Abstract
Nitrofurazone (NF) and its derivative, hydroxymethylnitrofurazone (NFOH), have presented antichagasic activity. NFOH has higher activity and lower mutagenicity. The aim of this work was to assess whether NF and its derivative NFOH would also be inhibitors of cruzain, besides their trypanothione reductase inhibitory activity. In vitro cruzain inhibition tests were performed for both compounds, and the 50% inhibitory concentration (IC50) for NF and NFOH presented values of 22.83 ± 1.2 μM and 10.55 ± 0.81 μM, respectively. AM1 semi-empirical molecular modeling studies were performed to understand the activity of the compounds, corroborating the observed cruzain inhibitory activity.
Introduction
Chagas’ disease is an important social and medical challenge for people living in Latin America. Infection caused by the intracellular protozoan Trypanosoma cruzi, the causative agent of Chagas’ disease, persists for the lifetime of the human/mammalian host. This disease is endemic in 21 countries, mostly in Latin America, with more than 300,000 new cases every year and aboutCitation16–18 million infected peopleCitation1–. Current therapy is based on nifurtimox (NFX) and benznidazole (BNZ) (), which are mainly effective in the acute phase of the disease and may cause serious adverse side-effectsCitation3,Citation4. In Brazil, the only drug available in the market is BNZ, in spite of the claim that Brazilian T. cruzi strains are more resistant to this drug than strains from other countriesCitation4. Therefore, new and better drugs are urgently needed to face this serious situation.
Nitrofurazone (NF), a 5-nitro-2-furfurylidenesemicarbazone, has been known for a long time to exhibit antimicrobial activityCitation5. Initially, NF was used as an antimicrobial agent against gram-positive microorganisms only for topical infections due to its side-effectsCitation6. Nevertheless, its trypanomicidal activity was earlier demonstratedCitation7,Citation8. The mechanism of action of NF was found to be based on the inhibition of trypanothione reductase, an enzyme involved in the antioxidant system of the parasite, by means of reduction of the nitro groupCitation9.
More recently, a novel NF derivative, hydroxymethylnitrofurazone (NFOH), was shown to present better trypanomicide activity and lower mutagenicity potential than NFCitation10. More specifically, NF and NFOH were tested in cell culture (LLC-MK2) infected with amastigote and trypomastigote forms of the parasite, and NFOH (5 μM) presented 100% inhibitory activity against amastigotes, while NF (5 μM) showed 90% and BNZ (10 μM) 80%. In T. cruzi trypomastigotes, NFOH (5 μM) resulted in total inhibition, while NF (5 μM) and BNZD (10 μM) led to parasite recrudescence on the 15th day. These data indicate higher activity for NFOH in comparison to NF. It is also important to point out that NFOH proved to be less mutagenic than NF, according to mutagenicity tests carried out in S. typhimurium TA98 strain. These promising results raised the need for other studies in order to explain the reasons for the higher activity of NFOH.
One of the driving forces for an antichagasic development has been the selectivity of action, through the knowledge of biochemical targets in the parasiteCitation11. Cysteine proteases are relevant to some paths of the parasite’s life-cycle and also to the parasite–host relationship. They have been considered good targets in the design of new chemotherapeutic agents against parasites. T. cruzi’s primary cysteine protease, cruzain or cruzipain, is essential for the infection of host cells, replication, and metabolism throughout the life-cycle of the parasiteCitation12. This enzyme exhibits a three-dimensional structure similar to those of the papain family proteases such as cathepsin L, but the substrate specificity is reminiscent of cathepsin B, because the S2 subsite of cruzain accepts basic amino acids quite wellCitation13–15.
The search for new nonpeptidic cruzain inhibitors has been used for the development of antichagasic chemotherapyCitation16. Recently, Du and co-workersCitation17 have suggested nucleophilic attack by cruzain’s Cys-25 of the semicarbazone group of inhibitor compounds. This mechanism was also suggested by Aguirre and co-workers for compounds containing thiosemicarbazone or semicarbazone groupsCitation18. Considering that both NF and NFOH () present the semicarbazone chemical function, one can envision that a similar inhibition reaction could take place between cruzain and these molecules.
Therefore, there could be a dual mechanism of action: a reduction of the nitro group associated with cruzain inhibition. Thus, the possibility of cruzain inhibition by NF and NFOH is worth investigating.
Mainly since the 1980s, molecular modeling has been shown to be a good tool in drug design, due to advances in computational techniques and facilities, i.e. the development of better hardware and softwareCitation19. By means of theoretical and computational chemistry, molecular modeling studies provide ways to study the physicochemical characteristics of a molecule with the aim of investigating its quantitative structure–activity relationships (QSARs). In addition, it allows better understanding of the molecule’s mechanism of action through the construction and interpretation of 3D modelsCitation20. Molecular modeling can be based on classical mechanics, molecular mechanics, or quantum mechanics, and may employ semi-empirical or ab initio methods.
The Hamiltonian Austin Model 1 (AM1) method employed in this work is a semi-empirical method that has shown good predictions for nitro compounds such as the antischistosomal drug oxaminiquine and megazol analogs with anti-tripanosomal activityCitation21,Citation22.
In this work, we investigated the inhibitory effect of NF and NFOH on the enzyme cruzain, employing molecular modeling as a tool to elucidate the stereoelectronic characteristics governing this inhibition. In vitro enzyme inhibitory tests were also carried out for both NF and NFOH.
Material and methods
Nitrofurazone (NF) (5-nitro-furfurylidenesemicarbazone) was from Avocado (Heysham, UK), and potassium carbonate was from Ecibra (São Paulo, Brazil). All other reagents were analytical grade from Merck (Darmstadt, Germany).
Synthesis23
5-Nitro-2-furfurylidenesemicarbazone (NF) (0.99 g, 5 mmol) was mixed with potassium carbonate (0.69 g, 5 mmol) and suspended in water (10 mL). Formaldehyde solution (37% v/v, 18 mL) was added in two steps, half at the beginning of the reaction and the other half 3.5 h later. The mixture was stirred at room temperature for 7 h and then filtered. The filtrate was evaporated under low pressure. The product, NFOH, was crystallized from methanol–water (6:0.1), and yellow crystals (85%) were obtained. The compound was identified as follows: melting point (150–154°C), 1H NMR (300 MHz, DMSO-d6, δ, ppm): 11.02 (s, 1H, H8), 7.81 (d, J = 3.9 Hz, 1H, H4—superimposed signals), 7.80 (s, 1H, H6)*, 7.64 (t, J = 6.3 Hz, 1H, H12), 7.25 (d, J = 3.9 Hz, 1H, H3), 5.57 (t, J = 6.9 Hz, 1H, H14), 4.61 (t, J = 6.6 Hz, 2H, H13); 13C NMR (75 MHz, DMSO-d6, δ, ppm): 154.47 (C9), 152.42 (C5), 151.25 (C2), 127.82 (C6), 115.11 (C4), 112.63 (C3), 63.07 (C13); IR (KBr, δ, cm−1): 3410 (ν O—H), 3334 and 3162 (ν N—H), 2980 and 2860 (ν C—H), 1674 (ν CO), 1522 and 1359 (ν NO2); and mass spectrometry: m/z: 228 [M+], 210, 198, 155.
Cruzain inhibitory assay
Cruzain truncated in the C-terminal extension was obtained from Escherichia coli (strain DH5a containing the expression plasmid), which was kindly supplied by J. H. McKerrow, from the University of California, San Francisco, USA, following the procedure previously describedCitation24. The substrate Bz-Phe-Arg-AMC was purchased from Sigma (St Louis, MO, USA).
The recombinant cruzain enzyme (0.23 nM) in 1 mL of buffer pH 6.5 (Na2PO4 50 mM; EDTA 2.5 mM) was incubated for 1 h in the presence or not of increasing concentrations of the compound under study (NF or NFOH); inhibitor concentrations used in μM: 0, 5, 10, 15, 20, 40, and 80. Then, 5 μL of the substrate (Bz-Phe-Arg-AMC) stock solution (170 μM) was added and the activity of the enzyme was determined through fluorimetric measurements on a Hitachi F4500 spectrofluorimeter (excitation: 380 nm; emission: 460 nm) at 37°C, and under magnetic stirringCitation15. The IC50 of enzymatic inhibition was calculated using the program GraFit 5.0Citation25.
Molecular modeling
Molecular modeling studies were performed using Spartan’02 for Linux, version 119a (Wavefunction Inc.). The MMFF94 force field was employed to construct the chemical structures and initial energy minimization for angle and bond distance correctionsCitation26. Complete geometry optimization and conformational analyses were performed using the AM1 semi-empirical method. In order to better evaluate the conformational features, systematic analyses were conducted adopting a step size of 30°, with 0–360° of rotation freedom, for the dihedral angles of the side-chainCitation27. The Hamiltonian Austin Model 1 (AM1) was chosen to model both NF and NFOH. This semi-empirical quantum chemical method is based on molecular orbitals and involves calculations of the electron valences in atomsCitation28.
The resulting minimal energy conformers were adopted for NF and NFOH. The two selected conformers (gas phase) had their stereo properties and electronic surfaces evaluated according to the single point energy calculation. The electrostatic atomic charges were calculated. Maps of molecular electrostatic potentials (MEPs) showing the total electron density surface were also calculated. In this work, the MEP isoenergy contour was generated in a −65 to +45 kcal/mol range and superimposed onto a surface of constant electron density of 0.002 e/au3. The LUMO (lowest unoccupied molecular orbital) orbitalar distribution and LUMO surface (0.000 (red) to 0.012 (blue) kcal/mol, superimposed onto an isodensity of 0.032 e/au3) were also calculated in order to better evaluate the electronic availability to cruzain nucleophilic attack selectivity. The constant values employed in these calculations are default parameters of the software employed.
Results and discussion
Cruzain inhibitory assay
As mentioned in the “Introduction”, NFOH was shown to present better antichagasic activity than NF. Since some studies have demonstrated that inhibition of cruzain can stop the development of T. cruziCitation17, cruzain inhibitory tests were carried out with NF and NFOH in order to find out whether these compounds are able to inhibit this enzyme.
In preliminary studies of cruzain inhibition, NF and NFOH were active. According to the results, a concentration of 10 μM NF inhibited 30% of enzyme activity, while at the same concentration its derivative NFOH inhibited 60% of enzyme activity.
We also obtained IC50 values for NF and NFOH using data from the dose-response of cruzain inhibiton in fluorimetric analysis (). Values of IC50 obtained for NF and NFOH were 22.83 ± 1.2 μM and 10.55 ± 0.81 μM, respectively. Although previous work has reported thiosemicarbazone cruzain inhibitors with IC50 values around 300 nMCitation17, the values obtained in this work can still be considered promising, and suggest these compounds as lead structures for the future development of novel cruzain inhibitors, principally regarding NFOH. The curves obtained from NF and NFOH assays are depicted in .
Molecular modeling
Systematic conformational analyses were performed to investigate the conformational behavior and to obtain the minimal global energy conformer of each compound studied in this work. Additionally, since various different lower energy conformers have been observed, these studies might indicate which conformer would be the most stable in solution when all sets of structures are compared. The conformational changes were important in providing the rational basis to explain the increase, decrease, or even the loss of antichagasic activity of the compounds. The geometry of these conformers in gas and solution phases was found to be similar for NF and NFOH. The global minimal energy conformer in the gas phase was chosen for calculation of the single point energy and energy surfaces in order to interpret the stereolectronic properties.
The AM1 method provided good predictions of conformation, reproducing electrochemical behavior and fitting experimental heats of formation of nitro compoundsCitation29–31. Molecular modeling results obtained by the AM1 method applied to NF and NFOH are presented in . The geometry of the minimal energy conformer of NFOH in the gas phase was found to be similar to that of the crystal structure of the compoundCitation24. This observation reinforces the AM1 semi-empirical as a good method for application in the development of models for this class of compounds. In addition, the selected minimal global energy conformers showed a planar structure for NF. For NFOH the minimal global energy conformer showed the molecule to be essentially planar except in the region of the hydroxymethyl group, where a different spatial orientation was observed (bond angles N4C7O5 113.1º and C7O5H5 108.1º), also in agreement with crystallography data (112.6 and 109º, respectively)Citation24.
Figure 4. Minimal energy conformers (gas phase) of NF (left) and NFOH (right) calculated by the AM1 method represented in the (A) tube model and corresponding electronic surfaces: (B) MEPs (discrete distribution on level 7) in the range of −65 (intense red) to 30 (intense blue) kcal/mol (0.002 e/au3); (C) LUMO orbitalar distribution (0.032 eV); (D) LUMO maps (discrete distribution on level 7) (0.000 (red) to 0.012 (blue) kcal/mol). Atom color code: C (gray), O (red), N (blue), and H (white).
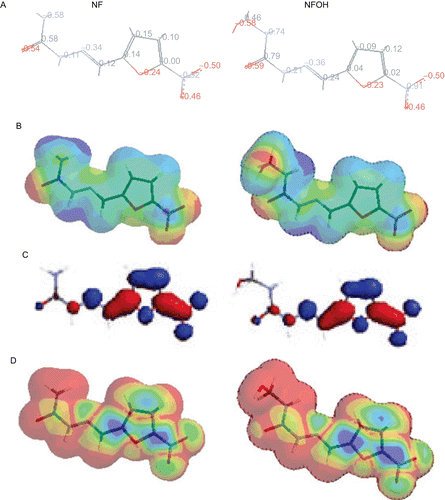
Du et al. studied over 100 semicarbazones and thiosemicarbazones (such as 5-(3-chlorophenyl)-2-furancarboxaldehyde semicarbazone and 3´-bromopropiophenone thiosemicarbazone) and suggested a mechanism of action for these compounds by nucleophilic attack by cruzain’s Cys-25 of the carbonyl carbon of the semicarbazone groupCitation17. The stereoelectronic properties of NF and NFOH were evaluated, and attack of the semicarbazone carbonyl group by the Cys-25 of cruzain was predicted. Atomic charges were calculated and showed the carbonyl carbon of NFOH (0.79) to be more positive relative to NF (0.58) (). This indicates that NFOH is more susceptible to nucleophilic attack, which is in accordance with the cruzain inhibitory assay.
Analyses of the MEPs pointed to lowest electron density over the region of the carbonyl carbon of NFOH, represented by a continuous blue region, when compared to that of NF, a sharpe blue region in the carbonyl carbon. In addition, analyses of the LUMO orbitalar distribution and LUMO surface have also demonstrated poorest electronic distribution on the carbonyl carbon of NFOH. Observation of the major lobules in LUMO distribution maps led to the conclusion that the NFOH carbonyl carbon is more susceptible to cruzain Cys-25 nucleophilic attack than the NF carbonyl carbon (). This effect can also be observed in , which represents the LUMO surface. A larger green region is observed on the NFOH carbonyl carbon, indicating lower electron density around this group in comparison to NF, and suggesting that it is more favorable for nucleophilic attack. MEPs, LUMO distribution, and LUMO surface results corroborate the atomic charges () showing that the nitrogen neighboring the carbonyl carbon in NFOH has a minor charge in relation to NF, suggesting that this carbonyl group is more susceptible to nucleophilic attack in accordance with the mechanism of action proposedCitation17.
On the other hand, the electronic distribution in the nitrofuran ring according to MEPs, LUMO distribution, and LUMO surfaces () is very similar in NF and NFOH compounds, suggesting a similar potential for nitro radical ion formation and consequently the same inhibitory activity of trypanothione reductase. Atomic charges calculated for the two molecules as represented in show a very similar distribution in the nitrofuran ring, thus indicating that nitro radical formation is not favored in one compound over the other. This was previously demonstrated by voltammetric studies, in which NFOH presented the same behavior as NF in terms of electroactivityCitation32.
Conclusion
Biological assays show that NF and NFOH present cruzain inhibitory activity, the latter being more active than the former. Stereoelectronic properties generated in molecular modeling represented by MEPs, LUMO distribution, LUMO surfaces, and atomic charges suggest that the most probable nucleophilic attack is of the carbonyl carbon of NFOH, in accordance with the cruzain inhibitory assay and the mechanism of action proposed.
Considering the potential of NFOH for cruzain inhibition and its ability to form the nitro radical ion, both evidenced in the molecular modeling approach, a dual mechanism of action can be envisioned to explain the high activity of this compound. In this sense, a two-point-attack molecule could decrease resistance in comparison to one-point-attack molecules. The importance of this finding resides not only in the suggestion of a dual mechanism of action by NF and NFOH against T. cruzi, but even more in the potential of NFOH as a lead compound for the rational design and development of new and more powerful drugs to treat Chagas’ disease.
Acknowledgments
This work was supported by grants from FAPESP (Project 01/01192-3, PhD Fellowship No. 04/06097-7 to G.H.G.T. and No. 02/09518-8 to A.M.), CAPES-PRODOC (to C.M.S.M), PROBRAL, CNPq (Research Fellowship), and PRP-USP Procontes. The authors wish to thank Prof. Dr. Cassius Vinicius Stevani, IQ-USP, for use of the spectrofluorimeter.
References
- World Health Organization. Geneva: WHO, 2002. http://www.who.int/topics/chagas_disease/en/.
- Coura JR, de Castro SL. A critical review on Chagas’ disease chemotherapy. Mem Inst Oswaldo Cruz 2002;97:3–24.
- Moncayo A. Chagas’ disease: current epidemiological trends after the interruption of vectorial and transfusional transmission in the Southern Cone countries. Mem Inst Oswaldo Cruz 2003;98:577–91.
- Moncayo A, Ortiz Yanine MI. An update on Chagas’ disease (human American trypanosomiasis). Ann Trop Med Parasitol 2006;100:663–77.
- de Andrade ALSS, Zicker F, de Oliveira RM, Silva SAE, Luquetti A, Travassos LR, et al. Randomised trial of efficacy of benznidazole in treatment of early Trypanosoma cruzi infection. Lancet 1996;348:1407–13.
- Dodd MC, Stillman WB. The in vitro bacteriostatic action of some simple furan derivatives. J Pharmacol ExpTher 1944;82:11–18.
- Andrade ZA, Brenner Z. Ação da nitrofurazona (5-nitro-2-furaldeído-semicarbazona) sobre as formas intracelulares do Trypanosoma cruzi na doença de Chagas experimental. Rev Inst Med Trop S Paulo 1969;11:222–8.
- Henderson GB, Ulrich P, Fairlamb AH, Rosenberg I, Pereira M, Sela M, et al. Engineering the substrate specificity of glutathione reductase toward that of trypanothione reduction. Proc Natl Acad Sci USA 1988;88:8769–73.
- La-Scalea MA, Chung MC, Cruz ML, Serrano SHP, Ferreira EI. Dissociation and electrooxidation of primaquine diphosphate as an approach to the study of anti-chagas prodrugs mechanism of action. Bioeletrochemistry 2001;53:55–9.
- Chung MC, Guido RVC, Martinelli TF, Goncalves MF, Polli MC, Botelho KCA, et al. Synthesis and in vitro evaluation of potential antichagasic hydroxymethylnitrofurazone (NFOH-121): a new nitrofurazone prodrug. Bioorg Med Chem 2003;11:4779–83.
- Sajid M, McKerrow JH. Cysteine proteases of parasitic organisms. Mol Biochem Parasitol 2002;120:1–21.
- Cazzulo JJ, Stoka V, Turk V. Cruzipain, the major cysteine proteinase from the protozoan parasite Trypanosoma cruzi. Biol Chem 1997;378:1–10.
- McGrath ME. The lysosomal cysteine proteases. Annu Rev Biophys Biomol Struct 1999;28:181.
- Melo RL, Pozzo RCB, Alves LC, Perissutti E, Caliendo G, Santagada V, et al. Synthesis and hydrolysis by cathepsin B of fluorogenic substrates with the general structure benzolyl-X-ARG-MCA containing non-natural basic amino acids at position X. Biochim Biophys Acta 2001;1547:82–94.
- Alves LC, Melo RL, Cezari MHS, Sanderson SJ, Mottram JC, Coombs GH, et al. Analysis of the S-2 subsite specificities of the recombinant cysteine proteinases CPB of Leishmania mexicana, and cruzain of Trypanosoma cruzi, using fluorescent substrates containing non-natural basic amino acids. Mol Biochem Parasitol 2001;117:137–43.
- Brak K, Doyle PS, MacKerrow JH, Ellman JA. Identification of a new class of nonpeptidic inhibitors of cruzain. J Am Chem Soc 2008;130:6404–10.
- Du XH, Guo C, Hansell E, Doyle PS, Caffrey CR, Holler TP, et al. Synthesis and structure-activity relationship study of potent trypanocidal thiosemicarbazone inhibitors of the trypanosomal cysteine protease cruzain. J Med Chem 2002;45:2695–707.
- Aguirre G, Boiani L, Cerecetto H, Fernandez M, Gonzalez M, Denicola A, et al. In vitro activity and mechanism of action against the protozoan parasite Trypanosoma cruzi of 5-nitrofuryl containing thiosemicarbazones. Bioorg Med Chem 2004;12:4885–93.
- Gasteiger J. The central role of chemoinformatics. Chemometr Intell Lab Syst 2006;82:200–9.
- Stahl M, Guba W, Kansy M. Integrating molecular design resources within modern drug discovery research: the Roche experience. Drug Discov Today 2006;11:326–33.
- Parise R, Menezes CMS, Pinto PLS, Paula GA, Brandt CA, da Silveira MAB. Design, synthesis and in vivo evaluation of oxaminiquine methacrylate and acrylamide prodrugs. Bioorg Med Chem 2007;15:1229–36.
- Rosselli FP, Albuquerque CN, Da Silva ABF. A chemometric study of megazol derivatives with activity against Trypanosoma equiperdum. SAR QSAR Environ Res 2006;17:533–47.
- Doriguetto AC, Silva CHTD, Ellena J, Trossini GHG, Chin CM, Ferreira EI. 5-Nitro-2-furaldehyde N-(hydroxymethyl)semicarbazone. Acta Crystallogr 2005;E-61:2099–101.
- Eakin AE, Mills AA, Harth G, McKerrow JH, Craik CS. The sequence, organization, and expression of the major cysteine protease (cruzain) from Trypanosoma cruzi. J Biol Chem 1992;267:7411–20.
- Leatherbarrow RJ. GraFit Version 5. Horley, UK: Erithacus Software Ltd., 2001.
- Halgren TA. Merck molecular force field. I. Basis, form, scope, parametrization, and performance of MMFF94. J Comp Chem 1996;17:490–519.
- Debnath AK, Decompadre RLL, Debnath G, Shusterman AJ, Hansch C. Structure activity relationship of mutagenic aromatic and heteroaromatic nitro-compounds – correlation with molecular-orbital energies and hydrophobicity. J Med Chem 1991;34:786–97.
- Dewar MJS, Zoebisch EG, Healy EF, Stewart JJP. The development and use of quantum-mechanical molecular-models .76. AM1 – a new general-purpose quantum-mechanical molecular-model. J Am Chem Soc 1985;107:3902–9.
- Lehmann MW, Singh P, Evans DH. Potential inversion in the reduction of trans-2,3-dinitro-2-butene. J Electroanal Chem 2003;549:137–43.
- Chen C, Wu JC. Correlations between theorical and experiemtnal determination of heat of formation of cartain aromatic nitro compounds. Comp Chem 2001;25:117–24.
- Chen PC, Wu JC, Chen SC. Correlations between theoretical and experimental determination of heat of formation of certain aliphatic nitro compounds. Comp Chem 2001;25:439–45.
- La Scalea MA, Menezes CMS, Juliao MSD, Chung MC, Serrano SHP, Ferreira EI. Voltammetric behavior of nitrofurazone and its hydroxymethyl prodrug with potential anti-Chagas activity. J Braz Chem Soc 2005;16:774–82.