP on Rota-rod test in mice. Mice were divided into five groups (n = 10): control group (normal saline, 20 mL/kg, i.g.) and four experimental groups (TTP at dose of 5, 20, 80 and 160 mg/kg, respectively). Values are means ± SD (n = 20), *p < 0.05, **p < 0.01 when compared with the control.
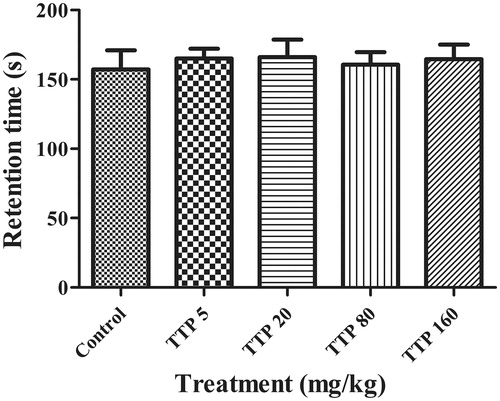
Pentobarbital sodium-induced sleep in mice
To further determine the sedative effect of TTP, pentobarbital sodium-induced sleeping time was analyzed in mice with treatment of different drugs. We observed that mice in the DZP group first lost “righting reflex” and went into sleep-mode with the shortest time (p < 0.01); the onset of sleep time was also significantly decreased by the administration with TTP at doses of 20, 80 and 160 mg/kg (p < 0.05, p < 0.05 and p < 0.01, respectively) compared with the control group (). However, no onset of sleep time was changed when mice treated with TTP at dose of 5 mg/kg. In addition, the duration of sleep was significantly prolonged in animals treated with DZP (p < 0.01); TTP at doses of 80 and 160 mg/kg also prolonged sleeping time (p < 0.01 and p < 0.01, respectively) compared with the control group (). Whereas mice in TTP at doses of 5, 20 mg/kg and control groups quickly recovered the “righting reflex”.
Figure 4. Effects of TTP on the analysis of pentobarbital sodium-induced sleeping time in mice. (A) Duration of mice sleep. (B) The onset of mice sleep. DZP was used as a positive control. Mice were divided into six groups (n = 10): control group (normal saline, 20 mL/kg, i.g.), positive group (DZP, 1 mg/kg) and four experimental groups (TTP at dose of 5, 20, 80 and 160 mg/kg, respectively). Values are means ± SD (n = 20), *p < 0.05, **p < 0.01 when compared with the control.
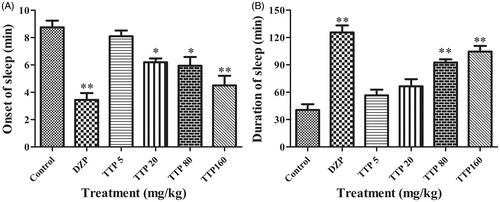
MES-induced seizures
As shown in , all mice in the control group convulsed and eight mice died. The convulsive inhibition of TTP at dose of 5 mg/kg was only 20% and the mortality rate was 25%, which meant have no anticonvulsant effects against MES-induced seizures; whereas compared with the control group, TTP at doses of 20, 80 and 160 mg/kg showed significant highly anticonvulsant effects with convulsive inhibitions at 50%, 80% and 100% (p < 0.05, p < 0.01 and p < 0.01, respectively), and the tonic-clonus rates in these experimental groups were relatively reduced (p < 0.05, p < 0.01 and p < 0.01, respectively). As a result, the mortality rates among TTP at doses of 20, 80 and 160 mg/kg groups were remarkably decreased (p < 0.01, p < 0.01 and p < 0.01, respectively). In addition, no convulsions or deaths were observed in the positive control group when treated with phenytoin (4 mg/kg, i.g.).
Table 1. Effect of TTP on tonic seizures induced by maximal electroshock in male mice (n = 20).
PTZ-induced seizures in mice
To confirm the antiepileptic effects of TTP, a PTZ-induced seizure mice model was established. As shown in , all mice in the control group convulsed and finally died. However, TTP at doses of 5, 20, 80 and 160 mg/kg showed significant anticonvulsant effects, the inhibitions of stages IV–V were 40, 60, 75 and 85% (p < 0.05, p < 0.01, p < 0.01 and p < 0.01, respectively) and the inhibitions of stage V were 45, 75, 85 and 95% (p < 0.05, p < 0.01, p < 0.01 and p < 0.01, respectively). Furthermore, mortality at doses of 5, 20, 80 and 160 mg/kg were 55, 45, 25 and 10%, respectively (p < 0.05, p < 0.01, p < 0.01 and p < 0.01, respectively). In addition, no convulsions or deaths were observed in mice treated with DZP (1 mg/kg).
Table 2. Effect of TTP on pentylentertrazole (PTZ)-induced seizures in mice (n = 20).
Measurement of Asp, GABA, Glu and Gly content in the mouse brain
showed that PTZ-induced convulsions could significantly increase the content of Glu in mice brain (p < 0.01), whereas when treatment with TTP in convulsive mice significantly decreased Glu content at doses of 5, 20, 80 and 160 mg/kg (p < 0.05, p < 0.05, p < 0.01 and p < 0.01, respectively); Furthermore, Asp content was also up-regulated in convulsive mice but the difference was not obvious (p > 0.01) when compared with the normal group. The level of Asp was significantly decreased by treatment with TTP at doses of 80 and 160 mg/kg (p < 0.05 and p < 0.05, respectively) when compared with the control group. In contrast, a distinct decrease of GABA content was detected in convulsive mice (p < 0.01) when compared with normal mice. After treatment with TTP at doses of 80 and 160 mg/kg, GABA content in convulsive mice increased significantly (p < 0.05 and p < 0.05, respectively). However, no change was observed about Gly content in both normal and convulsive groups, neither showed difference among all TTP treatment groups.
Figure 5. Effects of TTP on the Asp, GABA, Glu and Gly level of mice brain. (A) The contents of Glu. (B) The contents of GABA. (C) The contents of Asp. (D) The contents of Glu. Six groups (n = 10) of mice were treated as follows: normal group treated with no PTZ (normal saline, 20 mL/kg), control group (normal saline, 20 mL/kg) and four experimental TTP groups (5, 20, 80 and 160 mg/kg). Values are means ± SD (n = 20), *p < 0.05, **p < 0.01 when compared with the control.
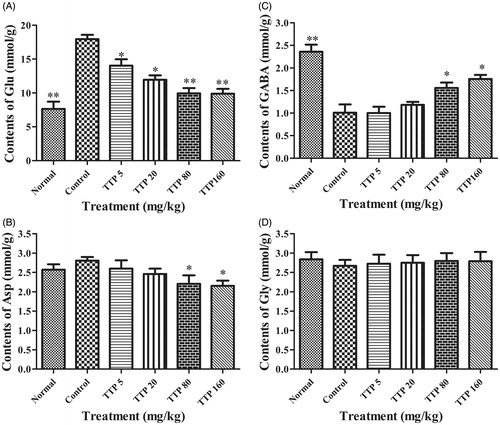
Effect of TTP on expression of GAD65 and GABAA in the brain of epileptic rats
Our results indicated that chronic epileptic rat seizures induced by PTZ could up-regulate the expression of GABAA (p < 0.05) when compared with the normal group; when treated with TTP at doses of 20, 80 and 160 mg/kg, the level of GAD65 was significantly increased in the brains of epileptic rats compared with the control group (p < 0.05, p < 0.01 and p < 0.01, respectively); furthermore, the expression levels of GABAA were also significantly upregulated in TTP groups at the doses of 5, 20, 80 and 160 mg/kg compared with the control group (p < 0.05, p < 0.01, p < 0.01 and p < 0.01, respectively) ().
Figure 6. Effects of TTP on GAD65 and GABAA expression in the brain of epileptic rats. (A) Western blot analysis of expressions of GAD65 and GABAA. β-Actin was considered as an internal control. (B) Quantitation of GAD65 expression in (A). (C) Quantitation of GABAA expression in (A). Six groups (n = 10) of rat were treated as follows: normal group without any treatment, control group (normal saline, 20 mL/kg), and four experimental TTP groups (5, 20, 80 and 160 mg/kg). Values are means ± SD (n = 8), *p < 0.05, **p < 0.01 when compared with the control.
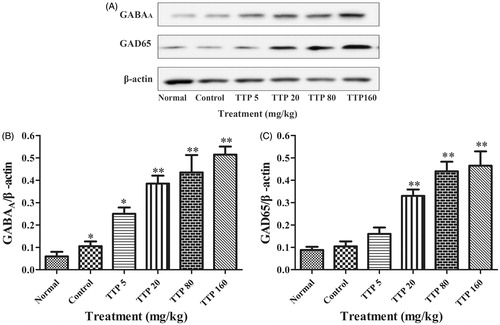
Discussion
Plant-derived compounds have been reported to therapeutically treat various diseases and have satisfied effects in China (Chan et al. Citation2012). To the best of our knowledge, our present study was the first to demonstrate that TTP extracted from P. cocos has significant antiepileptic effects and the mechanism may be closely involved in up-regulating levels of inhibitory neurotransmitter GABA and down-regulating levels of excitatory neurotransmitter Glu.
Locomotor activity and pentobarbital sodium-induced sleeping time analyses are sensitive models for detecting clinical sedation (Garzon-De la Mora et al. Citation1999). Treatment with different concentrations of triterpene acids significantly decreased locomotor activity, which indicates its crucial sedative property. This finding was confirmed by the ability of TTP to significantly prolong pentobarbital sodium-induced sleeping time and shorten sleep latency. Thus, we conclude that triterpene acids have satisfactory and reliable anticonvulsant and sedative effects. Ratarods tests are now commonly used and latency to fall off is thus a more stringent measure of ability to maintain balance (Chindo et al. Citation2014). Our study demonstrated that the TTP at the doses used had no influence on the motor coordination and body balance in mice, suggesting that the sedative effects of TTP extract might play a role through central mechanisms but not peripheral neuromuscular blockade (Perez et al. Citation1998).
The MES test is a classic animal model for evaluating antiepileptic activity against generalized tonic-clonic seizures. The PTZ test is a valid model for human generalized myoclonic and absence seizures (Krall et al. Citation1978; Blanco et al. Citation2009; Temkin Citation2009). The pathophysiology of epilepsy is thought to occur if the balance between excitatory and inhibitory neurotransmitters is altered (Madsen et al. Citation2010). GABA is a vital inhibitory neurotransmitter in the brain and contributes to the development of epilepsy (Schousboe et al. Citation2011). Previous studies have shown that PTZ induces seizures by blocking the major inhibitory pathways of GABA (Takechi et al. Citation2012), and standard antiepileptic agents such as DZP may exert their effects by increasing GABA-mediated inhibition in the brain (Miczek et al. Citation2002).
The results of our study demonstrated that triterpene acids significantly inhibited seizures induced by both MES and PTZ, which suggests that triterpene acids may achieve antiepileptic effects through modulation of GABA. In addition to GABA, there are several other endogenous neurotransmitters that play important roles in sleep mechanisms such as dopamine, acetylcholine, histamine, serotonin and neuropeptides (Murillo-Rodriguez et al. Citation2012). Glu, which is an excitatory neurotransmitter, plays an opposite role to GABA in the central nervous system (Albrecht et al. Citation2010). Therefore, an imbalance of GABA/Glu in the brain could cause epilepsy (Bonanno et al. Citation2006). In our study, we found that treatment with different doses of TTP significantly increased GABA content, whereas Glu content significantly decreased.
Glutamate decarboxylase (GAD) is responsible for GABA synthesis and has been reported to be connected with epilepsy (Alexopoulos & Dalakas Citation2013). GAD65 is an isoform of GAD and can inhibit sensitivity to epilepsy and improve neuropathological changes in epileptic mice (Awad et al. Citation2009; Xiang & Jiang Citation2013). In addition, GABAA, which is the most important receptor for GABA, is thought to be an important target for new antiepileptic drug development (Enell et al. Citation2007; Greenfield Citation2013). In our study, we found that treatment with different concentrations of TTP upregulated the expressions of GAD65 and GABA in chronic epileptic rat, which may be the underlying mechanisms through which triterpene acids treat epilepsy.
Poria cocos contains two principal groups of chemicals, the polysaccharides and the triterpenes (Rios Citation2011). In our study, we extracted TTP both from the epidermis (Fulingpi in Chinese) and the inner part of P. cocos (Baifuling in Chinese). Although the major chemical compositions of TTP from Fulingpi and Baifuling were dehydrotumulosic acid, dehydrotrametenolic acid, trametenolic acid and poricoic acid A, the content of TTP in Fulingpi is 10 times higher than that in Baifuling (Dong et al. Citation2015; Wang et al. Citation2015). Recently, by using advanced separation technology, nearly 40 similar chemical structures of triterpenes have been isolated from P. cocos (Rios Citation2011). In order to fully distinguish and elucidate the exact role of each type of triterpenes in the treatment of epilepsy, further investigations and novel methods are required.
In conclusion, triterpene acids extracted from P. cocos have significant antiepileptic effects and can regulate GABA and Glu content in the brain, as well as the expressions of GAD65 and GABA. Thus, our data indicate that triterpene acids isolated from P. cocos may represent promising therapeutic agents for treatment of epilepsy.
Acknowledgements
The authors are thankful to Professor Jing Zhang and Professor Lei Wang for their instruction in our research project.
Disclosure statement
The authors report no declarations of interest.
References
- Ahmadiani A, Mandgary A, Sayyah M. 2003. Anticonvulsant effect of flutamide on seizures induced by pentylenetetrazole: involvement of benzodiazepine receptors. Epilepsia. 44:629–635.
- Albrecht J, Sidoryk-Wegrzynowicz M, Zielinska M, Aschner M. 2010. Roles of glutamine in neurotransmission. Neuron Glia Biol. 6:263–276.
- Alexopoulos H, Dalakas MC. 2013. Immunology of stiff person syndrome and other GAD-associated neurological disorders. Expert Rev Clin Immunol. 9:1043–1053.
- Awad R, Ahmed F, Bourbonnais-Spear N, Mullally M, Ta CA, Tang A, Merali Z, Maquin P, Caal F, Cal V, et al. 2009. Ethnopharmacology of Q'eqchi' Maya antiepileptic and anxiolytic plants: effects on the GABAergic system. J Ethnopharmacol. 125:257–264.
- Beghi E, Hesdorffer D. 2014. Prevalence of epilepsy – an unknown quantity. Epilepsia. 55:963–967.
- Benamer HT, Grosset DG. 2009. A systematic review of the epidemiology of epilepsy in Arab countries. Epilepsia. 50:2301–2304.
- Blanco MM, dos Santos JG Jr, Perez-Mendes P, Kohek SR, Cavarsan CF, Hummel M, Albuquerque C, Mello LE. 2009. Assessment of seizure susceptibility in pilocarpine epileptic and nonepileptic Wistar rats and of seizure reinduction with pentylenetetrazole and electroshock models. Epilepsia. 50:824–831.
- Bonanno G, Raiteri L, Paluzzi S, Zappettini S, Usai C, Raiteri M. 2006. Co-existence of GABA and Glu transporters in the central nervous system. Curr Top Med Chem. 6:979–988.
- Chan K, Shaw D, Simmonds M, Leon CJ, Xu Q, Lu A, Sutherland I, Ignatova S, Zhu YP, Verpoorte R, et al. 2012. Good practice in reviewing and publishing studies on herbal medicine, with special emphasis on traditional Chinese medicine and Chinese materia medica. J Ethnopharmacol. 140:469–475.
- Chen L, Wang D, Wu J, Yu B, Zhu D. 2009. Identification of multiple constituents in the traditional Chinese medicine formula GuiZhiFuLing-Wan by HPLC-DAD-MS/MS. J Pharm Biomed Anal. 49:267–275.
- Chindo BA, Ya UJ, Danjuma NM, Okhale SE, Gamaniel KS, Becker A. 2014. Behavioral and anticonvulsant effects of the standardized extract of Ficus platyphylla stem bark. J Ethnopharmacol. 154:351–360.
- Clancy MJ, Clarke MC, Connor DJ, Cannon M, Cotter DR. 2014. The prevalence of psychosis in epilepsy; a systematic review and meta-analysis. BMC Psychiatry. 14:75.
- Cuellar MJ, Giner RM, Recio MC, Just MJ, Mañez S, Rios JL. 1997. Effect of the basidiomycete Poria cocos on experimental dermatitis and other inflammatory conditions. Chem Pharm Bull. 45:492–494.
- Dong H, Wu P, Yan R, Xu Q, Li H, Zhang F, Li J, Yang B. 2015. Enrichment and separation of antitumor triterpene acids from the epidermis of Poria cocos by pH-zone-refining counter-current chromatography and conventional high-speed counter-current chromatography. J Sep Sci. 38:1977–1982.
- Duncan JS, Sander JW, Sisodiya SM, Walker MC. 2006. Adult epilepsy. Lancet. 367:1087–1100.
- Enell L, Hamasaka Y, Kolodziejczyk A, Nässel DR. 2007. Gamma-Aminobutyric acid (GABA) signaling components in Drosophila: immunocytochemical localization of GABA(B) receptors in relation to the GABA(A) receptor subunit RDL and a vesicular GABA transporter. J Comp Neurol. 505:18–31.
- Feng YL, Lei P, Tian T, Yin L, Chen DQ, Chen H, Mei Q, Zhao YY, Lin RC. 2013a. Diuretic activity of some fractions of the epidermis of Poria cocos. J Ethnopharmacol. 150:1114–1118.
- Feng YL, Zhao YY, Ding F, Xi ZH, Tian T, Zhou F, Du X, Chen DQ, Wei F, Cheng XL, et al. 2013b. Chemical constituents of surface layer of Poria cocos and their pharmacological properties (I). Zhongguo Zhong Yao Za Zhi. 38:1098–1102.
- Forsgren L, Hauser WA, Olafsson E, Sander JW, Sillanpää M, Tomson T. 2005. Mortality of epilepsy in developed countries: a review. Epilepsia. 46:18–27.
- Garzon-Dela Mora P, Garcia-Lopez PM, Garcia-Estrada J, Navarro-Ruíz A, Villanueva-Michel T, Villarreal-de Puga LM, Casillass-Ochoa J. 1999. Casimiroa edulis seed extracts show anticonvulsive properties in rats. J Ethnopharmacol. 68:275–282.
- Giner EM, Manez S, Recio MC, Giner RM, Cerdá-Nicolás M, Ríos JL. 2000. In vivo studies on the anti-inflammatory activity of pachymic and dehydrotumulosic acids. Planta Med. 66:221–227.
- Greenfield LJ Jr. 2013. Molecular mechanisms of antiseizure drug activity at GABAA receptors. Seizure. 22:589–600.
- Kang BK, Park TY, Lee JA, Moon TW, Lee MS. 2015. Factor analysis on symptoms and signs of stroke based on traditional Korean Medicine theory. Int J Clin Exp Med. 8:949–960.
- Kaufman KR. 2011. Antiepileptic drugs in the treatment of psychiatric disorders. Epilepsy Behav. 21:1–11.
- Krall RL, Penry JK, White BG, Kupferberg HJ, Swinyard EA. 1978. Antiepileptic drug development: II. Anticonvulsant drug screening. Epilepsia. 19:409–428.
- Laxer KD, Trinka E, Hirsch LJ, Cendes F, Langfitt J, Delanty N, Resnick T, Benbadis SR. 2014. The consequences of refractory epilepsy and its treatment. Epilepsy Behav. 37:59–70.
- Lee HC, Cheng WY, Huang BETG, Hsu YH, Huang SY. 2014. Anti-inflammatory and hypoglycemic efficacy of Poria cocos and Dioscorea opposita in prediabetes mellitus rats. RSC Adv. 4:55649–55657.
- Luttjohann A, Fabene PF, van Luijtelaar G. 2009. A revised Racine's scale for PTZ-induced seizures in rats. Physiol Behav. 98:579–586.
- Madsen KK, White HS, Schousboe A. 2010. Neuronal and non-neuronal GABA transporters as targets for antiepileptic drugs. Pharmacol Ther. 125:394–401.
- Maguire MJ, Weston J, Singh J, Marson AG. 2014. Antidepressants for people with epilepsy and depression. Cochrane Database Syst Rev. 12:CD010682.
- Malagon-Valdez J. 2004. The new antiepileptic drugs: their indications and side effects. Rev Neurol. 39:570–575.
- Miczek KA, Fish EW, De Bold JF, De Almeida RM. 2002. Social and neural determinants of aggressive behavior: pharmacotherapeutic targets at serotonin, dopamine and gamma-aminobutyric acid systems. Psychopharmacology (Berl). 163:434–458.
- Moshe SL, Perucca E, Ryvlin P, Tomson T. 2015. Epilepsy: new advances. Lancet. 385:884–898.
- Murillo-Rodriguez E, Arias-Carrion O, Zavala-Garcia A, Sarro-Ramirez A, Huitron-Resendiz S, Arankowsky-Sandoval G. 2012. Basic sleep mechanisms: an integrative review. Cent Nerv Syst Agents Med Chem. 12:38–54.
- Newton CR, Garcia HH. 2012. Epilepsy in poor regions of the world. Lancet. 380:1193–1201.
- Noam Y, Raol YH, Holmes GL. 2013. Searching for new targets for treatment of pediatric epilepsy. Epilepsy Behav. 26:253–260.
- Perez RM, Perez JA, Garcia LM, Sossa H. 1998. Neuropharmacological activity of Solanum nigrum fruit. J Ethnopharmacol. 62:43–48.
- Rios JL. 2011. Chemical constituents and pharmacological properties of Poria cocos. Planta Med. 77:681–691.
- Ruthrich H, Grecksch G, Krug M. 2001. Development of long-lasting potentiation effects in the dentate gyrus during pentylenetetrazol kindling. Int J Dev Neurosci. 19:247–254.
- Schousboe A, Madsen KK, White HS. 2011. GABA transport inhibitors and seizure protection: the past and future. Future Med Chem. 3:183–187.
- Song PP, Gao JJ, Kokudo N, Tang W. 2011. Standardization of traditional Chinese medicine and evaluation of evidence from its clinical practice. Drug Discov Ther. 5:261–265.
- Spelman K, Burns J, Nichols D, Winters N, Ottersberg S, Tenborg M. 2006. Modulation of cytokine expression by traditional medicines: a review of herbal immunomodulators. Altern Med Rev. 11:128–150.
- Tai T, Akahori A, Shingu T. 1993. Triterpenses of Poria cocos. Phytochemistry. 32:1239–1244.
- Takechi K, Suemaru K, Kawasaki H, Araki H. 2012. Impaired memory following repeated pentylenetetrazol treatments in kindled mice. Yakugaku Zasshi. 132:179–182.
- Temkin NR. 2009. Preventing and treating posttraumatic seizures: the human experience. Epilepsia. 50:10–13.
- Ueki T, Mizoguchi K, Yamaguchi T, Nishi A, Sekiguchi K, Ikarashi Y, Kase Y. 2015. Yokukansan, a traditional Japanese medicine, decreases head-twitch behaviors and serotonin 2A receptors in the prefrontal cortex of isolation-stressed mice. J Ethnopharmacol. 166:23–30.
- Wambebe C. 1985. Influence of some agents that affect 5-hydroxytryptamine metabolism and receptors on nitrazepam-induced sleep in mice. Br J Pharmacol. 84:185–191.
- Wang W, Dong H, Yan R, Li H, Li P, Chen P, Yang B, Wang Z. 2015. Comparative study of lanostane-type triterpene acids in different parts of Poria cocos (Schw.) Wolf by UHPLC-Fourier transform MS and UHPLC-triple quadruple MS. J Pharm Biomed Anal. 102:203–214.
- Xiang J, Jiang Y. 2013. Antiepileptic potential of matrine via regulation the levels of gamma-aminobutyric acid and glutamic acid in the brain. Int J Mol Sci. 14:23751–23761.
- Yang T, Kong B, Gu JW, Kuang YQ, Cheng L, Yang WT, Cheng JM, Ma Y, Yang XK. 2013. Anticonvulsant and sedative effects of paederosidic acid isolated from Paederia scandens (Lour.) Merrill. in mice and rats. Pharmacol Biochem Behav. 111:97–101.
- Zhao YY, Feng YL, Bai X, Tan XJ, Lin RC, Mei Q. 2013a. Ultra performance liquid chromatography-based metabonomic study of therapeutic effect of the surface layer of Poria cocos on adenine-induced chronic kidney disease provides new insight into anti-fibrosis mechanism. PLoS One. 8:e59617.
- ZhaoYY, FengYL, DuX, Xi ZH, Cheng XL, Wei F. 2012. Diuretic activity of the ethanol and aqueous extracts of the surface layer of Poria cocos in rat. J Ethnopharmacol. 144:775–778.
- Zhao YY, Lei P, Chen DQ, Feng YL, Bai X. 2013b. Renal metabolic profiling of early renal injury and renoprotective effects of Poria cocos epidermis using UPLC Q-TOF/HSMS/MSE. J Pharm Biomed Anal. 202–209:81–82.
- Zhao YY, Li HT, Feng YL, Bai X, Lin RC. 2013c. Urinary metabonomic study of the surface layer of Poria cocos as an effective treatment for chronic renal injury in rats. J Ethnopharmacol. 148:403–410.