Abstract
Objectives. Transcatheter aortic valve implantation (TAVI) is currently expanding worldwide, however all available prostheses share some fundamental design drawbacks. We investigated the feasibility, safety and hemodynamic performance of the innovative transapical Acurate TA™ self-expandable device, which has the unique advantage of offering anatomically correct self-alignment within the aortic root. Design. Transapical TAVI was performed in six acute swine and six chronic sheep procedures, with follow-up of 7, 14, 21, 28, 60 and 90 days. TAVI was performed under TEE and angiographic guidance without rapid pacing. Results. A partial sternotomy approach was used to access the LV-apex. All valve implantations were performed as planned and all animals survived the implantation procedure. After deployment, no migration, embolization or coronary obstruction was observed during the observation period. Intraoperative TEE examination identified no signs of intravalvular leakage or valve dysfunction. Transvalvular mean pressure gradients were 5.4 ± 2.2 mmHg decreasing during follow-up (1.6 ± 0.8 mmHg, 1.8 ± 0.8 mmHg, 1.3 ± 0.2, 1.8 ± 0.7 mmHg, 1.6 ± 0.8 mmHg), with a slight increase atday 90 (4.0 ± 2.4 mmHg, P < 0.05). Macroscopic examination at necropsy showed correct anatomical positioning of the valve stent without any signs of structural valve deterioration. Conclusions. These first results of the innovative self-expandable transapical ACURATE TA™ device explore the feasibility and safety of anatomically correct off-pump implantation with optimal hemodynamic results.
Introduction
Transcatheter aortic valve implantation (TAVI) techniques pose the possibility of causing a paradigm shift in the management of patients with aortic stenosis, due to avoidance of significant operative and procedural risks arising from sternotomy, cardiopulmonary bypass and ischemic myocardial arrest (Citation1,Citation2). Transapical access, which in the majority of cases is performed with the balloon-expandable Sapien™ prosthesis, represents a breakthrough in the treatment of selected high-risk patients (Citation3). However, further development of transcatheter devices continues to be challenging, and several key obstacles must be overcome. All currently available aortic valve prostheses models share some fundamental design issues, including positioning-, deployment-, vascular- and conduction-related complications (Citation4). The transapical ACURATE TA™ device (Symetis SA, Ecublens, Switzerland) consists of a radially self-expanding Nitinol® stent, providing optimal, anatomically correct self-alignment within the aortic root. Our intention was to evaluate the feasibility and safety of implantation, as well as the hemodynamic performance during follow-up of this newly-designed aortic valve prosthesis, using an antegrade transapical approach, in both acute pig and chronic sheep experiments. These animal experiments provided amongst others, were the basis for the first human implants within the “First-In-Man” trial and later CE-mark approval of the ACURATE TA™ device.
Methods
Experimental evaluation was performed on 6 pigs and 6 sheep after institutional review board approval. Experiments were carried out between August 2009 and November 2010. All acute pig experiments were performed at the experimental animal laboratory of the University Hospital Essen, Germany (UHE). Chronic sheep experiments were performed at IMM Recherche, Institute Mutualiste, Montsouris, Paris, France (IMM). The experiment protocol was reviewed and approved by the committee on Research Animal Care, Land Nordrhein-Westfalen (Landesamt für Natur, Umwelt und Verbraucherschutz Nordrhein-Westfalen, AZ 8.87–51.05.30.10.060). All animals received humane care in compliance with the “Principles of Laboratory and Animal Care” and the “Guide for the Care and Use of Laboratory animals” prepared by the Institute of Laboratory Animal Resources, published by the National Institutes of Health (NIH publication no. 85–23, revised 1985), and in accordance with the “1996 NRC Guide for the Care and Use of Laboratory animals.”
Six male German landrace pigs were used for the acute valve implantation experiments, and 6 sheep for chronic, follow-up evaluation. Induction of anaesthesia was performed with a combination of ketamine (30 mg/kg bw), azaperon (2 mg/kg bw) and atropine (2 mg total dose) intramuscularly, and continued with disoprivan (10 mg/h), midazolam (1 μg/kg‐1/h‐1) and fentanyl (5 μg/kg‐1/h‐1) administered intravenously. Mechanical ventilation (Oxylog® 1000, Dräger, Lübeck, Germany) was performed in a pressure-controlled mode, with a ventilation rate of 10–20 breaths/min (according to end-expiratory CO2) and a maximum inspiratory pressure of 25 mmHg, at an inspiratory oxygen-tension (FiO2) of 1.0.
Technical equipment
Both laboratories (UHE and IMM) were equipped to perform routine cardio-surgical operations. All implantations were performed in a sterile setting with full anaesthesiological, surgical and angiographic equipment, by an experienced and qualified (Federation of European Laboratory Animal Science Association certification) team, including one attending veterinarian. The setup (UHE) included a monoplane fluoroscopic angiography system (Siemens Artis Zee, Siemens, Munich, Germany) and a C-arm (Angiograph OEC 9900 Elite, GE Healthcare, Waukesha, WI, USA) monoplane visualization system (IMM). The fluoroscopic and angiographic imaging of the aortic root during the procedure was of paramount importance, and was performed prior to implantation to obtain an optimal perpendicular view of the implantation site. Different angulations of the system were tested during this study, rather than using a fixed standard projection. In addition, a pigtail-catheter was placed deep within the right coronary sinus to further facilitate positioning by providing a reliable landmark for correct alignment of the prosthesis.
Device
The ACURATE TA™ device (Symetis SA, Ecublens, Switzerland) consists of a self-expandable Nitinol® stent, available in three sizes of 23, 25 and 27 mm (equal to S, M and L), covering an aortic annulus diameter of between 21 and 27 mm. This self- expandable Nitinol® stent acts as an anchoring structure within the native aortic annulus, consisting of an upper and lower crown, enabling correct valve fixation in a sub-coronary position. Three additional stabilisation arches within the outflow tract are used to orientate the bioprosthesis in the ascending aorta during deployment (). A three-leaflet porcine valve (already with CE-mark), composed of three independent porcine non-coronary leaflets is fixed within the lower part of the Nitinol® stent. A double PET-skirt (polyethylene terephthalate) covers the inner and outer surface of the lower crown to reinforce the biological porcine valve, and thereby avoid any direct contact between the biologic tissue and the metallic stent struts. This additional PET-skirt seals the cells of the Nitinol® stent frame and guarantees impermeability of the stent at aortic annular level in order to prevent leakage. The whole system enables a sheath-less implantation (28F equivalent) via the apex of the left ventricle. The valve prosthesis is contained within the distal section of the delivery device (). The valve is released by an “unsheathing” process, initiated by pulling back the trigger lever of the delivery device.
Delivery sequence
The delivery device was advanced past the aortic valve. Anatomically correct orientation was then confirmed by rotation of the device; one commissural post of the valve-stent was aligned with the centre of the fluoroscopic projection, in order to confirm whether the commissure was in an anterior or posterior position. Using clockwise rotation, the commissure of the valve stent was rotated toward the left main coronary artery, to ensure an anterior position. Three radiopaque markers provided additional information during this rotation. The valve was unsheathed, releasing the stabilisation arches within the ascending aorta. These arches are used to orientate the bioprosthesis during the deployment phase, and avoid it from tilting. The valve was then partially released (upper crown), and the longitudinal position of the valve-stent was corrected since the operator can ‘feel’ the proper placement at the native annulus by gently pulling the ACURATE TA™ down toward the left ventricle. Once an optimal position was achieved, the inferior crown was released, and the valve self-aligned within the aortic annulus. The whole delivery sequence is illustrated in .
Figure 2. Delivery sequence: (A) Intra-annular positioning of the device (blue bars indicating the annular level), (B) anatomical correct rotation (blue arrow) of the device, (C) partial unsheathing and pulling of the device (blue arrow) and placement of the upper crown, (D) after correct placement of the upper crown the lower crown (E) is released with the valve self-aligned within the aortic annulus (F).
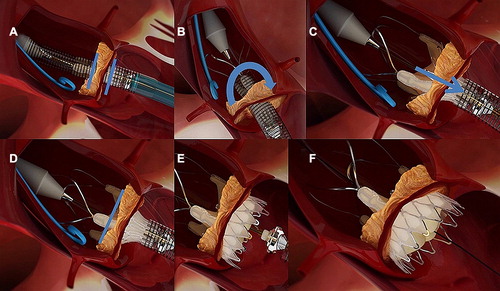
Surgical procedure
After induction of anaesthesia (as described above), a surgical tracheostomy was performed (acute experiments) or animals were intubated (size 7–8 mm tube). Anaesthesia was performed at the time of surgery (day 0), for follow-up echocardiography and on the day of sacrifice. ECG was connected and after administration of 5.000 IU of heparin, an arterial catheter was placed within the left carotid artery (acute experiments) or within the ear/femoral artery (chronic experiments) for hemodynamic monitoring. A venous catheter was placed within the right jugular vein for intravenous anaesthesia and an additional 6F sheath was placed within the femoral artery allowing for catheterization and angiography during the procedure. After partial median sternotomy and longitudinal incision of the pericardium, three to four pledged-armed apical U-strings (Prolene 3-0, MH needle) were placed in a circular, ‘star-like’ formation on the left ventricular apex, with sufficiently deep stitches through the myocardium above the apex on the anterior wall, and lateral to the left anterior descending coronary artery. All further steps were performed under fluoroscopic control with additional echocardiography. Transoesophageal echocardiography (TEE) guidance was used to aid with annular sizing, valve deployment and final evaluation of valve performance. TEE was performed with a multiplane 2.9–6.7 MHz (6T-RS) phased-array-probe (Vivid i, GE Healthcare, Waukesha, WI, USA) or with a MATRIX iE33 echocardiography system (Philips, Amsterdam, Netherlands). Under fluoroscopic and echocardiographic guidance, an 18 gauge percutaneous needle was used to puncture the apex, and a 0.035” guidewire was advanced through the needle into the left ventricle. After crossing the aortic valve, the aortic arch was crossed with a right coronary diagnostic catheter, and using the Seldinger technique a 0.035” super-stiff guidewire was placed within the descending aorta. A 14F delivery sheath was then placed transapically into the left ventricle (LV). The 14F sheath was then removed, before the crimped valve together with the delivery system was bluntly inserted, without the use of a sheath, through the apex of the LV. Valve positioning and implantation then followed, as described above. All six pigs were sacrificed after final valve deployment, and a pathological examination of the heart was performed to evaluate valve position. All sheep (n = 6) were kept in local animal housing facilities after the procedure. At 7, 14, 21, 28, and 60 days a hemodynamic valve assessment was performed by transthoracic echocardiography under general anaesthesia. Hemodynamic assessment during surgery and at 90 days was performed using sterile epi-cardiac echocardiography (ECE). All chronic study animals were sacrificed at 90 days.
Echocardiography
Under general anaesthesia, transthoracic 2D echocardiographic standard views were obtained at 7, 14, 21, 28 and 60 days postoperatively. Doppler flow data were acquired from the left ventricular outflow tract (LVOT) region in the pulsed wave mode, and from the aortic valve in the continuous wave mode in a five-chamber view. The modified Bernoulli equation was used to calculate peak and mean pressure gradients (MPG) across the prosthetic valve (Citation12). Continuity equation was used to calculate aortic valve area (AVA). Aortic regurgitation (AR) was evaluated and graded according to the current guidelines (AR: 0 – none, 1 – trace, 2 – mild, 3 – moderate and 4 – severe) (Citation13). At time of surgery and at 90 days follow-up, epi-cardiac echocardiography was performed, using a sterile covering over the transducer probe. Doppler assessment was then performed as described above.
Statistical analysis
Descriptive statistics are summarized for categorical variables as frequencies (%). Continuous variables were reported as mean ± standard deviation (SD). Data were analyzed by repeated measure analysis of variance (ANOVA). For data without repeated measurements a two-tailored Student´s t-test or Mann-Whitney U-test was applied as appropriate; p-values less than 0.05 were considered significant. All statistical analyses were performed using SPSS®, version 19.0 (IBM Corp., Armonk, NY, USA).
Results
All acute pig procedures were performed at UHE. The six chronic sheep experiments were performed at IMM. All valve implantations (n = 12) were performed off-pump as planned and all animals survived the implantation procedure. Induction and maintenance of anaesthesia was uneventful. All systemic hemodynamic parameters were stable throughout the entire observation time. Surgical exposure and preparation of the left apex took an average of 15.3 ± 4.2 min. No difficulties were experienced during preparation of the left ventricular apex. Despite all valve implantations being performed without rapid pacing, no proximal or distal valve embolization occurred. Fluoroscopic and angiographic guidance was used as the fundamental visualization method during implantation. Bleeding from the apex during implantation was easily managed by tightening the apical U-stitches, since no introducer sheath was used for valve delivery. After implantation, the final assessment of each valve confirmed a stable position of the prosthesis.
Acute pig implantations
The mean body weight of the swine (n = 6) prior to intervention was 62.6 ± 5.7 kg (range 57–71 kg). Mean aortic valve annular diameter, as measured by TEE, was 24.1 ± 1.0 mm (range 23–25 mm). As a result, all pigs received an M-size prosthesis. The final angiographic examination showed neither signs of AR nor any signs of coronary obstruction by the stent frame itself. Simultaneous TEE-evaluation, showed no significant AR post deployment, with good left-ventricular function throughout the experiments and until final sacrifice. MPG and AVA after valve deployments were 3.4 ± 2.1 mmHg and 2.4 ± 0.4 cm2, respectively. shows all intraoperative TEE results. All animals survived the implantation procedure and were sacrificed 30 min. after implantation. The final post-mortem analysis at necropsy revealed a firm and stable fixation of the valve stent and most importantly, the valve stent was orientated in an anatomically correct position within the aortic root, covering the leaflets of the native valve without coronary artery obstruction (). Valve competency was additionally tested by fluid application, which indicated no macroscopic signs of regurgitation.
Table I. Intraoperative echocardiographic (TEE) results (acute pig arm).
Chronic sheep implantations
The mean body weight of the sheep (n = 6) at the time of the surgical procedure was 43.5 ± 5.5 kg (range 36–52 kg). Aortic annulus diameters were measured at 22.1 ± 0.2 mm (range 19–24 mm). Four sheep received an M-size valve, and two sheep received an S-size valve. Valve deployment was uneventful and initial angiographic examination showed no signs of coronary obstruction by the stent frame. The post-mortem analysis at necropsy revealed a firm and stable fixation of the valve stent, and in concordance with the acute pig experiments, the valve stent was well orientated in an anatomically correct position. There were no cases of structural or non-structural valvular deterioration at 90 days.
Hemodynamic valve performance and follow-up
Prosthesis function and hemodynamic performance of all chronic sheep (n = 6) were assessed intraoperatively and at 7, 14, 21, 28, and 60 days follow-up. Intraoperatively and at 90 days follow-up, MPG and AVA as measured by ECE were 5.4 ± 2.2 mmHg and 1.8 ± 0.2 cm² and 4.0 ± 2.4 mmHg and 2.2 ± 0.4 cm², respectively. Echocardiographic data (MPG and AVA) at 7, 14, 21, 28, and 60 days are shown in . Intraoperative and 90 day MPG and AVA differed significantly from other follow-up measurements (P = 0.002). Intraoperatively, all animals showed either no or trace AR. During follow-up, mild to moderate paravalvular AR was noted in up to 50% of the sheep. Significant (Grade 3) paravalvular AR post-TAVI was infrequent, and was only observed in 1 sheep at 7 days and in 2 sheep at 60 days. Importantly, no severe paravalvular AR was observed during the study period. shows incidence of paravalvular AR at each time-point during follow-up. No signs of mitral valve impairment caused by the implanted prostheses were observed.
Discussion
Although transcatheter aortic valve implantation techniques are currently used to treat elderly and/or high risk patients with aortic stenosis (Citation5,Citation6), there is a substantial lack of experimental/animal studies evaluating new valves (Citation7). All models currently on the market share some important issues, such as positioning- and deployment-related complications (Citation4). The aim of the present experimental study was therefore to evaluate the safety, feasibility and ease of implantation, as well as the hemodynamic performance of an innovative self-expandable transapical transcatheter aortic valve prosthesis (ACURATE TA™) in an acute and chronic animal setup. This valve prosthesis, based on developments by Dr. von Segesser, aims to provide intra-annular fixation, self-centering and exact auto-positioning capabilities (Citation8). In addition, this stent valve is designed to offer the possibility of self-expansion, without the need for rapid pacing during implantation, and the possibility of partial repositioning (Citation8).
We initially investigated the feasibility, safety and ease of implantation of the ACURATE TA™ transcatheter aortic valve substitute in acute transcatheter aortic valve implantations in six pigs, evaluating only acute hemodynamics and valve performance. We additionally performed another six valve implantations in sheep, followed by a comprehensive follow-up period of 90 days. Pigs were chosen for the acute experiment due to the close similarity of their cardiac anatomy to that of humans (Citation9), while sheep were used in the chronic arm due to their calmer temperaments enabling them to be handled more easily. Moreover, sheep were selected for the chronic arm, since sheep represent a well-recognized model for chronic valve evaluation due to moderate weight and size increase during follow-up, so risk of paravalvular leak due to mismatch is limited.
The animal model used in the present study aims to mimic human anatomy, however it differs from standard clinical practice in several respects. Most notably, there is lack of pathology; the aortic annulus is elastic and the native leaflets are not calcified. Valve deployment is therefore different from that in the heavily calcified native human aortic valve. In this study, all valve prostheses were shown to be adequately anchored within the native aortic annulus without any dislocation or mal-positioning. This is largely owing to the self-expanding capabilities of the ACURATE TA™ prosthesis, and to the fact that the upper and lower crown of the stent enable intra-annular fixation by clamping the native annulus in between. The presumed advantages of the ACURATE TA™ prosthesis, of precise positioning and deployment in an anatomically correct site, were therefore verified by the present study since the device offers direct tactile feedback when pulling the device on the native annulus toward the left ventricle. Although there are no scientific data to back up the claim, surgeons perform commissural alignment in order to diminish turbulent flow through the valve and to ensure less leak with a better fit. It is very simple to align the ACURATE TA™ device with the native valve leaflets, it takes just a moment to do so, and could potentially ensure a better result with fewer leaks or flow interference which has to be evaluated in future evaluations. The ACURATE TA™ prosthesis is delivered through the apex of the left ventricle without the need for a sheath, which is generally used in other transapical TAVI-devices. On the one hand, the sheathless concept comes with the advantage of getting future devices even smaller to further reduce apical trauma and to result in a fast straight-forward procedure. On the other hand, the sheathless concept might result in an increased bleeding during the implantation period and in the case of necessary post-dilatation, a sheath must be inserted again. Additionally, we did not require rapid pacing during valve implantation, which is usually necessary in humans in case of balloon-expandable aortic valve implantation to ensure stable conditions during valve deployment. Balloon aortic valvuloplasty was also spared during this study, due to the non-calcified nature of the aortic valve of the study animals. Avoidance of both, rapid pacing and pre-dilatation might be useful in humans and has been reported by Grube et al. recently (Citation10). If avoidance of rapid pacing or pre-dilatation will also be offered by the present ACURATE TA™ device has to be evaluated in clinical human application.
Another important issue relates to the distances between the coronary ostia and the aortic annulus. Since the distances are shorter both in pigs and sheep compared to humans, it might be hypothetically possible, to observe a higher incidence of coronary ostia occlusion with transcatheter aortic valve implantation in an experimental animal setting. This might be true for several TAVI devices in this special setting, however in this study we did not observe any signs of coronary ostia occlusion at necropsy. This may be due to the stent design of the ACURATE TA™ prosthesis, which is intended to optimize anatomically correct positioning within the aortic annulus. Moreover, due to the possibility of anatomical correct implantation, the strut and commissural alignment might cause less influence on both coronary ostia.
We observed excellent hemodynamic parameters both in the acute and chronic study arms, despite a higher incidence of paravalvular leakage, which will be discussed below. In the acute experiments, we observed an MPG of 3.4 ± 2.1 mmHg and a calculated AVA of 2.4 ± 0.4 cm². After necropsy, no signs of prosthesis dislocation or coronary ostia occlusion were observed. Furthermore, all six prostheses implanted in the acute study arm were implanted in an anatomically correct position, as intended. These hemodynamic results of the acute experiments were replicated in the chronic sheep experimental study arm. In the sheep arm, approximate MPG values of around 1.3–1.8 mmHg and AVA values of around 3.0 cm² were obtained, which differed significantly intraoperatively and at 90 days follow-up. This can be explained by the two different methods of obtaining echocardiographic measurements, as the measurements were obtained by ECE intraoperatively and at 90 days, and by transthoracic echo at the other follow-up points. Although at these two-time points hemodynamic performance differed significantly with higher MPG and lower AVA, all observed values are still optimal with MPG around 5 mmHg and AVA around 2 cm². These echocardiographic findings indicate good results after ACURATE TA™ valve implantation, however final and long-term clinical application in humans is needed in order to verify these results.
At present, the ACURATE TA™ device is only available for implantation via the transapical approach, and has obtained CE Mark approval. Rigorous testing in animal models has been crucial in establishing the safety and effectiveness of new medical devices, and establishing a foundation for trials in human subjects. We are aware that reporting the results of an animal study after human results have already been published may undermine the usefulness of this paper, but we feel that it is important nevertheless due to the role of this work and similar studies in enabling the prosthesis to gain CE mark approval. A transfemoral version of this device is currently under clinical investigation with results expected in the future. A clinical study of the first 40 patients treated with this new transapical bioprosthesis has recently been published (Citation11). All 40 implants were delivered and implanted off-pump successfully in the desired position, however in one patient conversion to conventional surgery was necessary due to coronary occlusion. A balloon-post-dilatation was performed in 45% of patients, which was associated with a lower incidence of paravalvular aortic regurgitation (Citation11). In our present study some animals (2 mild at 90 days) showed signs of paravalvular aortic regurgitation (which was generally mild or moderate) during follow-up. We did not observe any signs of severe aortic regurgitation. This is due in part to the fact that all the animals had normal, uncalcified aortic valves, meaning that postdilatation would be unlikely to decrease their likelihood of developing paravalvular AR. In humans, the ACURATE TA™ prosthesis requires calcium for anchoring as both crowns capture the native leaflets and annular calcium within the waist of the device thereby pulling down the calcium into the annular plane. On the other hand, the compressed calcium may result in paravalvular leakage as well since the valve stent might be unable to expand completely. Additionally, the PET skirt acts as a “seal” prohibiting leak (Citation11). In the present study, although all valves have been implanted in “healthy” animals, emerging paravalvular aortic regurgitation (after several weeks) has been observed. This might be related to animal growth, resulting in an increased aortic annulus diameter as already mentioned by Bonhoeffer et al. (Citation14). Moreover, the given fact that in healthy animals no aortic annulus is clearly identifiable, might generally explain paravalvular leakage in an animal setup. This leads also to another potential point in a “healthy” animal study, possibly explaining paravalvular leakage even by the use of a PET-skirt valve, namely that the stent has to deal with a more “flexible” tissue throughout the complete cardiac cycle. In a previously published study, even in the low-pressure system of the right ventricle and pulmonary artery, paravalvular leakage after pulmonic valve implantation occurred (Citation15). Therefore, within the high-pressure environment of the left circulatory system, paravalvular leakage might be even more likely.
Limitations
The present study is limited due to the small study numbers, with only 12 animal TAVI procedures in total. In addition, the experimental setup did not reflect the real-world clinical practice, since all animals had normal aortic valves without any signs of calcification.
Conclusion
In this study, feasibility, safety, efficacy and hemodynamic performance of the ACURATE™ were demonstrated. We established that the ACURATE TA™ has an effective means of fixation, with precise positioning and anatomically correct orientation. We also demonstrated excellent hemodynamic results (gradients and aortic valve area) for this new device, both immediately after implantation and during a follow-up period of 90 days with signs of paravalvular leak related to the animal model. Further work needs to be done to evaluate this new valve in larger multicentre human studies with long-term follow-up.
Disclosures
Philipp Kahlert and Matthias Thielmann are clinical proctors for Edwards Lifesciences Inc. and have received honoraria payments. Stéphane Delaloye is an employee of the manufacturer Symetis SA and Luc Behr and Nicolas Borenstein are employees of the IMM Recherche Institut Mutualiste Montsouris and performed a contract work.
Declaration of interest: The authors report no conflicts of interest. The authors alone are responsible for the content and writing of the paper.
This work was funded by Symetis SA, Ecublens, Switzerland.
References
- Herrmann HC. Transcatheter Aortic Valve Implantation. Circ Cardiovasc Intervent 2008;1:159–60.
- Webb J, Cribier A. Percutaneous transarterial aortic valve implantation: what do we know? Eur Heart J 2011;32:140–7.
- Kempfert J, Rastan A, Holzhey D, Linke A, Schuler G, van Linden A, et al. Transapical aortic valve implantation: analysis of risk factors and learning experience in 299 patients. Circulation 2011;124:S124–9.
- Thielmann M, Kahlert P, Konorza T, Erbel R, Jakob H, Wendt D. Current developments in transcatheter aortic valve implantation techniques. Herz 2011;36:696–705.
- Leon MB, Smith CR, Mack M, Miller DC, Moses JW, Svensson LG, et al. Transcatheter Aortic-Valve Implantation for Aortic Stenosis in Patients Who Cannot Undergo Surgery. N Eng J Med 2010;363:1597–607.
- Smith CR, Leon MB, Mack MJ, Miller DC, Moses JW, Svensson LG, et al. Transcatheter versus Surgical Aortic-Valve Replacement in High-Risk Patients. N Eng J Med 2011;364:2187–98.
- Walther T, Dewey T, Wimmer-Greinecker G, Doss M, Hambrecht R, Schuler G, et al. Transapical approach for sutureless stent-fixed aortic valve implantation: experimental results. Eur J Cardiothoracic Surg 2006;29:703–8.
- Vergnat M, Henaine R, Kalejs M, Bommeli S, Ferrari E, Obadia JF, et al. A new self-expanding aortic stent valve with annular fixation: in vitro haemodynamic assessment. Eur J Cardiothoracic Surg 2009;35:970–5.
- Berreklouw E, Koene B, De Somer F, Bouchez S, Chiers K, Taeymans Y, et al. Sutureless replacement of aortic valves with St Jude Medical mechanical valve prostheses and Nitinol attachment rings: Feasibility in long-term (90-day) pig experiments. J Thorac Cardiovasc Surg 2011;141:1231–7.
- Grube E, Naber C, Abizaid A, Souza E, Mendiz O, Lemos P, et al. Feasibility of Transcatheter Aortic Valve Implantation Without Balloon Pre-Dilation: A Pilot Study. JACC: Cardiovascular Interventions 2011;4:751–7.
- Kempfert J, Rastan AJ, Beyersdorf F, Schönburg M, Schuler G, Sorg S, et al. Trans-apical aortic valve implantation using a new self-expandable bioprosthesis: initial outcomes. Eur J Cardiothorac Surg 2011;40:1114–9.
- Baumgartner H, Hung J, Bermejo J, Chambers JB, Evangelista A, Griffin BP, et al. Echocardiographic assessment of valve stenosis: EAE/ASE recommendations for clinical practice. J Am Soc Echocardiogr 2009;22:1–23.
- Zamorano JL, Badano LP, Bruce C, Chan KL, Gonçalves A, Hahn RT, et al.; Document Reviewers: European Association of Echocardiography (EAE): American Society of Echocardiography (ASE): The ASE Guidelines and Standards Committee. and the ASE Board of Directors. EAE/ASE recommendations for the use of echocardiography in new transcatheter interventions for valvular heart disease. Eur J Echocardiogr 2011;12:557–84.
- Boudjemline Y, Laborde F, Pineau E, Mollet A, Abadir S, Borenstein N, et al. Expandable right ventricular-to-pulmonary artery conduit: an animal study. Pediatr Res. 2006; 59:773–7.
- Boudjemline Y, Agnoletti G, Bonnet D, Behr L, Borenstein N, Sidi D, et al. Steps toward the percutaneous replacement of atrioventricular valves an experimental study. J Am Coll Cardiol 2005;46:360–5.