Abstract
Context and objective: Viperid venom-induced chronic local-toxicity continues even after anti-snake venom treatment. Therefore, traditional antidote Albizia lebbeck L. (Fabaceae) seed extract was tested against Echis carinatus S. (Viperidae) venom (ECV)-induced local toxicity to evaluate its complementary remedy.
Materials and methods: Soxhlet extraction of A. lebbeck seeds was performed with the increasing polarity of solvents (n-hexane to water); the extract was screened for phytochemicals (alkaloids, anthraquinones, flavonoids, glycosides, phenolics, saponins, steroids and tannins). In preliminary in vitro analysis, A. lebbeck methanolic extract (ALME) demonstrated significant inhibition of ECV proteases, the major enzyme–toxin responsible for local- toxicity. Therefore, in vitro neutralizing potential of ALME was further evaluated against hyaluronidases and phospholipase A2 (1:1–1:100 w/w). In addition, alleviation of ECV induced characteristic local- toxicity [haemorrhage (i.d.) and myotoxicity (i.m.)] was determined in mice.
Results: ALME contained high concentrations of phenolics and flavonoids and demonstrated significant in vitro inhibition of ECV protease (IC50 = 36.32 μg, p < 0.0001) and hyaluronidase (IC50 = 91.95 μg, p < 0.0001) at 1:100 w/w. ALME significantly neutralized ECV induced haemorrhage (ED50 = 26.37 μg, p < 0.0001) and myotoxicity by significantly reducing serum creatinine kinase (ED50 = 37.5 μg, p < 0.0001) and lactate dehydrogenase (ED50 = 31.44 μg, p = 0.0021) levels at 1:50 w/w.
Discussion and conclusion: ALME demonstrated significant neutralization of ECV enzymes that contribute in local tissue damage and haemostatic alterations. The study scientifically supports the anecdotal use of A. lebbeck in complementary medicine and identifies ALME as principle fraction responsible for antivenom properties.
Introduction
Advent of medicine has increased the life expectancy and health status of mankind (Jha et al. Citation2006). However, certain pathologies due to severe bites from venomous species are still hard to manage. Among venomous species, snakes are responsible for the majority of casualties (White Citation2005). Venomous snakes possess unique, sophisticated offensive system in the form of venom delivery apparatus, which introduces venom into the prey/victim by bite (Suntravat et al. Citation2010). The symptoms of snakebite range from mere local swelling to widespread loss of tissue integrity and, in most instances, death (Nanjaraj Urs et al. Citation2013). Among Indian snakes, Echis carinatus S. (Viperidae) envenomation is associated with severe progressive local tissue damage which arises due to the combined action of three hydrolytic enzymes (proteases, hyaluronidases and phospholipase A2s) (Nanjaraj Urs et al. Citation2013). Inhibition of the enzymes destructive action remains a challenge to the medical fraternity, since the local tissue damage continues to occur even after the administration of anti-snake venom (ASV) as it offers less protection against local tissue damage and also causes hypersensitivity issues (Juckett & Zhancox Citation2002; Gomes et al. Citation2010; Nanjaraj Urs et al. Citation2013). To overcome these limitations, plant-based medicines are being extensively used as an alternative/complementary therapy for snakebite (Gomes et al. Citation2010; Nanjaraj Urs et al. Citation2013). Hence, plant preparations and isolated compounds have gained importance in venom research as they can act individually or in combination to reduce the spread of toxin, dissociate the toxin complexes and reduce venom potency, provide optimal conditions to aid physiological response against venom toxicity, or neutralize the venom toxicity as a whole (Gomes et al. Citation2010).
Albizia lebbeck L. (Fabaceae) is a folk medicinal plant commonly called woman’s tongue tree (Siris in Hindi), used in Indian traditional medicine for the treatment of snakebite, scorpion sting, haemorrhage, gum inflammation, and related clinical conditions. All parts of the A. lebbeck plant are recommended in folk medicine for the treatment of snakebite (Kiritikar & Basu Citation1935; Tripathi et al. Citation1979; Kapoor Citation2000; Anonymous Citation2001; Khare Citation2008; Asad et al. Citation2011; Minu et al. Citation2012; Asad et al. Citation2014). Based on the available reports (Kiritikar & Basu Citation1935; Tripathi et al. Citation1979; Kapoor Citation2000; Anonymous Citation2001; Khare Citation2008; Asad et al. Citation2011; Minu et al. Citation2012; Asad et al. Citation2014) and personal conversations with local folk healers of Mysuru and Hassan (Karnataka, India), A. lebbeck seed extract was selected for the study. Systematic evaluation of its anecdotal potentials against the E. carinatus venom was performed both in vitro and in vivo.
Materials and methods
Chemicals
Alcian blue, Escherichia coli [lyophilized cells of strain W (ATCC9637)], fatty acids, fibrinogen, gelatin, N-acetyl glucosamine (NAG) and p-DMAB were purchased from Sigma Chemicals (St. Louis, MO). 14C-Oleic acid was obtained from Perkin Elmer Life Sciences Inc. (Boston, MA). Scintillation cocktail (Ultima Gold) was obtained from Packard Bioscience Co. (Meriden, CT). Hyaluronic acid was purchased from Across Organics (Morris, NJ). Thrombin was procured from Diagnostica Stago (Paris, France). Bovine serum albumin (BSA) and casein were obtained from Sisco Research Laboratories Pvt. Ltd. (Mumbai, India). All other chemicals and reagents used in this study were of analytical grade. Solvents were redistilled before use.
Snake venom
Lyophilized powder of E. carinatus venom (ECV) was procured from Irula Co-operative Society Ltd. (Chennai, India). The required amount of ECV was reconstituted with saline and centrifuged at 6000 g for 10 min to remove debris. The supernatant was stored as aliquots at −20 °C until further use. Protein content of crude venom was determined according to the published method (Lowry et al. Citation1951) and studies were carried out based on the protein content of ECV.
Animals
Swiss albino mice (20–30 g; 6–8 weeks; either gender) were obtained from Central Animal House Facility, University of Mysore (UOM), Mysuru (Karnataka, India). Animals were maintained in polypropylene cages with 12 h light/dark cycle. Animal care and handling were conducted in compliance with the National Regulations for Animal Research and the experiments were carried out according to the protocols reviewed by the Institutional Animal Ethical Committee (IAEC), UOM, Mysuru (sanction order no. UOM/IAEC/25/2011).
Human plasma
Blood was drawn from healthy human volunteers (20–30 years) with their consent, mixed with trisodium citrate (9:1) and centrifuged at 250 g for 15 min. Supernatant was used as platelet poor plasma (PPP) for coagulation assays. The experiments were conducted according to the protocols reviewed by the Institutional Human Ethical Committee (IHEC), UOM, Mysuru (sanction order no. IHEC-UOM No. 62/Ph.D/2011-12).
Plant material
Seeds of A. lebbeck were collected in Manasagangothri campus, UOM, Mysuru, during the month of February, 2013. The plant was identified and authenticated by Dr. K. A. Sharvani, Assistant Professor, Department of Botany, Yuvaraja's College, UOM, Mysuru (voucher specimen accession no. 0256).
Soxhlet extraction
The pulverized seeds of A. lebbeck (15 g) were used for preparation of hot extracts by the Soxhlet method using different solvents (1:25 w/v) with increasing polarity (n-hexane, chloroform, ethyl acetate, methanol and water). The extracts were filtered and dried using a rotary flash evaporator and further redissolved in dimethyl sulphoxide and serially diluted in PBS. Extract yield was calculated and expressed in percentage (dry weight of solvent extracts/dry weight of crude extract) (Nanjaraj Urs et al. Citation2015a).
Phytochemical screening
The extracts were screened quantitatively for different phytochemicals such as alkaloids, anthraquinones, flavonoids, glycosides, phenolics, saponins, steroids and tannins as described in our earlier publication (Nanjaraj Urs et al. Citation2015a).
Protease activity
Caseinolytic activity was determined according to the published method with slight modifications (Yariswamy et al. Citation2013). For inhibition studies, 25 μg of ECV was separately pre-incubated with various concentrations of A. lebbeck seed extracts (1:1–1:100 w/w) at 37 °C for 15 min prior to assay with appropriate controls. One unit of enzyme activity was defined as the amount of enzyme required to increase an absorbance of 0.01 at 660 nm/h at 37 °C.
Gelatinolytic activity of ECV by substrate gel assay was carried out according to the published protocol with slight modifications (Yariswamy et al. Citation2013). For inhibition studies, 10 μg of ECV was pre-incubated with A. lebbeck methanol extract (ALME) (1:100; w/w) at 37 °C for 15 min prior to assay with appropriate controls. The activity was visualized as a clear zone of hydrolysis against dark blue background. Zones of hydrolysis were measured using graph sheet and the results were expressed in mm2.
Plasma clot hydrolyzing activity
Human plasma clot hydrolyzing activity was performed according to our established method with slight modifications (Rajesh et al. Citation2007). The cleavage pattern of washed plasma clot by ECV was analyzed by 10% SDS-PAGE (Laemmli Citation1970). For inhibition studies, similar procedure was followed after pre-incubating 10 μg of ECV with various concentrations of ALME (1:10–1:100 w/w) for 15 min at 37 °C.
Haemorrhagic activity
Haemorrhagic activity was assayed according to our previously published method (Nanjaraj Urs et al. Citation2015b). Inhibition studies were carried out by pre-incubating (for 15 min), co-injecting (simultaneous) and independently injecting (5 min post ECV injection) various concentrations of ALME (1:10–1:50 w/w). Saline, 3 μg ECV and ALME alone injected (i.d.), respectively, served as negative, positive and extract controls. Inhibition of haemorrhagic activity was observed as a decreased area of haemorrhagic spot in comparison with ECV-injected haemorrhagic spot. Haemorrhagic spot was measured using graph sheet and the results were expressed in mm2.
Myotoxicity
Myotoxic activity was measured according to our previously published method (Nanjaraj Urs et al. Citation2015b). Inhibition studies were carried out by co-injecting various concentrations of ALME (1:10–1:50 w/w) to thigh muscle. Saline, 5 μg ECV and extracts alone injected (i.m.) served as negative, positive and extract controls, respectively.
Re-calcification time
Plasma re-calcification time was determined according to the published method with slight modifications (Rajesh et al. Citation2007). For inhibition studies, 1 μg of ECV was pre-incubated with various concentrations of ALME (1:1–1:100 w/w) at 37 °C for 15 min prior to assay with appropriate controls. Further, the specificity of ALME towards ECV metalloproteases was confirmed by thrombin time.
Fibrinogenolytic activity
Fibrinogenolytic activity was performed according to the published method with slight modifications (Rajesh et al. Citation2007). For inhibition studies, similar experiment was carried out by pre-incubating 2 μg of ECV with various concentrations of ALME (1:10–1:100 w/w) for 15 min at 37 °C. The degradation pattern of fibrinogen subunits was observed following electrophoresis on 10% SDS-PAGE under reducing conditions and staining with Coomassie brilliant blue (Laemmli Citation1970).
Hyaluronidase activity
Hyaluronidase activity was carried out according to the published method with slight modifications (Tarannum et al. Citation2012). For inhibition studies, hyaluronidase activity was determined after pre-incubating 100 μg of ECV with various concentrations of ALME (1:10–1:100 w/w) for 15 min at 37 °C with appropriate controls. One unit of enzyme activity was defined as the amount of enzyme required to increase an absorbance of 0.01 at 585 nm/h at 37 °C.
Further, hyaluronidase activity by substrate gel assay was performed as described in our earlier publication with slight modifications (Tarannum et al. Citation2012). For inhibition studies, similar experiment was carried out with 100 μg of ECV, following pre-incubation with ALME (1:100; w/w) for 15 min at 37 °C with appropriate controls. The activity was visualized as a clear zone of hydrolysis against greenish blue background. Zones of hydrolysis were measured using graph sheet and the results were expressed in mm2.
Phospholipase A2 activity
PLA2 activity was measured using 14C-oleate-labelled autoclaved E. coli cells as a substrate according to our previously published method using liquid scintillation counter (Mohamed et al. Citation2011). For inhibition studies, 30 μg of ECV was pre-incubated with various concentrations of ALME (1:10–1:100 w/w) at 37 °C for 15 min prior to assay with appropriate controls. Inhibition was expressed in percentage compared to ECV activity.
Statistical analysis
The results of experiments were expressed as mean ± SD (n = 3). Statistical analysis was carried out using Student's t-test. The comparison between the groups was considered significant if p ≤ 0.05. Data were analyzed using the statistical package Graph Pad Prism® (La Jolla, CA).
Results and discussion
Present work is an attempt to scientifically evaluate the medicinal importance of A. lebbeck seeds in treating ECV induced local manifestations. The pulverized seeds of A. lebbeck were subjected to Soxhlet extraction and the extracts obtained were filtered, dried and stored for further use. The yields of different solvent extracts were expressed as percentage (dry weight of solvent extract/dry weight of crude extract); n-hexane (0.6% w/w), chloroform (0.6% w/w), ethyl acetate (1.7% w/w), methanol (3% w/w) and water (2.2% w/w). Phytochemical screening of A. lebbeck seed extracts showed the presence of alkaloids, anthraquinones, flavonoids, glycosides, phenolics, saponins and steroids (). Among different solvent extracts, methanol extract showed the presence of all classes of phytochemicals except tannins. The major classes of phytochemicals in ALME were found to be phenolics and flavonoids. All the extracts were tested for the inhibition of ECV enzymatic toxins (proteases, hyaluronidases and phospholipase A2s) in vitro and their pharmacological actions in vivo using murine model.
Table 1. Summary of phytochemical constituents of Albizia lebbeck seed extracts.
Initially, inhibitory potential of A. lebbeck extracts were screened towards the inhibition of proteases, major enzymatic toxin of ECV. Among the extracts, ALME significantly inhibited proteolytic (caseinolytic) activity of ECV. The inhibition was concentration dependent and significant inhibition was observed at venom to extract ratio of 1:100 w/w (97%; p < 0.0001; IC50 = 36.32 μg) ( and ). Based on the above findings, ALME was retained for further neutralization studies. The inhibitory potential of ALME towards ECV proteases was further evaluated on gelatin, fibrin and fibrinogen. ECV metalloproteases efficiently hydrolyzed gelatin and fibrin (α subunit) in a dose-dependent manner. ALME significantly inhibited gelatinolytic activity of ECV at venom to extract ratio of 1:100 w/w (50%; p < 0.0001) (). Further, ALME also prevented the fibrinolytic activity of ECV, which was evident by the existence of α subunit of fibrin compared to ECV alone ().
Figure 1. Dose-dependent inhibition of protease activity of ECV by ALME: reaction mixture (1 mL) contained 0.4 ml of casein (2% in 0.2 M Tris-HCl buffer, pH 8.5), incubated with 25 μg of ECV + different concentrations of ALME ranging from 1:1 to 1:100 w/w for 2.5 h at 37 °C. Data represents mean ± SD (n = 3). *p < 0.05, **p < 0.01 and ***p < 0.001 compared with ECV.
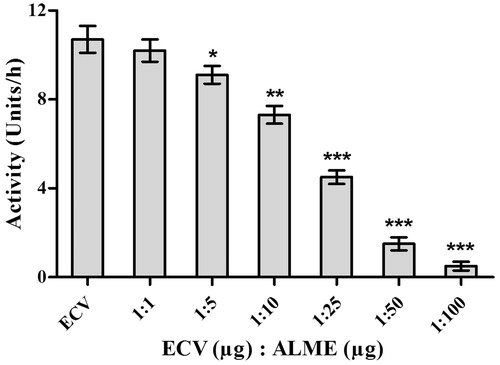
Figure 2. Inhibition of gelatinolytic activity of ECV by ALME: gelatin (0.08%) was incorporated into the 10% SDS polyacrylamide resolving gel matrix. ECV (10 μg) alone and ECV pre-incubated with ALME for 15 min was loaded onto separate wells. Lanes, (A) 10 μg ECV; (B) 1:100 [ECV (μg): ALME (μg)]. Data represent mean ± SD (n = 3). ***p < 0.001 compared with ECV.
![Figure 2. Inhibition of gelatinolytic activity of ECV by ALME: gelatin (0.08%) was incorporated into the 10% SDS polyacrylamide resolving gel matrix. ECV (10 μg) alone and ECV pre-incubated with ALME for 15 min was loaded onto separate wells. Lanes, (A) 10 μg ECV; (B) 1:100 [ECV (μg): ALME (μg)]. Data represent mean ± SD (n = 3). ***p < 0.001 compared with ECV.](/cms/asset/eceaaf9c-8eb8-4dda-b904-2fa8131d8616/iphb_a_1171882_f0002_b.jpg)
Figure 3. Dose-dependent inhibition of plasma clot hydrolyzing activity of ECV by ALME: washed plasma clot was incubated with 10 μg of ECV + varying concentrations of ALME in 10 mM Tris-HCl pH 7.6 for 3 h and cleavage pattern of the plasma clot was analyzed using 10% SDS-PAGE. (A) Plasma clot alone; (B) and (G) plasma clot +10 μg ECV; (C)–(F) 1:10, 1:25, 1:50, 1:100 ALME (w/w), respectively.
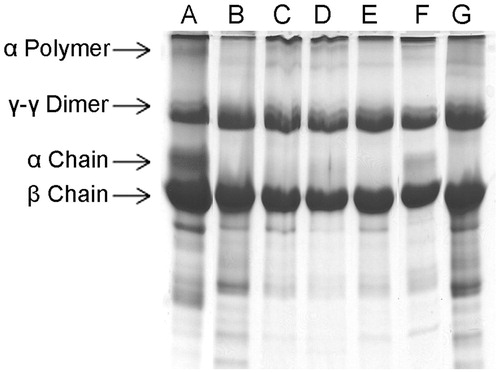
ECV is a rich source of proteases, which are responsible for haemostatic and local tissue manifestations due to their action on extracellular matrix (ECM) and haemostatic system (Nanjaraj Urs et al. Citation2015a). Majority of proteolytic activity of ECV is contributed by metalloproteases (about 90%) and the rest is due to serine proteases (about 10%) (Nanjaraj Urs et al. Citation2015b). Snake venom proteases acting on ECM and basement membrane proteins characterized to date are metalloproteases which cause haemorrhage and associated clinical complications. The onset of haemorrhage is due to destruction of basement membrane proteins and ECM surrounding the blood vessels, resulting in fragile blood vessels, which rupture easily. Apart from this, fibrinolytic activity of ECV results in clot dissolution contributing to haemorrhage. Haemorrhage in turn leads to hypoxia, a secondary event, which is the onset for progressive local tissue damage and necrosis (Hasson et al. Citation2012; Nanjaraj Urs et al. Citation2015b).
To support the inhibitory potential of ALME towards ECV mediated gelatinolytic and fibrinolytic activities, it was tested for ECV-induced haemorrhagic activity inhibition. Initially, pre-incubation experiments were conducted using different ratios of ECV and ALME (w/w). Complete inhibition of haemorrhage was observed at 1:50 w/w. Based on the results of pre-incubation and co-injection, independent injection studies (5 min post-ECV injection) were performed at 1:50 w/w. The result demonstrated significant prevention of haemorrhage upon independent injection at the tested dose (92%; p < 0.0001, ED50 = 26.37 μg) ( and ). Potent inhibition of ECV-induced haemorrhage by ALME suggests its role in preventing the onset of local tissue damage and further spreading. Metalloproteases of ECV induce myotoxicity, which is secondary to haemorrhage, characterized by elevated serum creatine kinase (CK) and lactate dehydrogenase (LDH) (Pierce et al. Citation2011). One of the isoforms of these enzymes is abundantly present in the cytosolic compartment of skeletal muscles and is released into the circulation following skeletal muscle damage as observed in many clinical conditions including snakebite (Arruda et al. Citation2002). ALME inhibited the myotoxicity induced by ECV significantly, evidenced by reduced serum CK (64%; p < 0.0001; ED50 = 37.5 μg) and LDH (64%; p = 0.0021; ED50 = 31.44 μg) levels at the ratio 1:50 w/w upon co-injection ( and ).
Figure 4. Haemorrhage inhibition studies of ECV using ALME: Mice were injected intradermal with constant 3 μg of ECV and various concentrations of ALME (5 min post ECV injection). After 3 h mice were sacrificed and haemorrhagic spot appeared on the inner surface was measured using graph sheet and the results were expressed in mm2. (A) 3 μg ECV; (B)–(D) 1:10, 1:25, 1:50 ALME (w/w), respectively; (E) saline; (F) ALME alone. Data represent mean ± SD (n = 3). ***p < 0.001 compared with ECV.
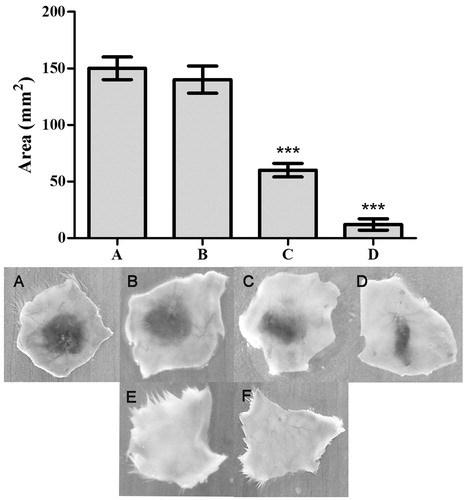
Figure 5. Myotoxicity inhibition studies of ECV using ALME: mice were co-injected with 5 μg of ECV + different concentrations of ALME (simultaneously). After 3 h mice were sacrificed and serum CK and LDH levels were assayed using AGAPPE kit. Data represent mean ± SD (n = 3). *p < 0.05, **p < 0.01 and ***p < 0.001 compared with ECV.
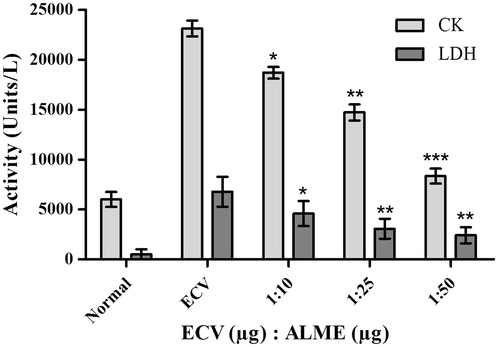
Apart from extensive tissue damage, ECV exerts its action towards various components of blood (Hiremath et al. Citation2013), mainly, the coagulation factors and exhibits a strong pro-coagulant effect in vitro [32 s versus 300 s (normal)]. The pro-coagulant effect of ECV was inhibited by ALME at the ratio of 1:100 w/w (83%; p < 0.0001) and the clotting time was restored to normal (250 s) (). The pro-coagulant effect of ECV is predominantly due to prothrombin activating metalloproteases – ecarin and carinactivase (Kornalik & Blomback Citation1975; Yamada et al. Citation1996). Inhibition of pro-coagulant activity of ECV by ALME was supported by the inhibition of fibrinogenolytic activity of ECV. ALME pre-treated ECV failed to hydrolyze Aα and Bβ subunits of fibrinogen, which are susceptible to the action of ECV, thereby resulting in an intact fibrinogen, which is responsible for restoration of clotting time (Hiremath et al. Citation2015). ALME showed complete inhibition of fibrinogenolytic activity of ECV at the ratio of 1:100 w/w (). The inhibition was evident by the existence of all the subunits of fibrinogen as compared with ECV-treated samples.
Figure 6. Dose-dependent inhibition of pro-coagulant activity of ECV by ALME: ECV (1 μg) was pre-incubated with ALME (1:1–1:100 w/w) for 15 min at 37 °C. It was added to 200 μL of citrated human plasma containing 20 μL Tris-HCl buffer (10 mM, pH 7.4) and time taken for the formation of visible clot was recorded. To confirm the specificity of inhibition of ECV metalloprotease, thrombin time was done. Data represent mean ± SD (n = 3). *p < 0.05, **p < 0.01 and ***p < 0.001 compared with ECV.
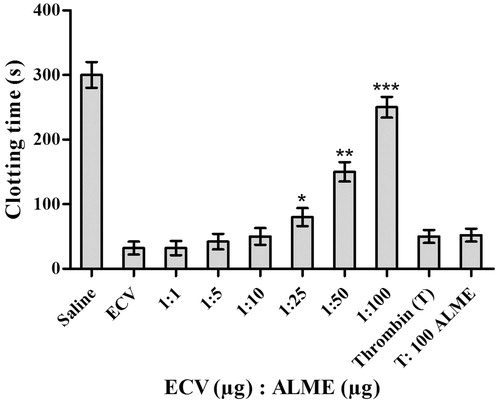
Figure 7. Dose-dependent inhibition of fibrinogenolytic activity of ECV by ALME: Fibrinogen (60 μg) was incubated with 2 μg of ECV + varying concentrations of ALME in 10 mM Tris-HCl pH 7.6 for 3 h and cleavage pattern was analyzed using 10% SDS-PAGE. (A) Fibrinogen alone; (B) fibrinogen +2 μg ECV; (C)–(F) 1:10, 1:25, 1:50 and 1:100 ALME (w/w), respectively.
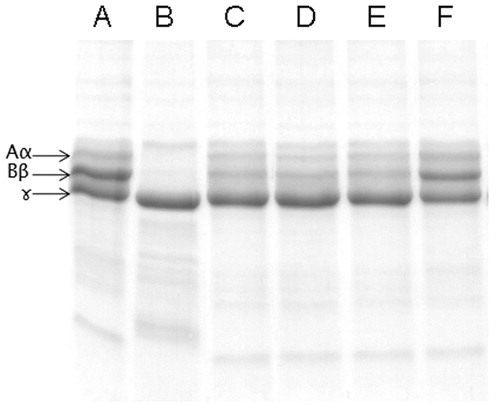
Even though proteases are associated with progressive tissue damage induced by ECV, the progression is largely contributed by the presence of hyaluronidases. Combinatorial action of these two enzymes plays a major role in spreading the toxic venom components from the bite site to the systemic circulation and eventually to multiple tissue targets. In addition, ECV hyaluronidases (ECVHYs) act to deplete hyaluronic acid holding the tissues together, thereby, resulting in loss of ECM integrity, eventually contributing to systemic spreading of locally acting proteases (Nanjaraj Urs et al. Citation2013). In this direction, ECVHYs inhibition studies by ALME were performed. ALME inhibited the hyaluronidase activity of ECV dose-dependently upon pre-incubation for 15 min with 100 μg of ECV. ALME inhibited hyaluronidase activity at the ratio of 1:100 w/w (50%; p < 0.0001; IC50 = 91.95 μg) ( and ). The ECV hyaluronidase inhibition was further confirmed by substrate gel assay. ALME also demonstrated inhibition of ECV hyaluronidase activity in zymography upon pre-incubation for 15 min with 100 μg of ECV, prior to electrophoresis at the ratio of 1:100 w/w (60%; p < 0.0001) ().
Figure 8. Dose-dependent inhibition of hyaluronidase activity of ECV by ALME: Reaction mixture (0.5 mL) contained 50 μL of hyaluronic acid (1 μg/μL in 0.1 M sodium acetate buffer containing 0.15 M NaCl, pH 8.5) incubated with 100 μg of ECV + different concentrations of ALME ranging from 1:1 to 1:100 w/w for 2.5 h at 37 °C. Data represent mean ± SD (n = 3). *p < 0.05, **p < 0.01 and ***p < 0.001 compared with ECV.
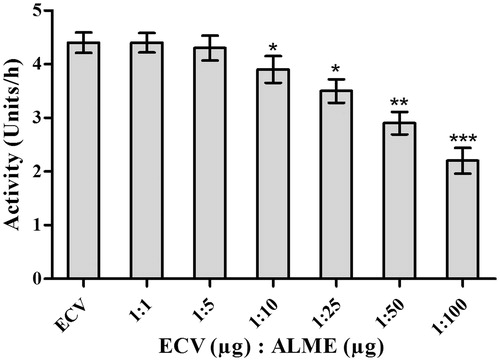
Figure 9. IC50/ED50 of antidote ALME against ECV: For the calculation of IC50/ED50 of ALME, activity (%) was plotted on the y-axis and ALME concentration in μg was plotted on the x-axis. To this plot, straight-line equation was obtained using MS-Excel (y = mx + c), in which y = activity (%) and x = ALME (μg). For IC50/ED50, we have substituted y = 50 and calculated x (IC50/ED50). (A) IC50 value of ECV protease; (B) IC50 value of ECV hyaluronidase; (C) ED50 value of haemorrhage neutralization; (D) and (E) ED50 value of myotoxicity neutralization (CK and LDH, respectively).
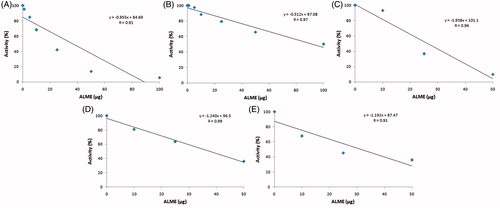
Figure 10. Inhibition of hyaluronidase activity of ECV by ALME: Hyaluronic acid was incorporated into the SDS polyacrylamide resolving gel matrix (0.05%). ECV (100 μg) and ECV pre-incubated with ALME for 15 min was loaded onto separate wells. (A) 100 μg ECV; (B) 1:100 [ECV (μg): ALME (μg)]. Data represent mean ± SD (n = 3). ***p < 0.001 compared with ECV.
![Figure 10. Inhibition of hyaluronidase activity of ECV by ALME: Hyaluronic acid was incorporated into the SDS polyacrylamide resolving gel matrix (0.05%). ECV (100 μg) and ECV pre-incubated with ALME for 15 min was loaded onto separate wells. (A) 100 μg ECV; (B) 1:100 [ECV (μg): ALME (μg)]. Data represent mean ± SD (n = 3). ***p < 0.001 compared with ECV.](/cms/asset/8c8bf1fb-e58d-46a2-88b8-372150f195cf/iphb_a_1171882_f0010_b.jpg)
In contrast to significant inhibition of proteolytic and hyaluronidase activities, ALME failed to inhibit PLA2 activity significantly (<30%) (data not shown).
Summary and conclusion
ALME demonstrated significant neutralization potential against the toxic enzymes of ECV (proteases and hyaluronidases). In addition, ALME prevented the characteristic ECV induced haemorrhage and myotoxicity following independent and co-injection studies in mice, thereby prevented local tissue damage and systemic spreading of ECV toxins. Based on the results, this study scientifically evaluated the ethno-medicinal use of A. lebbeck seed extract against snakebite. Therefore, ALME serves as a potent fraction for the isolation of phytochemical(s) with antivenom properties for the management of snakebite complications particularly at the site of envenomation.
Funding information
Authors thank University Grants Commission, New Delhi, and Institution of Excellence (funded by Ministry of Human Resource Development, Government of India), University of Mysore (UOM), Mysuru for Fellowship. Authors thank UGC-SAP, DST-PURSE and VGST for financial assistance to DOS in Biochemistry, UOM and Vijnana Bhavan, UOM.
Acknowledgements
Authors thank Institutional Animal and Human Ethical Committees (IAEC and IHEC) for ethical clearance. Authors thank Dr. K. A. Sharvani, herbarium in-charge, Yuvaraja's College, UOM for plant identification. Authors also thank G. Swamy, M. S. Sumanth, M. N. Savitha and G. V. Rudresha for their support during the work.
Disclosure statement
The authors have no conflicts of interests to disclose.
References
- Anonymous. 2001. Ayurvedic pharmacopoeia of India. 1st ed. (Part I, vol. III). Delhi: Government of India, The Ministry of Health & Family Welfare, Department of Ayush, The Controller of Publication, 201–202.
- Arruda EZ, Silva NM, Moraes RA, Melo PA. 2002. Effect of suramin on myotoxicity of some crotalid snake venoms. Braz J Med Biol Res. 35:723–726.
- Asad MHHB, Murtaza G, Siraj S, Khan SA, Azhar S, Hussain MS, Ismail T, Hussain MS, Hussain I. 2011. Enlisting the scientifically unnoticed medicinal plants of Pakistan as a source of novel therapeutic agents showing anti-venom activity. Afr J Pharm Pharmacol. 5:2292–2305.
- Asad MHHB, Sabih DE, Chaudhory BA, Ahmad I, Hussain MS, Izhar N, Akmal N, Shahzad AH, Hussain I. 2014. Anti-hemolytic property of local medicinal plant(s) upon Pakistani cobra venom induced hemolysis. J Anim Plant Sci. 24:1701–1708.
- Gomes A, Das R, Sarkhel S, Mishra R, Mukherjee S, Bhattacharya S, Gomes A. 2010. Herbs and herbal constituents active against snake bite. Indian J Exp Biol. 48:865–878.
- Hasson SS, Al-balushi MS, Said EA, Habbal O, Idris MA, Mothana RAA, Sallam TA, Al-Jabri AA. 2012. Neutralisation of local haemorrhage induced by the Saw-Scaled Viper Echis carinatus sochureki venom using ethanolic extract of Hibiscus aethiopicus L. Evid Based Complement Alternat Med. 2012:540671.
- Hiremath V, Nanjaraj Urs AN, Joshi V, Suvilesh KN, Savitha MN, Amog PU, Rudresha GV, Yariswamy M, Vishwanath BS. 2015. Differential action of medically important Indian BIG FOUR snake venoms on rodent blood coagulation. Toxicon. 110:19–26.
- Hiremath V, Yariswamy M, Nanjaraj Urs AN, Joshi V, Suvilesh KN, Ramakrishnan C, Nataraju A, Vishwanath BS. 2013. Differential action of Indian BIG FOUR snake venom toxins on blood coagulation. Toxin Rev. 33:23–32.
- Jha P, Chaloupka FJ, Moore J, Gajalakshmi V, Gupta PC, Peck R, Asma S, Zatonski W. 2006. Disease control priorities in developing countries. 2nd ed. Washington (DC): The International Bank for Reconstruction and Development/The World Bank Group.
- Juckett G, Zhancox JG. 2002. Venomous snakebites in the United States: management review and update. Am Fam Physician. 65:1367–1374.
- Kapoor LD. 2000. Handbook of ayurvedic medicinal plants: herbal reference library. Florida, United States: CRC Press LLC.
- Khare CP. 2008. Indian medicinal plants: an illustrated dictionary. New York: Verlag New York; Springer Science & Business Media.
- Kiritikar KR, Basu BD. 1935. Indian medicinal plants. 2nd ed., vol. II. Allahabad: Lalit Mahon Basu.
- Kornalik F, Blomback B. 1975. Prothrombin activation induced by ecarin – a prothrombin converting enzyme from Echis carinatus venom. Thromb Res. 6:57–63.
- Laemmli UK. 1970. Cleavage of structural proteins during the assembly of the head of bacteriophage T4. Nature. 227:680–685.
- Lowry OH, Rosebrough NJ, Farr AL, Randall RJ. 1951. Protein measurement with the Folin phenol reagent. J Biol Chem. 193:265–275.
- Minu V, Harsh V, Ravikant T, Paridhi J, Noopur S. 2012. Medicinal plants of Chhattisgarh with anti-snake venom property. Int J Curr Pharm Rev Res. 3:1–10.
- Mohamed R, Shivaprasad HV, Jameel NM, Shekar MA, Vishwanath BS. 2011. Neutralization of local toxicity induced by Vipera russelli phospholipase A2 by lipophilic derivative of ascorbic acid. Curr Top Med Chem. 11:2531–2539.
- Nanjaraj Urs AN, Yariswamy M, Joshi V, Nataraju A, Gowda TV, Vishwanath BS. 2013. Implications of phytochemicals in snakebite management: present status and future prospective. Toxin Rev. 33:1–24.
- Nanjaraj Urs AN, Yariswamy M, Joshi V, Suvilesh KN, Sumanth MS, Das D, Nataraju A, Vishwanath BS. 2015a. Local and systemic toxicity of Echis carinatus venom: neutralization by Cassia auriculata L. leaf methanol extract. J Nat Med. 69:111–122.
- Nanjaraj Urs AN, Yariswamy M, Ramakrishnan C, Joshi V, Suvilesh KN, Savitha MN, Velmurugan D, Vishwanath BS. 2015b. Inhibitory potential of three zinc chelating agents against the proteolytic, hemorrhagic, and myotoxic activities of Echis carinatus venom. Toxicon. 93C:68–78.
- Pierce RD, Kim ES, Girton LW, McMurry JL, Francis JW, Albrecht EA. 2011. Characterization of crude Echis carinatus venom-induced cytotoxicity in HEK 293T cells. J Venom Res. 2:59–67.
- Rajesh R, Shivaprasad HV, Gowda CD, Nataraju A, Dhananjaya BL, Vishwanath BS. 2007. Comparative study on plant latex proteases and their involvement in hemostasis: a special emphasis on clot inducing and dissolving properties. Planta Med. 73:1061–1067.
- Suntravat M, Nuchprayoon I, Perez JC. 2010. Comparative study of anticoagulant and procoagulant properties of 28 snake venoms from families Elapidae, Viperidae, and purified Russell's viper venom-factor X activator (RVV-X). Toxicon. 56:544–553.
- Tarannum S, Mohamed R, Vishwanath BS. 2012. Inhibition of testicular and Vipera russelli snake venom hyaluronidase activity by Butea monosperma (Lam) Kuntze stem bark. Nat Prod Res. 26:1708–1711.
- Tripathi RM, Sen PC, Das PK. 1979. Studies on the mechanism of action of Albizzia lebbeck, an Indian indigenous drug used in the treatment of atopic allergy. J Ethnopharmacol. 1:385–396.
- White J. 2005. Snake venoms and coagulopathy. Toxicon. 45:951–967.
- Yamada D, Sekiya F, Morita T. 1996. Isolation and characterization of carinactivase, a novel prothrombin activator in Echis carinatus venom with a unique catalytic mechanism. J Biol Chem. 271:5200–5207.
- Yariswamy M, Shivaprasad HV, Joshi V, Nanjaraj Urs AN, Nataraju A, Vishwanath BS. 2013. Topical application of serine proteases from Wrightia tinctoria R. Br. (Apocyanaceae) latex augments healing of experimentally induced excision wound in mice. J Ethnopharmacol. 149:377–383.