Abstract
Context: The quantification of total collagen is of major importance in a wide range of research areas, including the study of cutaneous wound healing and new drugs trials.
Objective: The total collagen content in skin biopsies was compared by biochemical hydroxyproline assay and by two computer-aided histomorphometric analyses of histological sections.
Materials and methods: Two methods were used to evaluate collagen formation: the hydroxyproline assay, as the gold standard and histomorphometric image analysis of the filled areas by corresponding stained collagen fibres, using picrosirius and Gomori’s trichrome staining. The image analyses were determined by digital densitometry recognition using computer-aided ImageJ software. One-way ANOVA, simple linear regression and ANCOVA were applied for the statistical analysis and correlation.
Results: In a simple linear regression analysis carried out on the 14th day period after the induction of skin injury, three techniques, picrosirius red (F = 33.57, p = 0.00), Gomori’s trichrome (F = 81.61, p = 0.00) and hydroxyproline content (F = 16.85, p = 0.00) were able to detect collagen production. After scale adjustment, there were no significant differences among either the slopes (F = 1.17, p = 0.32) or the intercepts (F = 0.69, p = 0.51) of the estimated regression lines. It seems that a highly significant correlation exists between the histomorphometrical analysis and hydroxyproline assay.
Discussion and conclusion: The morphometric analysis proved to be adequate and can be used as a simple, rapid, low-cost technology for evaluating total collagen in cutaneous wound specimens, compared with the gold standard hydroxyproline assay.
Introduction
Wound healing is a dynamic process aimed at restoring the structure of the injured tissue (Menke et al. Citation2008; Ranzato et al. Citation2011). It can be divided into four overlapping phases: inflammation, coagulation, tissue formation and tissue remodelling (Menke et al. Citation2008; Stroncek et al. Citation2009). The process begins immediately after injury, with the release of platelet granules, cytokines and growth factors, which are important in recruiting inflammatory cells to the wound, an essential cellular influx for local debridement (inflammatory phase) to degrade foreign particles and to provide a provisional matrix to further proliferation of fibroblasts leading to formation of granulation tissue (Gurtner et al. Citation2008; Velnar et al. Citation2009).
The phase of new tissue formation is characterized by the formation of granulation tissue. Intense fibroblast proliferation and migration occur in order to synthesize the new extracellular matrix in the damaged tissue area (Stroncek et al. Citation2009; Velnar et al. Citation2009; Guo & Dipietro Citation2010). Once in the damaged tissue area, fibroblasts synthesize new matrix elements such as proteoglycans, glycosaminoglycans and collagen, the main components of the extracellular matrix, which are deposited in the damaged area to replace the initial provisional matrix, comprised initially of fibrin (Campos et al. Citation2008; Velnar et al. Citation2009; Guo & Dipietro Citation2010). Collagen is the major structural component of granulation tissue, strengthening the extracellular matrix. The amino-acid proline is an important component of the collagen fibre, and hydroxyproline is a biochemical marker for collagen tissue, as a positive indication of the progression of healing (Kokane et al. Citation2009; Nayak et al. Citation2011).
Studies on new therapeutic healing substances have gained importance in recent years because of the long treatment time and high costs of medical care associated with extensive or long-term wound therapy (Mulder et al. Citation2012; Moura et al. Citation2013). Some substances can stimulate fibroblast migration and/or proliferation, resulting in accelerated accumulation of extracellular matrix produced by these cells during the wound healing process. These events are important for tissue formation and the subsequent remodelling phase (Mulder et al. Citation2012).
Although there are studies in the literature on the quantification of the new collagen formation during the wound healing process by biochemical and/or morphometrical methods, reports comparing the two methods are rare (Kliment et al. Citation2011; Minossi et al. Citation2014). Therefore, the aim of this study was to compare, quantitatively and objectively, the total area occupied by collagen fibres in cutaneous wound specimens in Wistar rats, using the biochemical hydroxyproline assay and computer-aided histomorphometric analysis.
Materials and methods
Animals
Protocols involving the use of animals were performed according to the ethical guidelines of the Brazilian College of Animal Experimentation (COBEA), and approved by the Ethics Committee on Animal Experimentation (CETEA), of the Ribeirão Preto School of Medicine, University of São Paulo (FMRP-USP), registry number 085/2011. Forty adult male Wistar rats (180–200 g), aged 7–8 weeks were obtained from the central Bioterium of the Medical School, Ribeirão Preto, University of São Paulo (FMRPUSP). In order to prevent skin lesions from fighting males, which could interfere with wound repair, the animals were housed individually, at least one week before, and throughout the entire experimental period. The animals were maintained under standard laboratory conditions with a 12 h light–dark cycle and free access to food and water.
Surgical procedure and groups
Immediately before excisional wound induction, the animals were weighed and deeply anesthetized by intraperitoneal (i.p.) administration of a combination of ketamine (80 mg/kg) and xylazine (15 mg/kg). The animals backs were shaved and swabbed with 70% ethanol for asepsis; two circular full-thickness excision wounds were made on the dorsum cervical region with a sterile histological punch (1.5 cm diameter) comprising all the skin layers. Samples of the excised skin were saved for subsequent histological and biochemical assays, representing samples of basal collagen content in the rat skin (day 0).
The postoperative periods were defined according to the day of euthanization, as per standard protocols (n = 10 rats each postoperative days) (Fronza et al. Citation2014; Caetano et al. Citation2015). Both wounds of each animal were treated with 0.9% saline solution immediately after wound induction and, at the same time, each day thereafter. The wounds of all the animals were covered with gauze and tape, which were changed daily.
Harvesting of the material for study
The animals were euthanized on the 2nd, 7th, 14th and 21st postoperative days with an overdose of anaesthetic, and the wounds/scars were cut with a sterile biopsy punch (1.5 cm diameter). One wound from each animal was frozen in liquid nitrogen and stored at −80 °C for biochemical assay (hydroxyproline), and the other wound was used for the histological analysis.
Histological processing
The wound biopsies were fixed with buffered formaldehyde solution at 3.7% (pH 7.4) on days 0, 2, 7, 14 and 21 post-wounding, and processed according to the standard routine light microscope tissue protocols. The processed tissues were embedded in paraffin, (GT) and serial sections 5.0 μm thick were mounted on glass slides, dewaxed, rehydrated and stained separately with Gomori’s trichrome (GT) and picrosirius red (PR) for the analysis of collagenesis, with one glass slide to each staining on all post wounding days.
Histomorphometric analysis of collagenesis by image analysis
The slides of both staining in each day were examined and photomicrographed in a blinded fashion using a digital camera (LEICA DFC 280, Wetzlar, Germany) attached to a light microscope (LEICA DM 4000B, Wetzlar, Germany). Six images per slide were captured at 100× magnification (three fields from the epidermis-upper dermis and three fields from the lower dermis–hypodermis) for both histological staining. The colour deconvolution ImageJ software was used to evaluate the percentage of blue staining for GT and of red staining for PR, in the whole area of each image, with the dye indicating the presence of collagen fibres in the tissue. The morphometric analysis corresponding to the blue and red colours was measured as the percentage of total pixels in each image, using the Threshold (ImageJ software), as described by Caetano et al. (Citation2015). Data were reported as the pixel average of ten samples per day.
Hydroxyproline content (HyP)
Collagenesis was also measured using a biochemical hydroxyproline assay, according to the procedures described in the literature, but with minor modifications (Reddy & Enwemeka Citation1996; Reddy et al. Citation2008). Samples were incubated at 60 °C in open microtubes for 15 h to obtain the dry weight, cut using a Polytron tissue homogenizer with 6 N hydrochloric acid solution (100 μL per milligram of dry tissue) and incubated for 4 h at 130 °C to promote acid hydrolysis. Afterwards, the pH was adjusted to 7.0 at room temperature. Hydroxyproline standard solutions were prepared at concentrations of 1.0–100 μg/mL and 10 μL of standard and samples were transferred to a 96-well microplate. Then, 90 μL of 0.056 M chloramine-T solution and 100 μL of Ehrlich's reagent were added to each well. The absorbance values were measured at 550 nm and compared to those of the standard curve to determine the concentration of hydroxyproline in the samples. The concentrations of hydroxyproline in tissue homogenates were determined per volume of HCl used, and finally per milligram of dry tissue, based on a standard curve.
Statistical analysis
Data were expressed in scatter graphs and bar graphs expressed as mean ± standard error of mean (SEM) for the quantitative variables performed using GraphPad software (San Diego, CA). Statistical correlation between the histomorphometrical analysis of collagenesis by image analysis and hydroxyproline content were determined using one-way ANOVA, simple linear regression, and ANCOVA run in Systat 12.0 (Wilkinson Citation2007). For both methods, the null hypothesis was rejected when p ≤ 0.05.
Results
Collagenesis by quantitative image analysis
Collagen synthesis, deposition and degradation are important events that occur during the entire cutaneous wound-healing processes. Over the experimental period, we observed that the cutaneous wounds showed a marked and robust increase in the deposition and organization of collagen fibres, detected after staining with PR and GT in the wound biopsy ( and ). shows photomicrographs of each day of histological analyses for GT, in which the collagen is represented by blue-coloured tissue, and PR is represented by red-coloured tissue.
Figure 1. Collagen accumulation in wound areas at day 0, 2, 7, 14 and 21 after wounding. Representative photomicrograph of wounds tissue sections stained with Gomori’s trichrome (GT) and picrosirius red (PR) staining (100×). Note the collagen intensity and disposition of fibres stained blue and red, respectively.
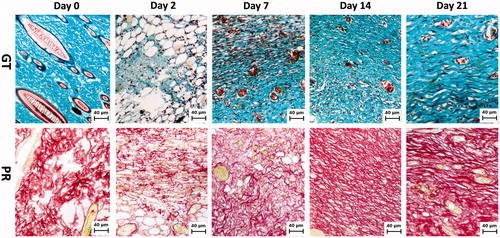
Figure 2. Quantification of collagen accumulation in the wound specimen on days 0, 2, 7, 14 and 21 post-wounding. Collagen content was measured by digital densitometry using ImageJ in sections of wound tissue stained with picrosirius red staining (open bars) and Gomori’s trichrome (dashed bars). The results show the collagen content in each specimen as a percentage of total area. The data represent the means ± SEM (n = 10 wounds/group). *The statistical evidence (p < 0.05) was analyzed by one-way-ANOVA.
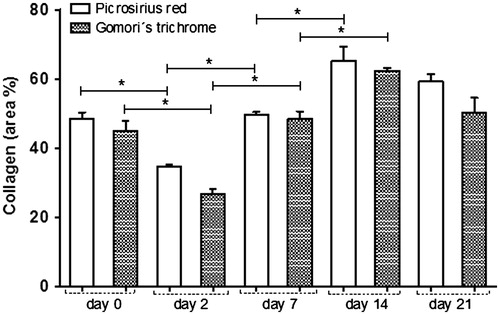
Histomorphometric analysis of collagenesis by image analysis from the skin revealed an expected decrease in the collagen fibres in the wounds on day 2, compared with day 0 (). The density of collagen fibres on day 7 post-wounding were higher than on day 2, and similar to the physiological levels observed on day 0, increasing still further after 14 d. Interestingly, on day 21, the concentration of collagen fibre slightly decreased, as a result of tissue remodelling, and seemed better organized and distributed compared to previous days ( and ).
Measurement of hydroxyproline content in the wounds
The results of the hydroxyproline concentration in the skin display similar pattern to those results observed in the histomorphometrical analysis. At day 2 post-wounding, the hydroxyproline content in the skin was significantly lower (p < 0.05) than on day 0. After 7 and 14 d, a continuous increase in hydroxyproline was observed. Following the same fact as observed in the histological slides, on day 21, the collagen content declined slightly, to levels similar to those observed on day 0 ().
Figure 3. Determination of collagen accumulation in wound biopsies at day 0, 2, 7, 14 and 21 after injury. Determination of wound hydroxyproline content as an indicator of collagen levels in μg of hydroxyproline/mg of dry wound tissue. The data represent means ± SEM (n = 10 wounds/group). *Statistical evidence was (p < 0.05) analyzed by one-way ANOVA.
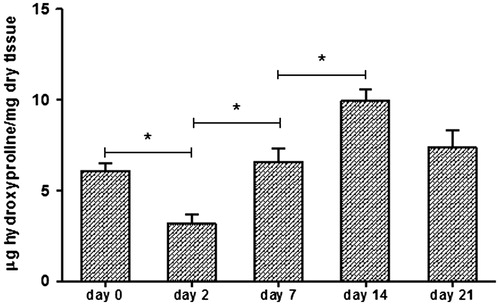
Correlations between methods
Percentage data obtained from the image measures were transformed into the arcsine of the square root of their respective proportions. In order to adjust the scale for data comparisons, all measurements were standardized through ranging (Zar Citation2010). The assumption of normality for colour measurements in both the staining and the biochemical techniques were verified by Lillefors test, and the null hypothesis of normality was rejected for p ≤ 0.05 (Zar Citation2010). After transformation and standardization of the colour measurements, they were distributed normally for PR (p = 0.27) and GT staining (p = 0.14). Differences in capability of collagen detection among the three techniques were tested through two-way analysis of variance (ANOVA), and the predictability of collagen production over the period 2–14 d was verified through simple linear regression taking collagen production as a function of time in days of observation (Zar Citation2010). Differences among the slopes and the intercepts of the estimated regression lines were tested through analysis of covariance – ANCOVA. Two-way ANOVA, simple linear regression and ANCOVA were run in Systat 12.0 (Wilkinson Citation2007), and for both of them, the null hypothesis was rejected when p ≤ 0.05).
In relation to collagen detection power, there were no significant differences among the measurements obtained through the three techniques in each treatment, from normal skin (day 0) or injured skin, over a 21-d period of observation (FANOVA = 0.02, df = 2;70, p = 0.99, r = 0.80, r2 = 0.63). Even when the techniques were contrasted without considered the healing phases, no significant difference could be found among them (FANOVA = 0.03, df = 2;72, p = 0.92, r = 0.80, r2 = 0.63). The only significant differences found were due to the wound healing phase after injury (FANOVA = 124.14, df = 1;74, p = 0.00). However, the estimated linear model had a non-expressive capability to explain the natural variance in the original data (r = 0.16, r2 = 0.02), since the normal skin (day 0) and the skin 21 d after injury were outside the linear trend in collagen production ().
Figure 4. Correlation between methods. Collagen production measured by the Hydroxyproline production assay (―•―), Picrocirius red (-▴-) and Gomori’s trichrome (- -•- -).
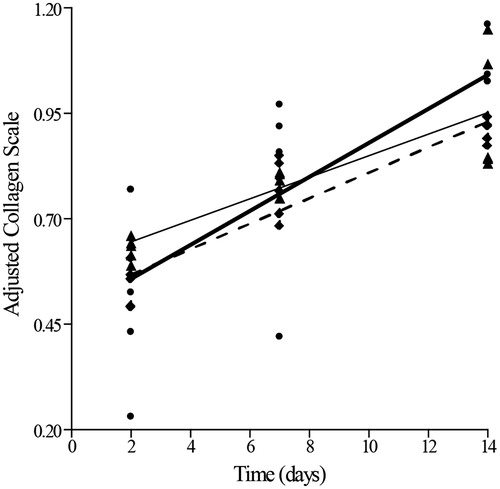
In the simple linear regression analysis during 14 d after skin injury (2, 7 and 14 d post-wounding), the three techniques, PR (F = 33.57, p = 0.00), GT (F = 81.61, p = 0.00) and hydroxyproline content (F = 16.85, p = 0.00), were able to detect a growing collagen production (). After scale adjustment for comparisons, there were no significant differences among either the slopes (F = 1.17, p = 0.32), or the intercepts (F = 0.69, p = 0.51) of the estimated regression lines. Therefore, the three techniques were equally sensitive in detecting collagen synthesis (and ).
Table 1. Statistical parameters of the estimated regression lines for the three techniques used to assess collagen production in the regeneration process.
Discussion
In an animal model of cutaneous wound healing, it is important to measure new collagen formation and its quality. Collagen is the major component of the connective tissue. The wound-healing processes depend on the right metabolism of collagen, which comprises the regulatory production, deposition and subsequent maturation of collagen. These events are of vital interest not only in cutaneous wound healing but also in a number of clinically important diseases that are characterized by an accumulation or loss of tissue collagen. On one hand, excessive production of collagen has been documented in many disorders, such as liver cirrhosis, lung fibrosis, and hypertrophic and keloid scars. On the other hand, loss of tissue collagen has been observed in certain disorders of the connective tissue, including rheumatoid arthritis and wound/ulcer damaged tissues (Milner & Cawston Citation2005; Wynn Citation2008; Shih et al. Citation2010).
In some clinical situations, such as tissue repair and wound healing, the production and deposition of collagen during the initial stages of healing are required to heal the damaged tissues (Frade et al. Citation2001, Citation2004). Hence, collagen plays a vital role in maintaining structural integrity and in determining tissue function. Considering the important role of collagen in healing, there is a demand for techniques for its evaluation and quantification (Yung-Kai & Che-Yung Citation2010).
The analysis of hydroxyproline in biological tissues is considered the gold standard method for routine measurement of collagen content in extracts of various tissue specimens (Reddy & Enwemeka Citation1996). Despite the numerous assay procedures described for hydroxyproline analysis, this assay has its limitations in regard to specificity, sensitivity, reproducibility, accuracy and practical approach. In general, the procedures are laborious and involve many time-consuming steps (Dang et al. Citation2005; Ignat’eva et al. Citation2007; Kliment et al. Citation2011).
In this study, the correlations between hydroxyproline concentrations and collagen content measured by digital densitometry in wound tissue sections stained with PR and GT were equally sensitive. In relation to collagen detection power, there were no significant differences among the measurements obtained through the three techniques in each blocked treatment, from normal skin (day 0) or injured skin, over a 21-d long period of observation. Even comparing the techniques without considering the healing phases, no significant differences were seen among them.
Histomorphometric analysis by histological sections has been proven to be a simple, rapid assay that uses low-cost technology and materials. However, there is a very important standardization step during the quantification that researchers need to bear in mind. In this study, the authors used the ImageJ software to work on all the images and quantifications for both stained slides. The colour deconvolution plugin from ImageJ software was used to evaluate the blue and red staining percentage (collagen) in the image area from GT and PR, respectively. The plugin recognizes the image colours and decomposes them into another three basic images. The blue image for GT and the red image for PR should be used for the quantification. The morphometric analyses, corresponding to the blue or red colours, were measured as the percentage of total pixels in each image using the ‘Threshold plugin’, also from ImageJ software, as described previously by Caetano et al. (Citation2015). Two bars to adjust the colour intensity in each image are created by the Threshold plugin. This is the crucial step for obtaining the real result, because both bars need to be standardized prior to start the quantification, otherwise researchers could obtain unrealistic data.
Perini et al. (Citation2015) also used histomorphometric analysis and the biochemical assay to determine collagen deposition in samples. The authors demonstrated the similarity of the two methods. Pessoa et al. (Citation2015) also used GT and PR staining to evaluate new collagen in rat skin samples. The authors reported good efficacy of the method to achieve realistic and satisfactory data.
Conclusion
In conclusion, a simple, convenient and reliable computer-aided histomorphometric method for the determining total collagen in cutaneous wound specimens has been proven to be comparable to the gold standard hydroxyproline assay. The methods proved to be equivalent, therefore, the choice of the methodology will depend on the laboratory conditions and infrastructure. It is important to note that both methods are considered useful tools in clinical and biomedical research.
Funding information
The authors gratefully acknowledge FAPESP, CAPES, FAEPA-HCFMRP-USP, FAPES and CNPq for their financial support.
Disclosure statement
The authors report that they have no conflicts of interest.
References
- Caetano GF, Frade MAC, Andrade TAM, Leite MN, Bueno CZ, Moraes AM, Ribeiro-Paes JT. 2015. Chitosan–alginate membranes accelerate wound healing. J Biomed Mater Res Part B: Appl Biomater. 103:1013–1022.
- Campos AC, Groth AK, Branco AB. 2008. Assessment and nutritional aspects of wound healing. Curr Opin Clin Nutr Metab Care. 11:281–288.
- Dang YY, Ren QS, Liu HX, Ma JB, Zhang JS. 2005. Comparison of histologic, biochemical, and mechanical properties of murine skin treated with the 1064-nm and 1320-nm Nd: YAG lasers. Exp Dermatol. 14:876–882.
- Frade MA, Valverde RV, de Assis RV, Coutinho-Netto J, Foss NT. 2001. Chronic phlebopathic cutaneous ulcer: a therapeutic proposal. Int J Dermatol. 40:238–240.
- Frade MAC, Cursi IB, Andrade FF, Coutinho-Netto J, Barbetta FM, Foss NT. 2004. Management of diabetic skin wounds with natural latex biomembrane. Med Cutan Iber Lat Am. 32:157–162.
- Fronza M, Caetano GF, Leite MN, Bitencourt CS, Paula-Silva FWG, Andrade TAM, Frade MAC, Melfort I, Faccioli LH. 2014. Hyaluronidase modulates inflammatory response and accelerates the cutaneous wound healing. PLoS One. 9:e112297.
- Guo S, Dipietro LA. 2010. Factors affecting wound healing. J Dent Res. 89:219–229.
- Gurtner GC, Werner S, Barrandon Y, Longaker MT. 2008. Wound repair and regeneration. Nature. 453:314–321.
- Ignat’eva NY, Danilov NA, Averkiev SV, Obrezkova MV, Lunin VV, Sobol EN. 2007. Determination of hydroxyproline in tissues and the evaluation of the collagen content of the tissues. J Anal Chem. 62:51–57.
- Kliment CR, Englert JM, Crum LP, Oury TD. 2011. A novel method for accurate collagen and biochemical assessment of pulmonary tissue utilizing one animal. Int J Clin Exp Pathol. 4:349–355.
- Kokane DD, More RY, Kale MB, Nehete MN, Mehendale PC, Gadgoli CH. 2009. Evaluation of wound healing activity of root of Mimosa pudica. J Ethnopharmacol. 124:311–315.
- Menke MN, Menke NB, Boardman CH, Diegelmann RF. 2008. Biologic therapeutics and molecular profiling to optimize wound healing. Gynecol Oncol. 111:S87–S91.
- Milner JM, Cawston TE. 2005. Matrix metalloproteinase knockout studies and the potential use of matrix metalloproteinase inhibitors in the rheumatic diseases. Curr Drug Targets Inflamm Allergy. 4:363–375.
- Minossi JG, Lima Fde O, Caramori CA, Hasimoto CN, Ortolan EV, Rodrigues PA, Spadella CT. 2014. Alloxan diabetes alters the tensile strength, morphological and morphometric parameters of abdominal wall healing in rats. Acta Cir Bras. 29:118–124.
- Moura LIF, Dias AMA, Carvalho E, de Sousa HC. 2013. Recent advances on the development of wound dressings for diabetic foot ulcer treatment – a review. Acta Biomater. 9:7093–7114.
- Mulder G, Wallin K, Tenenhaus M. 2012. Regenerative materials that facilitate wound healing. Clin Plast Surg. 39:249–267.
- Nayak BS, Kanhai J, Milne DM, Pinto-Pereira L, Swanston WH. 2011. Experimental evaluation of ethanolic extract of Carapa guianensis L. leaf for its wound healing activity using three wound models. Evid Based Complement Alternat Med. 1–6, 2011. Article ID 419612.
- Perini JA, Angeli-Gamba T, Alessandra-Perini J, Ferreira LC, Nasciutti LE, Machado DE. 2015. Topical application of Acheflan on rat skin injury accelerates wound healing: a histopathological, immunohistochemical and biochemical study. BMC Complement Altern Med. 15:203–210.
- Pessoa WS, Estevão LR, Simões RS, Mendonça Fde S, Evência-Luz L, Baratella-Evência L, Florencio-Silva R, Sá FB, Evêncio-Neto J. 2015. Fibrogenesis and epithelial coating of skin wounds in rats treated with angico extract (Anadenanthera colubrina var. cebil). Acta Cir Bras. 30:353–358.
- Ranzato E, Martinotti S, Burlando B. 2011. Wound healing properties of jojoba liquid wax: an in vitro study. J Ethnopharmacol. 134:443–449.
- Reddy AS, Kalyani S, Kumar NS, Boddu VM, Krishnaiah A. 2008. Dehydration of 1,4-dioxane by pervaporation using crosslinked calcium alginate–chitosan blend membranes. Polym Bull. 61:779–790.
- Reddy GK, Enwemeka CS. 1996. A simplified method for the analysis of hydroxyproline in biological tissues. Clin Biochem. 29:225–229.
- Shih B, Garside E, McGrouther DA, Bayat A.2010. Molecular dissection of abnormal wound healing processes resulting in keloid disease. Wound Repair Regen. 18:139–153.
- Stroncek JD, Bell N, Reichert WM. 2009. Instructional Powerpoint presentations for cutaneous wound healing and tissue response to sutures. J Biomed Mater Res. 90A:1230–1238.
- Velnar T, Bailey T, Smrkolj V. 2009. The wound healing process: an overview of the cellular and molecular mechanisms. J Int Med Res. 37:1528–1542.
- Wilkinson L. 2007. The system for statistics. Version 12.0. San José (CA): Software Inc.
- Wynn TA. 2008. Cellular and molecular mechanisms of fibrosis. J Pathol. 214:199–210.
- Yung-Kai L, Che-Yung K. 2010. Development of 4-hydroxyproline analysis kit and its application to collagen quantification. Food Chem Toxicol. 119:1271–1277.
- Zar JH. 2010. Biostatistical analysis. Englewood Cliffs (NJ): Prentice-Hall.