Abstract
The synthesis and biological evaluation of 3,4,5-trimethoxyphenyl acrylamides 1a–f as novel antinarcotic agents are described. The molecules were prepared by the Wittig reaction, followed by a coupling reaction between 3,4,5-trimethoxycinnamic acid (9) and aliphatic amines, which resulted in good yields. When tested for biological activity, compounds 1d–f exhibited strong inhibitory effects on the morphine withdrawal syndrome in mice due to their high binding affinities with serotonergic 5-HT1A receptors.
Introduction
Derivatives of cinnamic acid are common in plants. Methoxycinnamic acid and its derivatives have been shown to have interesting and important biological properties including analgesicCitation1, anti-arthritisCitation2, anti-inflammatoryCitation3, antipyreticCitation4, antibacterialCitation5, antiviralCitation6, and anti-cancerCitation7 effects. They are also useful key intermediates for many industrial products such as dyesCitation8 and liquid crystalsCitation9. Hydroxylated (p-coumaric acid, 3) or methoxylated (ferulic acid, 4 and sinapic acid, 5) derivatives of methoxycinnamic acid have been used as antioxidant agents, as well as inhibitors of monocarboxylate transport across plasma membranesCitation10. Recently, analogs of 3,4,5-trimethoxycinnamic acids were found to have antidepressant-like effects as well as sedative activity through suppression of norepinephrine in the locus coeruleusCitation11. A number of structurally diverse glucosidase inhibitors have exhibited potent anti- inflammatory and antiviral efficacy with little toxicity or few side effects in animal models ()Citation12. The Hashimoto groupCitation13 described the novel photoreactive cinnamic acid and its derivatives to elucidate phenylalanine ammonia-lyase. The Keung groupCitation14 demonstrated that aromatic-substituted cinnamic acid and its structural analogs inhibit monoamine oxidase A and exhibit antidepressant-like activity in mice. The Geraci groupCitation15 described the synthesis of hydroxycinnamic acid clustered by a calixarene and evaluated its radical scavenging and antioxidant activity by using 2,2′-diphenyl-1-picrylhydrazyl (DPPH)-induced radicals and the azo-bis-isobutyrylnitrile (AIBN)-induced linoleic acid peroxidation test. Recently, 3,4,5-trimethoxycinnamic acid (TMCA) was identified as a component of Onji, a sedative treatment used in Japanese traditional Kampo medicine. The Kawashima groupCitation16 demonstrated that TMCA induces corticotropin-releasing hormone (CRH) and that TMCA has a sedative effect by suppressing norepinephrine (NE) content in the locus coeruleus (LC). Hyperactivity of LC neurons is an important symptom of opiate withdrawal. Opiate withdrawal syndrome is mediated by an excitatory amino acid input to the LC that causes hyperactivity in the LC regionCitation17. During naloxone-induced jumping behavior, there is a significant increase in extracellular levels of NECitation18. These studies suggest that TMCA may be active in opioid withdrawal by suppressing NE or by blocking excitatory amino acid input to the LC. We have synthesized 3,4,5-trimethoxyphenyl acrylamides, and determined their effect on morphine dependence in mice and their binding affinities on serotonergic receptors. We report herein the short synthesis protocol for novel 3,4,5-trimethoxyphenyl acrylamides 1a–f, which we synthesized in high yields starting from commercially available 3,4,5-trihydroxybenzaldehyde (7) via Wittig and coupling reactions.
Materials and methods
General procedures
All other commercial reagents and solvents were used as received without further purification. Reaction solvents were distilled from calcium hydride for dichloromethane and from sodium metal and benzophenone for tetrahydrofuran. The reactions were monitored and the Rf (retention factor) values determined using analytical thin layer chromatography (TLC) with Merck silica gel 60, F-254 precoated plates (0. 25 mm thickness). Spots on the TLC plates were visualized using ultraviolet light (254 nm) and a cerium sulfate/ammonium dimolybdate/sulfuric acid solution, followed by heating on a hotplate. Flash column chromatography was performed with Merck silica gel 60 (230–400 mesh) to purify the reaction mixture which was recrystallized by diethyl ether in dichloromethane. 1H nuclear magnetic resonance (NMR) and 13C NMR spectra were recorded on a Bruker DRX 250 spectrometer at 250 MHz and 63 MHz, or a DRX 400 at 400 MHz and 100 MHz, respectively. Proton chemical shifts are reported in ppm (δ) relative to internal tetramethylsilane (TMS; δ 0.00 ppm) or with the solvent reference relative to TMS employed as the internal standard (CDCl3, δ 7.26 ppm). Data are reported as follows: chemical shift {multiplicity [singlet (s), doublet (d), triplet (t), quartet (q), and multiplet (m)], coupling constants [Hz], integration}. Carbon chemical shifts are reported in ppm (δ) relative to TMS with the respective solvent resonance as the internal standard (CDCl3, 77.16 ppm). Infrared (IR) spectra were recorded on a Jasco FT/IR-430 spectrometer. Data are reported in wave numbers (cm−1). Electrospray ionization-liquid chromatography- mass spectrometry (ESI-LC-MS) was done on a Waters ZQ 4000 LC-MS spectrometer. Melting points were determined on a Bibby Stuart Scientific melting point appratus SMP3. Elemental analyses were performed on a CE instrument EA1110.
General procedure for the preparation of amides 1a–f
To a stirred solution of 3,4,5-trimethoxycinnamic acid (9, 1.0 g, 4.2 mmol) in dry dichloromethane (30 mL) was added 1-ethyl(dimethylaminopropyl)carbodiimide (EDCI, 0.97 g, 5.0 mmol), 1-hydroxybenzotriazole (HOBt, 0.68 g, 5.0 mmol), and triethylamine (TEA, 0.70 mL, 5.0 mmol), and then the reaction mixture was stirred at room temperature for 30 min. The several amines were then added to the reaction mixture and the resulting mixture was stirred at room temperature for 24 h. The reaction mixture was washed with brine (20 mL) and water (20 mL). The organic layer was separated, dried over anhydrous MgSO4, and filtered, and the solvent was removed under reduced pressure. The residue was purified by flash column chromatography (silica gel, ethyl acetate/hexanes = 2:1, v/v).
(E)-N-Methyl-3-(3,4,5-trimethoxyphenyl) acrylamide (1a) Yield: 81%, white solid. mp 123–124°C; Rf = 0.2 (ethyl acetate/hexanes = 3:1, v/v); IR νmax (CHCl3, KBr) 3430 2936 2343 1659 1622 1556 1506 1422 1325 1285 1126 cm−1; 1H NMR (250 MHz, CDCl3) δ 7.53 (d, 1H, J = 15.6), 6.70 (s, 2H), 6.62 (s, 1H), 6.46 (d, 1H, J = 15.6), 3.85 (s, 6H), 3.82 (s, 3H), 2.94 (d, 3H, J = 4.7); 13C NMR (63 MHz, CDCl3) δ 166.8 153.3 140.3 139.3 130.5 120.3 104.8 60.9 56.0 26.5; LC-MS (ESI+) m/z 274.02 [M + Na].
(E)-N-Allyl-3-(3,4,5-trimethoxyphenyl) acrylamide (1b) Yield: 81%, white solid. mp 148–149°C; Rf = 0.4 (ethyl acetate/hexanes = 2:1, v/v); IR νmax (CHCl3, KBr) 3439 1634 1124 cm−1; 1H NMR (250 MHz, CDCl3) δ 7.56 (d, 1H, J = 15.6), 6.72 (s, 2H), 6.42 (d, 1H, J = 15.5), 6.19 (t, 1H), 5.82–5.97 (m, 1H), 5.24 (dd, 1H, J = 1.4, 17.2), 5.16 (dd, 1H, J = 1.3, 10.2), 4.02 (t, 2H), 3.86 (s, 6H), 3.85 (s, 3H); 13C NMR (63 MHz, CDCl3) δ 165.9 153.4 141.1 139.5 134.2 130.5 120.1 116.6 104.9 61.0 56.1 42.2; LC-MS (ESI+) m/z 300.12 [M + Na].
(E)-N-Butyl-3-(3,4,5-trimethoxyphenyl) acrylamide (1c) Yield: 69%, white solid. mp 152–153°C; Rf = 0.3 (ethyl acetate/hexanes = 2:1, v/v); IR νmax (CHCl3, KBr) 3272 2958 2933 1659 1621 1583 1552 1505 1463 1417 1323 1279 1241 1212 1126 1004 cm−1; 1H NMR (250 MHz, CDCl3) δ 7.54 (d, 1H, J = 15.5), 6.67 (s, 2H), 6.36 (d, 1H, J = 15.5), 5.90 (t, 1H), 3.87 (s, 9H), 3.39 (q, 2H); 1.50–1.61 (m, 2H), 1.34–1.46 (m, 2H), 0.94 (t, 3H); 13C NMR (63 MHz, CDCl3) δ 165.9 153.6 140.9 139.8 130.6 120.4 105.1 61.1 56.3 39.6 31.9 20.3 13.9; LC-MS (ESI+) m/z 316.29 [M + Na].
(E)-N-Hexyl-3-(3,4,5-trimethoxyphenyl) acrylamide (1d) Yield: 67%, white solid. mp 127–128°C; Rf = 0.4 (ethyl acetate/hexanes = 2:1, v/v); IR νmax (CHCl3, KBr) 3286 2932 2853 1659 1620 1584 1556 1505 1463 1418 1324 1278 1127 1005 cm−1; 1H NMR (250 MHz, CDCl3) δ 7.54 (d, 1H, J = 15.5), 6.73 (s, 2H), 6.35 (d, 1H, J = 15.5), 5.82 (t, 1H), 3.78 (s, 9H); 3.38 (q, 2H), 1.51–1.59 (m, 2H), 1.31 (br s, 6H), 0.86 (br s, 3H); 13C NMR (63 MHz, CDCl3) δ 165.9 153.4 140.8 139.7 130.6 120.3 104.8 61.1 56.2 39.9 31.6 29.7 26.7 22.7 14.2; LC-MS (ESI+) m/z 344.35 [M + Na].
(E)-N-Octyl-3-(3,4,5-trimethoxyphenyl) acrylamide (1e) Yield: 86%, white solid. mp 119–120°C; Rf = 0.6 (ethyl acetate/hexanes = 2:1, v/v); IR νmax (CHCl3, KBr) 3291 2919 2852 1653 1619 1582 1454 1418 1384 1325 1282 1241 1128 cm−1; 1H NMR (250 MHz, CDCl3) δ 7.54 (d, 1H, J = 15.5), 6.72 (s, 2H), 6.34 (d, 1H, J = 15.5), 5.79 (t, 1H), 3.87 (s, 9H), 3.38 (q, 2H), 1.50–1.60 (m, 2H), 1.26 (br s, 10H), 0.87 (br s, 3H); 13C NMR (63 MHz, CDCl3) δ 165.9 153.4 140.9 139.3 130.6 120.2 104.7 61.1 56.1 39.9 31.9 29.8 29.4 29.3 27.1 22.8 14.2; LC-MS (ESI+) m/z 372.56 [M + Na]; Elemental analysis Calcd for C20H31NO4: C, 68.74; H, 8.94; N, 4.01%. Obsd: C, 68.87; H, 8.96; N, 4.17%.
(E)-N-Decyl-3-(3,4,5-trimethoxyphenyl) acrylamide (1f) Yield: 78%, white solid. mp 117–120°C; Rf = 0.7 (ethyl acetate/hexanes = 2:1, v/v); IR νmax (CHCl3, KBr) 3439 3276 2919 2849 1655 1615 1582 1541 1505 1454 1413 1321 1271 1239 1129 cm−1; 1H NMR (250 MHz, CDCl3) δ 7.53 (d, 1H, J = 15.5), 6.71 (s, 2H), 6.37 (d, 1H, J = 15.5), 6.00 (s, 1H), 3.86 (s, 6H), 3.85 (s, 3H), 3.38 (q, 2H), 1.56 (m, 2H), 1.23 (br s, 14H), 0.88 (t, 3H); 13C NMR (63 MHz, CDCl3) δ 165.9 153.4 140.7 139.4 130.6 120.4 104.9 61.0 56.1 39.9 32.0 31.8 29.8 29.6 29.4 29.4 27.1 26.7 22.7 14.2; LC-MS (ESI+) m/z 400.04 [M + Na]; Elemental analysis Calcd for C22H35NO4: C, 69.99; H, 9.34; N, 3.71%. Obsd: C, 69.90; H, 9.43; N, 3.78%.
Animals and drug administration
C57BL/6 mice were obtained from Daehan Biolink (Eumsung, Korea). They were housed in a 12 h light–dark cycle with temperature maintained at 24 ± 3°C. All animal procedures were in accordance with the Institutional Animal Care and Use Committee of Ewha Womans University. The mice (male, 20 ± 2 g) were randomly divided into 10 groups and were given saline, morphine, or both morphine and 3,4,5-trimethoxyphenyl acrylamides. Morphine chloride (10 mg/kg/day; Myungmun Pharm., Seoul) was dissolved in saline, and 3,4,5-trimethoxyphenyl acrylamides (5 mg/kg/day) were dissolved in 10% Cremophor solution containing 2% dimethyl sulfoxide. Morphine and 3,4,5- trimethoxyphenyl acrylamides were administered daily for 7 days intraperitoneally. 3,4,5-Trimethoxyphenyl acrylamides were administered 30 min prior to the injection of morphine. Naloxone hydrochloride (5 mg/kg, i.p.) was injected 6 h after the final morphine injection for the induction of morphine withdrawal syndrome in mice.
Measurement of morphine withdrawal syndrome
Morphine withdrawal syndrome was induced by the injection of naloxone (5 mg/kg), which is a competitive antagonist with high opioid receptor affinity. Immediately after the naloxone injection, mice were placed into individual observation cylinders ( 24 cm in diameter and 50 cm in height), and the frequency of jumps of each mouse was observed for 30 min.
[3H]8-OH-DPAT binding to serotonin 5-HT1A receptor
Membranes from a stable CHO-K1 cell line expressing the human recombinant 5-HT1A serotonin receptor (PerkinElmer Life and Analytical Sciences, MA, USA) were used. For the binding assay, aliquots of receptor membranes, 0.25 nM [3H]8-OH-DPAT (8-hydroxy-2-(di-N-propylamino)tetralin, 154.3 Ci/mM; New England Nuclear, Boston, MA) and appropriate concentrations of test compounds were added to 0.25 mL of 50 mM Tris-HCl (pH 7.4) buffer containing 10 mM MgSO4, 0.5 mM ethylenediaminetetraacetic acid (EDTA), and 0.1% ascorbic acid. Incubations were carried out for 60 min at 27°C, and these were terminated by rapid filtration using a MicroBeta FilterMate-96 harvester (PerkinElmer) through a Filtermat A glass fiber filter presoaked in 0.3% polyethylenimine (PEI). The filter was washed with ice-cold 50 mM Tris-HCl buffer solution (pH 7.4), and was then covered with MeltiLex, sealed in a sample bag, and dried in an oven. The radioactivity retained in the filter was finally counted using MicroBeta Plus (Wallac, Finland). Nonspecific binding was defined with 0.5 μM of methiothepin. The binding affinity (IC50) of a compound was calculated by computerized nonlinear regression analysis (GraphPad Prism Software; GraphPad, San Diego, USA) using 7–8 varied concentrations of the compound run in duplicate tubes.
[3H]Ketanserin binding to serotonin 5-HT2A receptor
For serotonin 5-HT2A binding, an aliquot of frozen membrane from a CHO-K1 cell line expressing the human recombinant 5-HT2A receptor (PerkinElmer) and [3H]ketanserin (1 nM; PerkinElmer) were used in the presence of mianserin (20 μM) as nonspecific. The reaction mixture was incubated for 60 min at 27°C using 50 mM Tris-HCl (pH 7.4) buffer containin 4 mM CaCl2 and 0.1% ascorbic acid, and harvested through a Filtermat A glass fiber filter presoaked in 0.5% PEI.
[3H]Mesulergine binding to serotonin 5-HT2C receptor
Frozen membranes from a stable CHO-K1 cell line expressing the human recombinant 5-HT2C receptor (PerkinElmer) were used. For the binding assay, [3H]mesulergine (1 nM; Amersham Biosciences, Buckinghamshire, UK), receptor membrane, and test compounds were added into 50 mM Tris-HCl (pH 7.4) buffer containing 0.1% ascorbic acid and 4 mM CaCl2. Nonspecific binding was determined using 100 μM mianserin. The incubations were performed for 60 min at 27°C, and these were terminated by rapid filtration through a Filtermat A glass fiber filter presoaked in 0.5% PEI.
[3H]LSD binding to serotonin 5-HT6 and 5-HT7 receptors
For receptor binding assays, human 5-HT6 and 5-HT7 serotonin receptors expressed in CHO-K1 cells (PerkinElmer) were used. For 5-HT6 receptor binding, frozen membrane, 1 nM [3H]LSD (lysergic acid diethylamide; PerkinElmer), and appropriate concentrations of test compounds were added to 0.25 mL of 50 mM Tris-HCl (pH 7.4) buffer containing 0.1% ascorbic acid and 4 mM CaCl2. Nonspecific binding was determined using 10 μM methiothepin. The incubations were performed for 60 min at 27°C, and these were terminated by rapid filtration through a Filtermat A glass fiber filter presoaked in 0.5% PEI. For the 5-HT7 receptor binding assay, cell membrane, 3 nM [3H]LSD, and appropriate concentrations of test compounds were added to 0.25 mL of 50 mM Tris-HCl (pH 7.4) buffer containing 10 mM MgSO4 and 0.5 mM EDTA. The mixture was incubated for 120 min at 27°C, and the reaction was terminated by rapid filtration through a Filtermat A glass fiber filter presoaked in 0.3% PEI. Nonspecific binding was determined using 10 μM methiothepin.
[3H]Imipramine binding to serotonin transporter
For serotonin transporter binding studies, frozen membrane from a stable HEK-293 cell line expressing the human recombinant 5-HT transporter (PerkinElmer), 3 nM [3H]imipramine (PerkinElmer), and appropriate concentrations of test compounds were added to 0.25 mL of 50 mM Tris-HCl (pH 7.4) buffer containing 120 mM NaCl and 5 mM KCl. Nonspecific binding was determined using 200 μM imipramine. The incubations were performed for 30 min at 27°C, and these were terminated by rapid filtration through a Filtermat A glass fiber filter presoaked in 0.5% PEI.
Primary cortical culture
Cortical cell culture was prepared from embryos of C57BL/6 mice at the gestational age of 15 days. The brain was dissected and kept in an ice-cold solution. The cortical tissues were dissociated to single cells by a gentle suspension. The cell suspension was centrifuged at 1000 rpm for 5 min, and the resulting pellets were suspended in minimum essential medium (MEM; Gibco-BRL, Rockville, MD, USA) supplemented with 5% heat-inactivated fetal calf serum and house serum (Gibco) and dispensed on a plate coated with poly-d-lysine (Sigma, St. Louis, MO, USA). The cells were cultured in a CO2 incubator (5% (v/v), 37°C). Seven days after plating, the cells were treated with 10 μM cytosine arabinofuranoside (Ara C) to reduce the growth of contaminating non-neuronal cells. The cortical neuronal cells were cultured in MEM supplemented with 10% horse serum, but without glutamine.
Western immunoblot analysis
The 3,4,5-trimethoxyphenyl acrylamides (10 μM) were treated with or without WAY 100635 (1 μM), a selective antagonist of 5-HT1A receptor, for 30 min in cortical neuronal cells. The cortical neuronal cell samples were extracted with lysis buffer (150mM NaCl, 50 mM Tris-HCl pH 8, 1 mM EDTA, 1% Triton-X 100, 1 mM PMSF (phenylmethylsulfonyl fluoride), 1 mM Na3VO4) and centrifuged at 13,500 rpm for 15 min at 4°C. Protein concentrations were determined using a protein assay kit (Pierce Biotechnology). Sample buffer (× 4) was added to extract and boiled for 4 min at 100°C. Equal amounts of cellular protein were separated by 10% polyacrylamide gels and were electrophoretically transferred to polyvinylidene fluoride (PVDF) membranes. Transfer blots were blocked in 3% skim milk in TBST (Tris-buffered saline–Tween 20) 0.1% for 1 h and incubated with antibodies specific to pERK1/2 (1:1000; Santa Cruz Biotechnology, CA, USA) or GAPDH (glyceraldehyde 3-phosphate dehydrogenase, 1:1000; Cell Signaling), at 4°C overnight. Blots were washed three times for 30 min in 0.1% TBST, and incubated with an appropriate horseradish peroxidase-conjugated secondary antibody (Zymed Laboratiories). Bound antibodies were visualized following chemiluminescence detection (Amersham Pharmacia Biotechnology, CA, USA).
Statistical analysis
All values are expressed as mean ± standard error (SE). The results were subjected to analysis of variance (ANOVA) using the Newman–Keuls multiple comparison test.
Results and discussion
Synthesis of 3,4,5-trimethoxyphenyl acrylamides
The synthesis of 3,4,5-trimethoxyphenyl acrylamides is outlined in . 3,4,5-Trimethoxy benzaldehyde (8) was prepared by modification of a previously reported procedureCitation19, where commercially available 3,4,5-trihydroxy benzaldehyde (7) was treated with dimethylsulfate in the presence of KOH to generate aldehyde 8 in 60% yield. Compound 8 was produced by the Wittig reaction by using freshly prepared Wittig salts (triphenyl phosphine and ethyl 2-bromoacetate in toluene) and KOH in dimethylsulfoxide (DMSO) to give 3,4,5-trimethoxycinnamic acid (TMCA) (9) in 52% yieldCitation20. Acid 9 was coupled with various amines (methylamine, allyl amine, n-butyl amine, n-hexyl amine, n-octyl amine, n-decyl amine) in the presence of 1-ethyl(dimethylaminopropyl) carbodiimide (EDCI), 1-hydroxybenzotriazole (HOBt), and triethylamine (TEA) in dichloromethane to give 3,4,5-trimethoxyphenyl acrylamides 1a–f in 67% to 86% yields ().
Antinarcotic properties of 3,4,5-trimethoxyphenyl acrylamides
Mice received morphine (10 mg/kg/day, i.p.) for 7 days to develop a dependence. The effects of 3,4,5- trimethoxyphenyl acrylamides (5 mg/kg/day, i.p.) were examined on naloxone-induced jumping behavior in morphine-dependent mice. As shown in , the frequency of jumping, which is an indicator of morphine withdrawal symptoms, was decreased by treatment with 3,4,5-trimethoxyphenyl acrylamides. Interestingly, each of the 3,4,5-trimethoxyphenyl acrylamides were found to inhibit jumping frequency in morphine dependent mice: 1a (13%), 1b (27%), 1c (57%), 1d (78%), 1e (88%), and 1f (80%). Furthermore, compound 1e displayed the greatest degree of attenuation, and 5 mg/kg of this compound administered intraperitoneally is as effective as the 5-HT1A receptor agonist (+)8-OH-DPAT (0.5 mg/kg, i.p.).
Figure 2. Effects of 3,4,5-trimethoxyphenyl acrylamides on naloxone-induced jumping behavior in morphine-dependent mice. TMCA and 3,4,5-trimethoxyphenyl acrylamides (5 mg/kg, i.p.) and (+)8-OH-DPAT (0.5 mg/kg, i.p.) were injected 30 min before morphine injection (10 mg/kg, i.p.) for 7 days. On the 7th day, naloxone (5 mg/kg, i.p.) was injected 6 h after final drug administration. The number of jumps in a 30 min interval was counted after naloxone injection. *p < 0.05, **p < 0.01 in comparison with morphine only group.
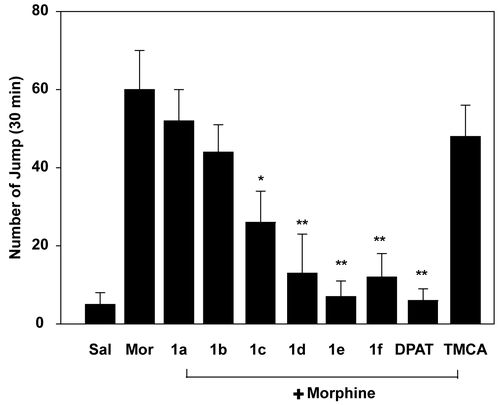
Receptor binding affinity analysis of 3,4,5-trimethoxyphenyl acrylamides
To elucidate the mechanistic basis for the antinarcotic effect of 3,4,5-trimethoxyphenyl acrylamides, their ability to bind several serotonergic receptors including 5-HT1A, 5-HT2A, 5-HT2C, 5-HT6, 5-HT7, and the 5-HT transporter was evaluated. Interestingly, all of the 3,4,5-trimethoxyphenyl acrylamides showed a high binding affinity to the 5-HT1A receptor. Similarly, the basal form of 3,4,5-trimethoxycinnamic acid (TMCA) also had a high affinity for the 5-HT1A receptor (). Among the six 3,4,5-trimethoxyphenyl acrylamides 1a–f, compound 1e showed the highest binding affinity to the 5-HT1A receptor. Interestingly, compound 1f exhibited a significant binding affinity to several serotonergic receptors including 5-HT1A, 5-HT2A, 5-HT2C, 5-HT6, 5-HT7, and the 5-HT transporter.
Table 1. Serotonin (5-HT) receptor and transporter binding affinities of 3,4,5-trimethoxyphenyl acrylamides.
Effect of 5-HT1A receptor-specific antagonist on 3,4,5-trimethoxyphenyl acrylamide-induced pERK expression in cultured cortical neurons
The ability of all the newly synthesized 3,4,5- trimethoxyphenyl acrylamides to act as 5-HT1A receptor agonists was tested by monitoring the activation of ERK1/2 expression in the presence of WAY 100635, a selective 5-HT1A receptor antagonist. As shown in , when cortical neuronal cells were treated with 3,4,5-trimethoxyphenyl acrylamides 1a–f (10 μM) for 30 min, pERK expression was elevated. However, this induction of pERK was suppressed upon treatment with WAY 100635 (1 μM). Of the compounds tested, 1a and 1c were the most potent activators of pERK expression in the absence of WAY 100635.
Conclusions
The 3,4,5-trimethoxyphenyl acrylamides 1a–f were synthesized and tested for their antinarcotic properties in naloxone-induced morphine withdrawal syndrome in mice and receptor binding affinity on serotonergic receptors. Four of the six 3,4,5-trimethoxyphenyl acrylamide compounds tested, 1c–f, elicited significant inhibition of the morphine withdrawal syndrome in mice and showed strong binding affinities for serotonergic 5-HT1A receptors. Of these analogs, compound 1e exhibited the most potent inhibitory action against jumping, which is an indicator of morphine-induced withdrawal symptoms. These results suggest that 3,4,5-trimethoxyphenyl acrylamides could be useful for the development of antinarcotic agents.
Acknowledgments
This work was supported by Ewha Global Challenge (BK21), a KFDA grant (2008), and a Korean Research Foundation Grant funded by the Korean Government (MOEHRD) (KRF-2006-312-C00267), the Republic of Korea. Two of the authors (D.M. and Y.L.) appreciate the fellowship of the BK21 program from the Ministry of Education and Human Resources Development. The first two authors (J.-C.J. and D.M.) contributed equally to this work.
References
- Rennison D, Neal AP, Cami-Kobeci G, Aceto MD, Martinez-Bermejo F, Lewis JW, et al. Cinnamoyl derivatives of 7alpha-aminomethyl-6,14-endo-ethanotetrahydrothebaine and 7alpha-aminomethyl-6,14-endo-ethanotetrahydrooripavine and related opioid ligands. J Med Chem 2007;50:5176–82.
- Rodeschini V, Boiteau JG, Van de Weghe P, Tarnus C, Eustache J. MetAP-2 inhibitors based on the fumagillin structure. Side-chain modification and ring-substituted analogues. J Org Chem 2004;69:357–73.
- Chen L, Zhang Y, Kong X, Peng S, Tian J. Synthesis and biological evaluation of nitric oxide-releasing derivatives of oleanolic acid as inhibitors of HepG2 cell apoptosis. Bioorg Med Chem Lett 2007;17:2979–82.
- Eagle E, Carlson AJ. Toxicity, antipyretic and analgesic studies on 39 compounds including aspirin, phenacetin and 27 derivatives of carbazole and tetrahydrocarbazole. J Pharmacol Exp Ther 1950;99:450–7.
- Carvalho SA, da Silva EF, de Souza MVN, Lourenco MCS, Vicente FR. Synthesis and antimycobacterial evaluation of new trans-cinnamic acid hydrazide derivatives. Bioorg Med Chem Lett 2008;18:538–41.
- Hegazi AG, Farghaly AA, Abd El Hady FK. Egyptian propolis: 3. Antioxidant, antimicrobial activities and chemical composition of propolis from reclaimed lands. J Vet Sci 2004;38:11–15.
- Watanabe S, Sato S, Nagase S, Shimosato K, Saito T. Polyamine levels in various tissues of rats treated with 3-hydroxy-4-methoxycinnamic acid and 3,4-dimethoxycinnamic acid. Anti-Cancer Drugs 1996;7:866–72.
- Mishra S, Bisaria VS. Production and characterization of laccase from Cyathus bulleri and its use in decolourization of recalcitrant textile dyes. Appl Microbiol Biotechnol 2006;71:646–53.
- Rout DK, Choudhary RNP, Nigam GD. Optical and dielectric studies of azobenzene and p-methoxy cinnamic acid(p-MCA). Mol Cryst Liquid Cryst 1988;158B:151–62.
- Lee GS, Widjaja A, Ju YH. Enzymatic synthesis of cinnamic acid derivatives. Biotech Lett 2006;28:581–5.
- Kawashima K, Miyako D, Ishino Y, Makino T, Saito K, Kano Y. Anti-stress effects of 3,4,5-trimethoxycinnamic acid, an active constituent of roots of Polygala tenuifolia (Onji). Biol Pharm Bull 2004;27:1317–19.
- Adisakwattana S, Sookkongwaree K, Roengsumran S, Petsom A, Ngamrojnavanich N, Chavasiri W, et al. Structure–activity relationships of trans-cinnamic acid derivatives on α-glucosidase inhibition. Bioorg Med Chem Lett 2004;14:2893–6.
- Hashimoto M, Hatanaka Y, Nabeta K. Novel photoreactive cinnamic acid analogues to elucidate phenylalanine ammonia-lyase. Bioorg Med Chem Lett 2000;10:2481–3.
- Tao G, Irie Y, Li DJ, Keung WM. Eugenol and its structural analogs inhibit monoamine oxidase A and exhibit antidepressant-like activity. Bioorg Med Chem Lett 2005;13:4777–88.
- Consoli GML, Galante EG, Daquino C, Granata G, Cunsolo F, Geraci C. Hydroxycinnamic acid clustered by a calixarene platform: radical scavenging and antioxidant activity. Tetrahedron Lett 2006;47:6611–14.
- Kawashima K, Miyako D, Ishino Y, Makino T, Saito K, Kano Y. Anti-stress effects of 3,4,5-trimethoxycinnamic acid, an active constituent of roots of Polygala tenuifolia (Onji). Biol Pharm Bull 2004;27:1317–19.
- Akaoka H, Aston-Jones G. Indirect serotonergic agonists attenuate neuronal opiate withdrawal. Neuroscience 1993;54:561–5.
- Fuentealba JA, Forray M, Inés Gysling K. Chronic morphine treatment and withdrawal increase extracellular levels of norepinephrine in the rat bed nucleus of the stria terminalis. J Neurochem 2001;75:741–8.
- Beninton F, Morin RD, Clacke LC Jr. Synthesis of 4-hydroxy- and 4-ethoxy-3,5-dimethoxy-β-phenethylamines. J Am Chem Soc 1954;76:5555–6.
- Srivastava V, Darokar MP, Fatima A, Kumar JK, Chowdhury C, Saxena HO, et al. Synthesis of diverse analogues of Oenostacin and their antibacterial activities. Bioorg Med Chem 2007;15:518–25.