Abstract
Objectives. Inflammation is involved in cell proliferation and collagen deposition causing vessel wall remodeling and restenosis after plain balloon angioplasty. Local drug delivery of bioactive agents that reduce the incidence of adverse wall remodeling is of considerable interest concerning treatment strategies for coronary vessel disease and could alter the need of repeated revascularization. Design. In this study, 34 domestic pigs undergoing coronary balloon injury were randomly assigned to Tetradecylthioacetic acid (TTA) or placebo delivered locally. After four weeks, vessel wall collagen density, inflammatory markers and lipid fractions were assessed as well as cell proliferation. Results. Collagen particle count was lower after TTA compared to placebo, 177 ± 11 n/area versus 225 ± 13 n/area (p = 0.007). Interleukin-2 (IL-2) concentration was reduced, 1.6 ± 0.02 pg/ml versus 2.6 ± 0.5 pg/ml, (p = 0.01). The anti-inflammatory index was increased after TTA, 46.28 ± 12.1 versus 34.66 ± 4.5, (p = 0.025). There were no differences between TTA and placebo with regard to cell proliferation. Conclusions. Local delivery of TTA reduced the local inflammatory response and collagen accumulation. Local balloon delivery of TTA into the vessel wall may represent an alternative antiproliferative strategy for preventing restenosis, in particular for vessels with obstructive disease not available for stent implantation.
Introduction
Treatment of coronary artery disease by balloon angioplasty or stent implantation is limited by the development of restenosis which may occur several weeks or months after intervention. Drug-eluting stents (DES) have been shown to be effective in reducing the occurrence of restenosis and the need for repeat revascularization (Citation1–3). In such stents anti-inflammatory or anti-proliferative drugs are gradually eluted from the polymer coating on the surface of the stent during the first weeks after stent implantation. Similarly, using paclitaxel-coated balloons for angioplasty has been shown to be associated with lower restenosis rates in peripheral arteries as well as in small coronary vessels (Citation4,Citation5). However, the use of DES is limited by the size of the artery, and is confined to arteries larger than 2.25 mm in diameter. The use of DES in small vessel disease requires the use of dual platelet inhibition for months and there is also an increased risk for stent thrombosis. Effective treatment strategies for smaller vessels are therefore required.
We have previously shown that the sulfur containing Tetradecylthioacetic acid (TTA), given as per-oral supplements or locally delivered to the vessel wall, reduce restenosis after balloon angioplasty injury in the rabbit iliac model (Citation6) and porcine coronary arteries (Citation7). Further, TTA has been shown to have potent antioxidant and antiproliferative properties in vitro and in vivo and to reduce pro- inflammatory cytokines (interleukins-2, and -6, and tumor necrosis factor alpha (TNF-α)) (Citation6,Citation8,Citation9). However, when TTA is systemically administered the level of the anti-inflammatory interleukin-10 is increased. In the present paper we tested the hypothesis that local delivery of TTA inhibits coronary vessel wall inflammation, collagen accumulation, and cell proliferation after balloon angioplasty and thereby reduces constrictive remodeling and neointimal formation in a porcine model.
Materials and methods
Study design
A well-established porcine model was used for assessing the responses of the vessel wall following transluminal balloon angioplasty (Citation10,Citation11). The study was performed in a total of 34 pigs in 2 separate series (). All pigs underwent percutaneous coronary balloon injury. TTA or placebo was administered at the injury site via a local drug delivery angioplasty balloon.
Figure 1. Study design. TTA = Tetradecylthioacetic acid, RCA = right coronary artery, LCX = left circumflex coronary artery, BrdU = bromodeoxyuridine, AIFAI = anti-inflammatory fatty acid index.
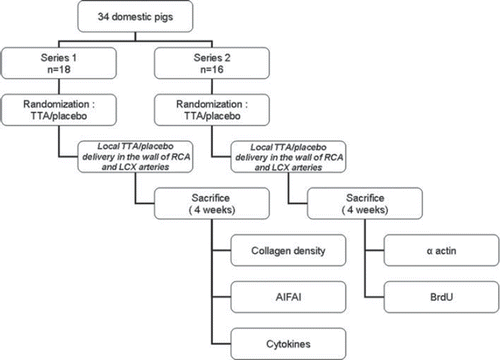
Series 1: Eighteen domestic pigs were randomly assigned to either TTA or placebo locally delivered into the wall of the right coronary (RCA) and left circumflex (LCX) arteries after percutaneous balloon injury. Animals were sacrificed 4 weeks later. The RCA and LCX were isolated and fixed until further histomorphometric analysis of collagen density or frozen for biochemical analysis of fatty acids composition, anti-inflammatory fatty acid index, and enzyme-linked immunosorbent assay (ELISA) of inflammatory cytokines.
Series 2: Sixteen pigs undergoing the same percutaneous balloon injury were randomly assigned to either TTA or placebo as in series 1 and sacrificed 4 weeks later. RCA and LCX were isolated and prepared for analysis of cell proliferation by immunohistochemistry using antibodies against BrdU, whereas antibodies against α-actin were used for detection of smooth muscle cells and myofibroblasts.
Animals
Domestic farm pigs (Sus scrofa, weight 40 kg) of either sex were used in the study. A health certificate confirming the absence of serious infections (Scabies, Pasteurella multocida, Shigella dysenteriae and Mycoplasma pneumonia) followed each pig. Animals arrived at least 7 days before the procedure for acclimatization. The pigs were kept under controlled environmental conditions with temperature of 20°C, a relative humidity of 40% and 15–20 air changes per hour. The lighting was artificial, and on for 12 hours/off for 12 hours with sunset-/sunrise dimming. Pigs were fed twice daily on a standard diet (Format Kombi Fri Sur, FK) without cholesterol supplementation. The study was approved by the local ethics for animal-care and use. The protocol conforms to the Guide for the Care and Use of Laboratory Animals published by the US National Institutes of Health (NIH Publication No. 85–23, revised 1996).
Percutaneous coronary injury and local drug delivery
Prior to the procedure, premedication was given as a combination of ketamine/methomidine and atropine. Aspirin 300 mg was given as a single dose 24 hours before the procedure. General anesthesia was achieved by gas stream of 5% Isoflurane in 40% oxygen and 60% nitrous oxide. Blood pressure, heart rate and ECG were monitored during the procedure. A combination of ketorolac and diazepam was administered for analgesia before the surgical cut down. Ketorolac was repeated after the procedure and two times the first postoperative day. After infiltrating the skin with 1% Xylocaine for local anesthesia, the right femoral artery was exposed and a 6F sheath was inserted and anchored with ligatures. A bolus of 100 IU/kg unfractionated heparin was given before percutaneous intervention.
A 6F multi-purpose hockey stick catheter or Judkin right served as guiding catheters for coronary cannulation. Coronary angiography was performed in 2 orthogonal views (RAO and LAO 25 degrees). The angioplasty balloon catheter was advanced into the coronary arteries over a floppy guide wire 0.014. Coronary artery injury was performed on the LCX and RCA using an oversized semi compliant angioplasty balloon 20 mm long (balloon/vessel ratio 1.3) expanded to 12 atmospheres for 30 seconds. In both vessels, the distal end of the injured area was marked with a stainless steel coronary stent deployed at 12 atmospheres for 15 seconds. The left anterior descending artery was used as a control. TTA was dissolved in 0.9% saline and heated to 75°C until a clear solution was obtained. Then 5% bovine serum albumine (BSA) was added to prevent precipitation. A final concentration of 9 mM TTA was used. Placebo solution consisted of 0.9% saline and 5% BSA. Two ml of TTA/placebo solutions were administered at the injury segment, proximal to the stented segment using a Transport® multiporous angioplasty balloon catheter (Boston Scientific Corporation, USA). To ensure direct apposition of transport balloon with the vessel wall, the balloon was inflated up to 6 atmospheres during delivery. Delivery was performed in 2 steps; 1 ml delivered over 30 seconds, the balloon was then deflated and withdrawn proximally to avoid ischemia. The balloon was thereafter repositioned, and another 1 ml was injected at the injury site. By the end of the procedure, catheters and wires were removed, the femoral artery was repaired, and anesthesia was reversed.
Harvesting and collagen density analysis
Median sternotomy was performed under general anesthesia. Animals were sacrificed by intra-atrial injection of 20 ml potassium chloride. The heart was exposed and excised. The RCA with the underlying myocardial segment was excised, flushed with a solution containing ringer acetate and heparin, and fixed by infusion of 4% formalin, pH 7.4 at a pressure of 100 mmHg in 15 minutes. The implanted stent served as a marker to identify the distal site of injury. Using a transport balloon catheter (balloon-length 20 mm) the proximal and distal part of injury/delivery site were marked by needles. The coronary artery between the needles, representing the injury/delivery site, was then excised. All peri-vascular tissue was excised and the artery segment was dehydrated in ethanol and xylene, embedded sequentially in paraffin, and then sectioned (4 μm) every 1(-2) mm. Paraffin sections were de-waxed and hydrated, stained in picrosirius red for 1 hour, then washed with acidified water, dehydrated with ethanol, cleared in xylene and mounted in a resinous medium (). Picrosirius-labeled collagen was quantified by use of a computer-interfaced color imaging system. True color images were transferred from the light microscope via a digital video camera to the image analysis software (Olympus BX51, analysis® by Soft Imaging System) to measure the fractional areas of collagen in stained sections. Collagen measurements were performed on the entire neointima, media, and adventitia. Each vessel layer was divided into 10 equal regions of interest (ROI). Collagen density was measured in each ROI as expressed as particle counts, and total density was calculated by adding densities of all ROIs. All measurements were performed by an independent assessor, blinded to animal randomization.
Cytokines analysis
Cytokine analysis including interleukins (IL2, IL6), and tumor necrosis factor alpha (TNF-α), was performed using the SEARCHLIGHT® Porcine Cytokine Array (Pierce Biotechnology, Inc. Rockford, IL. 61105 U.S.A). It is a multiplex sandwich ELISA (enzyme-linked immunosorbent assay) for the quantitative measurement of cytokines. Each well of the microplate provided was pre-spotted with cytokine-specific antibodies. These antibodies capture specific cytokines in the standards and samples added to the plate. After unbound proteins were washed away, the biotinylated detecting antibodies were added and attached to a second site on the target proteins. After washing away excess detecting antibody, streptavidin-horseradish peroxidase (SAHRP) was added. This labeled-antibody complex was visualized with SUPERSIGNAL® ELISA Femto Chemiluminescent Substrate (Thermo Fisher Scientific Inc., Rockford, IL, USA) was detected with a cooled CCD camera.
Fatty acid composition
Lipids were extracted from the arterial wall and analyzed as previously described (Citation12,Citation13). The anti-inflammatory fatty acid index (AIFAI) (C20:3n-6 + C20:5n-3 + C22:5n-3 + C22: 6n-3/C20:4n-6) was calculated as described elsewhere (Citation14).
Immunohistochemistry
BrdU (SDS Biosciences, Falkenberg, Sweden) was administered as oral supplementations in all animals in a 2 week period started at the day of the procedure. Animal sacrifice was done 4 weeks after the procedure as described above. RCA and LCX were isolated and shock-frozen in liquid nitrogen-chilled isopentane and stored at −80°C. Cryostat sections (4 μm) were chemically stabilized with a 3.7% neutral buffered formaldehyde solution, made permeable with 0.1% Triton X-100, incubated in a 0.2 M glycine solution and then incubated with normal serum for 30 minutes and thereafter with primary antibodies. For the labeling of α-actin, specific monoclonal antibodies (DAKO, Glostrup, Denmark) were applied for 1 hour at room temperature at a dilution of 1:100. Sections were thereafter incubated with fluorescence isothiocyanate (FITC)-conjugated secondary antibodies (Sigma, Saint Louis, MO, USA) diluted 1:40. Similarly, for visualization of BrdU, sections were stained with mouse anti-BrdU antibodies (ICN Pharmaceuticals Inc., Aurora, OH, USA) at a dilution of 1:10 and then visualized with the FITC-conjugated secondary antibodies. Antibody-labeled sections were then viewed by a video camera and digitized by a computer equipped with an image analysis system (Quantinet WIN Color; Cambridge software, Cambridge, MA, USA)
Statistics
Data are presented as mean ± SEM. The two- independent sample t-test was performed for analyzing for any statistical differences between the groups. Level of evidence, p < 0.05, was considered of statistical significance. Data analysis was performed by SPSS version 14 (SPSS Inc.Chicago, IL, USA).
Results
Collagen
Collagen depositions across the vessel wall were expressed by collagen particle counts and the particle count from the entire wall was significantly reduced after intervention with TTA compared to placebo, 177 ± 11 n/area versus 225 ± 13 n/area (p = 0.007). When analyzing the different wall layers, a significantly lower count was detected in the adventitia (239 ± 20 n/area versus 325 ± 17 n/area; p = 0.002), whereas a numeric but non-significant reduction between TTC and placebo-treated vessels was accounted for in the intima and media (42 ± 8 n/area versus 52 ± 13 n/area, p = 0.1; and 250 ± 19 n/area versus 297 ± 24 n/area, p = 0.3, respectively).
Cytokines
The mean values of inflammatory cytokines showed that TTA significantly inhibited the local release of IL2 compared to placebo 1.6 ± 0.02 pg/ml, versus 2.6 ± 0.5 pg/ml, p = 0.01). In addition, there was a tendency to decreased IL6 levels in TTA-treated animals compared to the placebo group (5.4 ± 1.5 pg/ml versus 10.6 ± 2.2 pg/ml, p = 0.07). No significant difference was detected for TNFα. (p = 0.1)
Fatty acids composition and AIFAI
We investigated whether local delivery of TTA could affect the fatty acid compositions in the arterial wall after balloon injury. Traces of TTA were detected in the injured wall segments at 4 weeks. However, no changes were seen in the total level of saturated, monounsaturated, or polyunsaturated fatty acids levels, compared to the placebo-treated vessels, or the arterial wall adjacent to the injury segment. Moreover, the ratio of the free levels of omega- 3 and omega-6 was not changed by TTA. The level of arachidonic acid (C 20:4 n-6; expressed as weight % of total fatty acids) was significantly reduced in the arterial wall after TTA compared to placebo (1.7 ± 1, versus 2.3 ± 0.7, p = 0.02). This fatty acid change was accompanied by a significantly reduced ∆-5 desaturase index of n-6 fatty acid (5.12 ± 1 versus 6.6 ± 1.3, p = 0.02), whereas the -6 desaturase index was not changed. The anti-inflammatory fatty acid index increased significantly in the arterial wall of animals treated by TTA compared to placebo (46.28 ± 12.1 versus 34.66 ± 4.5, p = 0.025).
α-actin and BrdU
The number of α-actin particles per unit area was not significantly different between the TTA and placebo groups when examined for the entire vessel wall (136 ± 16 n/area versus 101 ± 10 n/area, p =0.07), or separately for the different layers; adventitia (219 ± 21 n/area versus 183 ± 18 n/area, p = 0.2); media (136 ± 33 n/area versus 78 ± 15 n/area, p = 0.1); intima (53 ± 14 n/area versus 42 ± 6 n/area, p = 0.5), respectively. The mean BrdU area was similar in both groups (30 ± 3 μm2 versus 29 ± 3 μm2, p = 0.8).
Discussion
Coronary restenosis after angioplasty is a result of cellular proliferation and vessel constriction with the latter as the more dominant. The radial strength of stents have greatly reduced the importance of constriction and antiproliferative agents used as stent coatings have been very successful to counteract coronary restenosis. Subsets of patients may not be amenable for stenting and in patients undergoing plain balloon angioplasty constriction may therefore still be an important focus area. We have previously shown that TTA reduces coronary stenosis after balloon angioplasty by an inhibiting effect on constrictive remodeling in a porcine model (Citation7). The present study was designed to further identify the possible molecular mechanisms of the TTA-induced effects. We found that TTA inhibited the inflammatory response after coronary injury up to 4 weeks after local delivery. Further, it was demonstrated that TTA significantly reduces the total collagen accumulation within the vessel wall, while no significant effects on cell proliferation or distribution of α-actin positive particles were observed.
Local delivery of drugs to artery segments in order to prevent restenosis has been achieved in a number of studies by using drug-eluting stents or by drug-coated balloons (Citation1–5). The drugs used include rapamycin (sirolimus and derivatives) and paclitaxel which have different modes of action. Rapamycin binds to intracellular proteins and the complex inhibits the mTOR-mediated signal-transduction pathways, resulting in the arrest of cell cycle in G1 phase as well as immune suppression (Citation15). Paclitaxel interferes with the normal breakdown of microtubules during cell division and blocks cells in the G2/M phase of the cell cycle. Both these groups of drugs have been best documented using drug-eluting stents whereas the effects in unstented vessels are more scarcely documented. The mechanisms of restenosis in stented and unstented arteries are likely to be different and may therefore require different pharmacological approaches to reduce restenosis.
It has been suggested that wall remodeling rather than neointimal formation explains late lumen loss after vessel injury (Citation16–18). Theoretically, arterial shrinkage requires collagen formation and contractile elements, such as α-actin-positive cells. In our study, we measured collagen density at 4 weeks after injury in accordance with prior reported angiographic and histomorphometric findings that found lumen loss and constrictive remodeling taking place between days 7 and 28 after injury (Citation17,Citation18). Changes in collagen content, subtype composition, and biosynthesis after angioplasty have been investigated in several different animal models. Prior studies reported a significant increase in collagen content and biosynthesis 4 weeks after angioplasty (Citation19–21). In an atherosclerotic rabbit model collagen was shown to make up >50% of vascular protein content by 30 days after angioplasty (Citation20). Strauss et al. (Citation21) reported a 4–10 fold increase in collagen, elastin, and glycoprotein synthesis after angioplasty. However, the correlation between collagen content and vessel wall remodeling remains controversial (Citation18), and in some studies collagen content was reported to be significantly decreased in restenotic versus non- restenotic vessels after angioplasty (Citation22). Kingston et al. (Citation23) using a porcine model found that antagonism of TGF-ß1 stimulates the formation of a dense collagenous adventitia which was suggested to prevent constrictive remodeling by acting as an external scaffold. Moreover, Schmidt et al. (Citation18) showed that the accumulation of actin-positive cells and collagen takes place in neointima occurring concomitantly with luminal narrowing. Lafont et al. (Citation24) attempted to quantify structural (ie, collagen) alterations that occur after angioplasty and to evaluate their respective relations with restenosis and arterial remodeling in atherosclerotic rabbit femoral arteries. They found positive correlation between restenosis and collagen accumulation in neointima and media. These findings are consistent with those from Tyagi et al. (Citation25) who detected collagen accumulation and decreased elastin density in human coronary atherectomy restenotic samples. It is hypothesized that posttranslational alteration of collagen, such as cross-linking, may also contribute to constrictive remodeling as “scar contraction”, and inhibition of collagen cross-linking influences the remodeling process and eventually reduces restenosis (Citation18).
In the present study, cell proliferation after coronary angioplasty was not inhibited by TTA. Formation of neointima, including smooth muscle cell proliferation has traditionally been considered to represent an important cause of restenosis (Citation17). It was, therefore, hypothesized that TTA may have antiproliferative properties (Citation26). In vitro, Kuiper et al. (Citation6) showed that TTA inhibits smooth muscle cell proliferation. This finding, however, was not repeated in the present in vivo study. This may also be related to dose-dependent effects of TTA (Citation7).
Higher doses of TTA, and or interaction between stent material and TTA, in addition to the differences in the adverse response to injury between balloon angioplasty and stent, may explain the shift from the anti-inflammatory to proinflammatory properties of TTA when loaded on stents. Thus, the inflammatory modulation of TTA seems to vary according to dose, type of intervention and time course following intervention.
Inflammatory cytokines, released from activated macrophages and lymphocytes, stimulate collagen synthesis by smooth muscle cells and myofibroblasts with subsequent formation of the extracellular matrix (Citation27–29). The inhibitory effects of TTA on collagen accumulation observed in this study may be partially linked to its anti-inflammatory properties as a peroxisome proliferator-activated receptor (PPARs) agonist. TTA has been shown to be a potent peroxisome proliferator (Citation26), and the anti-inflammatory effects of PPARs α and γ agonists have been previously verified (Citation30). The present results, therefore, support the theory that inflammation and collagen deposition play important roles in the development of vascular lesions after balloon angioplasty (Citation29).
In conclusion, local delivery of TTA, a sulfur containing fatty acid, inhibits inflammation and subsequent collagen accumulation within the vessel wall after coronary injury. These properties may be linked to its counteraction of adverse remodeling after balloon angioplasty, and may represent an alternative approach for preventing restenosis.
Declaration of interest: The authors report no conflicts of interest. The authors alone are responsible for the content and writing of the paper.
References
- Morice M, Serruys PW, Sousa JE, Fajadet J, Ban Hayashi E, Perin M, . A randomized comparison of a sirolimus-eluting stent with a standard stent for coronary revascularization. N Engl J Med. 2002;346:1773–80.
- Moses JW, Leon MB, Popma JJ, Fitzgerald PJ, Holmes DR, O’Shaughnessy C, . Sirolimus-eluting stents versus standard stents in patients with stenosis in a native coronary artery. N Engl J Med. 2003;349:1315–23.
- Stone GW, Lansky AJ, Pocock SJ, Gersh BJ, Dangas G, Wong SC, . Paclitaxel-eluting stents versus bare-metal stents in acute myocardial infarction. N Engl J Med. 2009; 360:1946–59.
- Unverdorben M, Kleber FX, Heuer H, Figulla HR, Vallbracht C, Leschke M, . Treatment of small coronary arteries with a paclitaxel-coated balloon catheter. Clin Res Cardiol. 2010;99:165–74.
- Tepe G, Zeller T, Albrecht T, Heller S, Schwarzwälder U, Beregi JP, . Local delivery of paclitaxel to inhibit restenosis during angioplasty of the leg. N Engl J Med. 2008; 358:689–699.
- Kuiper KKJ, Muna ZA, Erga KS, Dyrøy E, Svendsen E, Berge RK, . Tetradecylthioacetic acid reduces stenosis development after balloon angioplasty injury of rabbit iliac arteries. Atherosclerosis. 2001;158:269–75.
- Pettersen RJ, Muna ZA, Kuiper KKJ, Svendsen E, Muller F, Aukrust P, . Sustained retention of tetradecylthioacetic acid after local delivery reduces angioplasty-induced coronary stenosis in the minipig. Cardiovasc Res. 2001;52: 306–13.
- Muna ZA, Bolann BJ, Chen X, Songstad J, Berge RK. Tetradecylthioacetic acid and tetradecylselenoacetic acid inhibit lipid peroxidation and interact with superoxide radical. Free Radic Biol Med. 2000;28:1068–78.
- Muna ZA, Gudbrandsen OA, Wergedahl H, Bohov P, Skorve J, Berge RK. Inhibition of rat lipoprotein oxidation after tetradecylthioacetic acid feeding. Biochem Pharmacol. 2002;63:1127–35.
- Schwartz RS, Huber KC, Murphy JG, Edwards WD, Camrud AR, Vliestra RE, . Restenosis and proportional neointimal response to coronary artery injury: results in a porcine model. J Am Coll Cardiol. 1992;19:267–74.
- Schwartz RS, Murphy JG, Edwards WD, Comrud AR, Vliestra RE, Holmes DR. Restenosis after balloon angioplasty. A practical proliferative model in porcine coronary arteries. Circulation1990;82:2190–200.
- Gudbrandsen OA, Dyrøy E, Bohov P, Skorve J, Berge RK. The metabolic effects of thia fatty acids in rat liver depend on the position of the sulfur atom. Chem Biol Interact. 2005;155:71–81.
- Wergedahl H, Liaset B, Gudbrandsen OA, Lied E, Espe M, Muna Z, . Fish protein hydrolysate reduces plasma total cholesterol, increases the proportion of HDL cholesterol, and lowers acyl-CoA: cholesterol acyltransferase activity in liver of Zucker rats. J Nutr. 2004;134:1320–7.
- Chavali SR, Zhong WW, Utsunomiya T, Forse RA. Decreased production of interleukin-1-beta, prostaglandin-E-2, and thromboxane-B-2, and elevated levels of interleukin-6 and -10 are associated with increased survival during endotoxic shock in mice consuming diets enriched with sesame seed oil supplemented with Quil-A saponin. Int Arch Allergy Immunol. 1997;114:153–60.
- Sehgal SN. Sirolimus: its discovery, biological properties, and mechanism of action. Transplant Proc. 2003;35:7S–14S.
- Andersen HR, Maeng M, Thorwest M, Falk E. Remodeling rather than neointimal formation explains luminal narrowing after deep vessel wall injury: insights from a porcine coronary (re)stenosis model. Circulation. 1996;93:1716–24.
- Maeng M, Olesen PG, Emmertsen NC, Thorwest M, Nielsen TT, Kristiansen BØ, . Time course of vascular remodeling, formation of neointima and formation of neoadventitia after angioplasty in a porcine model. Coron Artery Dis. 2001;12:285–93.
- Schmidt MR, Maeng M, Kristiansen SB; Andersen HR, Falk E. The natural history of collagen and alpha-actin expression after coronary angioplasty. Cardiovasc Path. 2004; 13:260–7.
- Coats WD, Cheung DT, Han B, Currier JW, Faxon DP. Balloon angioplasty significantly increases collagen content but does not alter collagen subtype I/III ratios in the atherosclerotic rabbit iliac model. J Mol Cell Cardiol. 1996;28:441–6.
- Karim MA, Miller DD, Farrar MA, Elefheriades E, Redyy BH, Brelan CM, . Histomorphometric and biochemical correlates of arterial procollagen gene-expression during vascular repair after experimental angioplasty. Circulation. 1995;91:2049–57.
- Strauss BH, Chisholm RJ, Keeley FW, Gottlieb AI, Logan RA, Faxon DP. Extracellular-matrix remodeling after balloon angioplasty injury in a rabbit model of restenosis. Circ Res. 1994;75:650–8.
- Coats WD, Whittaker P, Cheung DT, Currier JW, Han B, Faxon DP. Collagen content is significantly lower in restenotic versus nonrestenotic vessels after balloon angioplasty in the atherosclerotic rabbit model. Circulation. 1997;95: 1293–300.
- Kingston PA, Sinha S, David A, Castro MG, Lowenstein PR, Heagerty AM. Adenovirus-mediated gene transfer of a secreted transforming growth factor-beta type II receptor inhibits luminal loss and constrictive remodeling after coronary angioplasty and enhances adventitial collagen deposition. Circulation. 2001;104:2595–601.
- Lafont A, Durand E, Samuel JL, Besse B, Addad F, Levy BI, . Endothelial dysfunction and collagen accumulation: two independent factors for restenosis and constrictive remodeling after experimental angioplasty. Circulation. 1999;100:1109–15.
- Tyagi SC, Meyer L, Schmaltz RA, Reddy HK, Voelker DJ. Proteinases and restenosis in the human coronary artery: extracellular matrix production exceeds the expression of proteolytic activity. Atherosclerosis. 1995;116:43–57.
- AbdiDezfuli F, Fr yland L, Thorsen T, Aakvaag A, Berge RK. Eicosapentaenoic acid and sulphur substituted fatty acid analogues inhibit the proliferation of human breast cancer cells in culture. Breast Cancer Res Treat. 1997;45:229–39.
- Forrester JS, Fishbein M, Helfant R, Fagin J. A paradigm for restenosis based on cell biology: clues for the development of new preventive therapies. J Am Coll Cardiol. 1991;17:758–69.
- Hayashi S, Watanabe N, Nakazawa K, Suzuki J, Tsushima K, Tamatoni T, . Roles of P-selectin in inflammation, neointimal formation, and vascular remodeling in balloon-injured rat carotid arteries. Circulation. 2000;102:1710–7.
- Libby P, Schwartz D, Brogi E, Tanaka H, Clinton SK. A cascade model for restenosis. A special case of atherosclerosis progression. Circulation. 1992;86:47–52.
- Verges B. Clinical interest of PPARs ligands - particular benefit in type 2 diabetes and metabolic syndrome. Diabetes Metabol. 2004;30:7–12.