Abstract
We report the detailed studies on the inhibitory effect of tannic acid (TA) on Clostridium histolyticum collagenase (ChC) activity against degradation of extracellular matrix component of collagen. The TA treated collagen exhibited 64% resistance against collagenolytic hydrolysis by ChC, whereas direct interaction of TA with ChC exhibited 99% inhibition against degradation of collagen and the inhibition was found to be concentration dependant. The kinetic inhibition of ChC has been deduced from the extent of hydrolysis of N-[3-(2-furyl) acryloyl]-Leu-Gly-Pro-Ala (FALGPA). This data provides a selective competitive mode of inhibition on ChC activity seems to be influenced strongly by the nature and structure of TA. TA showed inhibitor activity against the ChC by molecular docking method. This result demonstrated that TA containing digalloyl radical possess the ability to inhibit the ChC. The inhibition of ChC in gaining new insight into the mechanism of stabilization of collagen by TA is discussed.
Introduction
The matrix metalloproteinases (MMPs) are a large family of calcium-dependent zinc-containing endopeptidases, which are responsible for remodeling and degradation of the extracellular matrix (ECM) and also MMPs-1, 8, 13, 18 (collagenase I, II, III and IV) are capable of degrading native interstitial collagens I, II and III at a specific site at the ¾ length from the N-terminus of the molecules. Therefore, in pathophysiological conditions, the developments of MMP inhibitors are important aid to treat some diseases such as arthritis, cancer and some other pathological diseases, etc.Citation1.
Tannic acid (TA, Penta-m-digalloyl-glucose), a specific commercial form of tannin, is a hydrolyzable polyphenol composed of a central glucose (Glc) molecule derivatized at its hydroxyl groups with one or more digalloyl residues. Similar to many other plant polyphenols, TA possesses anti-oxidant, anti-inflammatory, anti-microbial, anti-proteolytic effects, anti-mutagenic effectCitation2. It is known to form multiple hydrogen bonds with proteins, particularly those rich in proline such as elastin and collagen and used in the manufacture of gelatin product and leather adhesivesCitation3,Citation4. It has been suggested as a potential cross-linking agent and improves the elastin and collagen stability in cardiovascular implants as evidenced by an increased resistance to proteolytic degradation against collagenase activityCitation5,Citation6. The inhibition of MMPs caused by plant polyphenolics was confirmed by gelatin zymography and observed for MMPs associated with various connective tissuesCitation7,Citation8. Plant polyphenolics were tested for their ability to inhibit the prokaryotic and eukaryotic cell derived collagenase activity. Pre-incubation of collagenase with plant polyphenol reduced the collagenase activity as wellCitation9,Citation10.
In addition to MMPs-collagenases, there are also other enzymes such as bacterial collagenases, which can degrade collagen. One such bacterial collagenase known as Clostridium histolyticum collagenase (ChC), which belongs to the family of M-31 metalloproteinase family, is capable of hydrolyzing triple helical region of the collagen molecules under physiological conditions as well as many synthetic peptidesCitation11,Citation12. The MMPs-collagenases and ChC, though relatively different, are considered to act through the same mechanism of action in the hydrolysis of collagens and collagen-like synthetic peptidesCitation13–16.
In this study, we evaluated the protective effect of collagen by TA against collagenase degradation. In order to understand the role of TA, the mode and degree of inhibition of ChC has now been attempted.
Materials and methods
Materials
All reagents and chemicals used were of analytical grade. TA, Clostridium histolyticum collagenase (Type IA) (ChC) and N-[3-(2-furyl) acryloyl]-Leu-Gly-Pro-Ala (FALGPA) were sourced from Sigma chemicals Co., USA. All other reagents and chemicals were sourced from SRL Ltd., India.
Treatment of TA with RTT
Tendons from tails of 6-month-old male albino rats (Wistar strain) were teased and washed with 0.9% NaCl at 4°C to remove the adhering muscles and other soluble proteins. The RTT was subsequently washed extensively in double distilled water and treated with 20–160 µM TA for 24 h at room temperature (27°C) without any agitation. After 24 h, the TA treated RTT was stored in water at 4°C before testing for resistance to ChC.
ChC hydrolysis of TA treated RTT
The percentage (%) of collagen degradation against ChC activities was calculated by the method described earlierCitation17. The cleavage of native and TA treated RTT collagen was monitored by the release of soluble form of Hyp from insoluble collagenCitation18. The residue free of acid was made up to a known volume and the % of Hyp was determined using the methodCitation19.
Monitoring of collagen degradation by inhibition of ChC activity
The % of collagen degradation by native and TA treated ChC was calculated by the methodCitation17. The % of hydroxyproline was determined using the methodCitation19. % Inhibition of ChC by TA was calculated as differences in the % degradation of RTT collagen treated by native and TA treated ChC.
Kinetic investigations on inhibition of ChC by TA
Inhibition of ChC assay using FALGPA as the substrate was performed according to the method reported earlierCitation17,Citation20,Citation21.
Molecular docking of ChC-TA interaction
The molecular docking studies comprise the determination of the three-dimensional structure of the TA by energy minimization methods and the simulation of the interaction between TA and ChC active site (Accelrys Discovery Studio 2.5). The ChC and TA structure was obtained from Protein Data Bank (structure 1NQD) PubChem (CID: 16129778) for this study.
Results
We have carried out a comprehensive study to determine the role of TA interaction with collagen and inhibition of collagenolytic activity of ChC against ECM component of collagen degradation. It is profound interest to establish how TA exhibits dose-dependent inhibition to ChC activity. The polymeric structure of TA molecule, mainly Glc esters of the gallic acid units, is shown () and chemical formula is given as C76H52O46.
Figure 1. Canonical surface of energy minimized structure of tannic acid (TA, Penta-m-digalloyl-glucose).
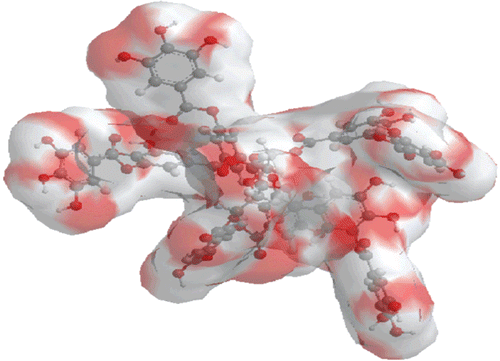
Stability of TA treated RTT collagen against ChC activity
% Collagen degradation (based on Hyp released) for the native and TA treated RTT collagen fiber by ChC at 72 h was determined. This reaction was carried out in 0.04 M CaCl2 solution buffered at pH 7.2 with 0.05 M tris HCl. The collagen degradation was 77, 55, 52 and 41% observed for TA at concentrations 20, 40, 80, 160 µM, respectively. Significant reduction in the degradation of collagen has been observed for the fibers treated with the TA compared to native RTT collagen fibers. The TA treated RTT collagen fibers exhibited 36% degradation (64% resistance to ChC activity) of collagen as against 93% degradation in the case of native collagen at 72-h period of incubation. TA effectively inhibited ChC breakdown of collagen at concentrations as low as 20 µM reduced collagen degradation by approximately 68% after 72-h incubation. At a TA concentration of 160 µM there was over 36% inhibition of ChC degradation of collagen after 72-h incubation. The TA can interact with collagen through hydrogen bonding and hydrophobic interactions. The hydrophobic core of the TA molecule, likely incorporates itself into hydrophobic areas, while hydroxyl moieties of the TA may establish multiple hydrogen bonds with neighboring collagen molecules, resulting in improved stabilization of collagen and prevent the free access of ChC to reactive sites on the collagen chains. A schematic representation of interaction of collagen with phenolic group of TA leading to polymerization, complex formation is shown (). The stability of TA treated collagen fibers against ChC would have been brought about by protecting the reactive sites in collagen through interaction with TA recognized by ChC. The significant differences in the enzymatic stability offered by TA could be due to the effectiveness of the latter in exhibiting better interaction with collagen through multiple hydrogen-bonded cross-links. It is an important probe for further the ability of this TA in the direct inhibition of ChC.
Inhibition of ChC by TA against RTT collagen degradation
Inhibition of ChC activity was tested using RTT collagen fibers as a substrate. A known amount of ChC was incubated with 20, 40, 80 and 160 μM of TA solution for 18 h in 1 mL of 0.1 M Tris-HCl (pH 7.4) containing 0.05 M CaCl2 at 25°C. The ChC inhibition was 98, 99, 99.4, 99.8 and 99.9% observed for TA at low concentration 20, 40, 80, 180 μM, respectively. TA effectively inhibited ChC breakdown of collagen at concentrations as low as 20 µM reduced collagen degradation by approximately 98% after 72-h incubation. At a TA concentration of 160 µM, there was over 99% inhibition of ChC degradation of collagen after 72-h incubation. The TA treatment to ChC exhibited the dose-dependent inhibition on the collagenolytic activity against RTT collagen fiber degradation. Almost complete inhibition of ChC was observed when TA was used at concentrations higher than 20 µM. The inhibition increased with increasing concentration of TA at 160 µM concentration, 99% inhibition has been observed against ChC hydrolysis after 72 h. This is indicative of the effectiveness of TA in direct inhibition of ChC. TA having additional Glc esters of digalloyl units and hydroxyl groups can exhibit better hydrogen bonding and hydrophobic interactions with ChC and hence can influence significant changes in the conformation of ChC resulting in high inhibition of ChC activity against collagen degradation. The difference in the inhibition of ChC was statistically significant (Student’s t-test, p < 0.0005). TA demonstrated higher inhibitory properties against ChC.
Kinetic analysis of inhibition of ChC
Inhibition kinetics of ChC activity was measured in the presence of increasing FALGPA concentrations and increasing TA concentrations. The Lineweaver–Burk plot, i.e., Double-reciprocal plot and Dixon plot for the hydrolysis of FALGPA by TA treated ChC have been determined (). The kinetic parameters like Km, Vmax and Ki were calculated and tabulated ().
Table 1. Michaelis–Menten parameters for ChC hydrolysis of FALGPA at 25°C, pH 7.5 in the presence of varying concentrations of TA.
Inhibition kinetics of ChC by TA
The velocity (v) of the reaction was calculated for each substrate concentration (S), and the data were presented in Lineweaver–Burk plot (1/v vs. 1/S) to determine the kinetic parameters. The Lineweaver–Burk plots, i.e., Double-reciprocal plots obtained for FALGPA hydrolysis by ChC incubated in the presence of different concentrations of TA revealed that there was no change in the 1/Vmax compared to control. We know that Vmax is unchanged by the presence of a competitive inhibitor. The TA exhibited the dose-dependent inhibition on ChC activity and Lineweaver–Burk plots clearly showed a competitive mode of inhibition ().
Determination of Michaelis–Menten constant
The Michaelis–Menten constant (Km) obtained from Lineweaver–Burk plot for native ChC was Km = 0.55 mM for the substrate FALGPA at 25°C pH = 7. This Km value of the FALGPA hydrolysis by ChC is found to be similar to that obtained earlierCitation19. The Vmax was found to be 0.2439 ± 0.05 mmol s−1. From the Michaelis–Menten constants (), the addition of 160 μM of TA to ChC resulted in Km values of 3.33 ± 0.007 mM. And those six lines obtained with the enzyme only and five concentrations of TA intercept at the same position on the Y-axis indicate that Vmax is the same in all cases (). Furthermore, the intercept of the lines on the x-axis demonstrates that the Km value increase when the TA concentration increases. This is a characteristic of the competitive mode of inhibition.
Determination of inhibition constant
The inhibition constant Ki is obtained from a Dixon plot of V−1 vs. various concentrations of TA and FALGPA. For the determination of Ki of TA against ChC activity, the velocity of cleavage of FALGPA was measured at TA concentrations ranging from 10 to 160 µM. In this plot, the reciprocals of velocity were plotted against inhibitor concentrations at different concentrations of FALGPA. A series of straight lines was obtained, converging to the same point, and that value represents −Ki. It was found that the Ki for TA is 20 µM. The competitive mode of inhibition exhibited by TA may work through direct competition with the FALGPA by binding to the active site or binding to a remote site and causing a conformational change in the ChC. Both the mechanisms give identical positive results. It is important to study whether there are any changes in the conformation of ChC on the binding of the TA.
Molecular docking of ChC-TA interaction
Molecular docking of ChC-TA interaction was carried out action of TA. and shows the closest structure to the ChC structure, where the contact surfaces are colored according to their distance. The best geometries obtained through the docking represent the low interaction energy between TA-ChC, resulting in stronger chemical bonds. Among them stand out hydrogen bonds, whose involvement of groups of ChC active site can explain the effect on the ChC activity. ChC is represented by its solvent-accessible surface. The light orange regions represent the possible hydrogen bonds and the pale gray regions are involved in hydrophobic interactions. These representations clearly show the possible anchoring points of TA digalloyl moieties with the ChC active site.
Discussion
The pathophysiological conditions are generally associated with enhanced activity of lysosomal enzymes and MMPs, such as carbonic anhydrases (CAs) and also pathogenic microbial collagenases are responsible for the efficient degradation of all components of the ECM and related to some carcinogenic and tumor progression processes. The ChC hydrolyzes collagen resulting in degradation of ECM. Recently, MMPs, CAs and ChC inhibitors in the drug design have been studiedCitation22–25. The phenolic acids and phenol natural products were investigated for their inhibitory effects against the metalloenzyme CAsCitation26. Therapeutic use of plant polyphenolic on inhibition of ChC for drug design is of great pharmaceutical interest. The role of polyphenolics on degenerative diseases such as arthritis has also been under research. The nature of interaction of polyphenolics with ECM and inhibition of MMPs and CAs activities are currently under investigation. The focus of the present work is on the potential of TA on control of ChC activity against collagen degradation.
In this present study, we have investigated the inhibition of collagen degradation by ChC using TA that might stabilize collagen through hydrogen bonding. In order to measure collagen degradation, a method that measured ChC-induced degradation of collagen by Hyp assay method was used, similar to previously describedCitation17. Using this Hyp method, the time course of ChC degradation of collagen and inhibitory effect of TA was monitored. This study also demonstrates that TA on competitive inhibition of ChC in the dose-dependent manner.
Collagen-TA-ChC interactions
Collagen-TA interactions
The collagen can interact with TA which is mediated by hydroxyl group through specific side chain, as well as by available carbonyl and amide groups present in the collagen molecule as well as minor roles of both ionic and covalent bonding effects. The hydrophobic interactions of penta-m-digalloyl units of TA with hydrophobic residues of collagen are the major forces involved in the stabilization of collagen. There are many hydroxyl groups present in the TA which can act as hydrogen bond donor/acceptor with different side chain groups of amino acids of collagen namely, aspartic acid (Asp), asparagine (Asn), hydroxyproline (Hyp), lysine (Lys) and serine (Ser). Previous report has also indicated the interaction of collagen with green tea polyphenolCitation27. In addition, vegetable tannins have a long history of use in stabilizing collagen in the leather industry and are known to strongly binding to collagensCitation28. The gallotannin groups in large tannin molecules are known to provide extensive hydrogen bonding capacity with collagen and thus stabilize the collagen triple helical chains. More recently, catechin molecules also containing galloyl groups have been shown to stabilize collagen not only through H-bonding but also by hydrophobic interactions of the catechin and epigallocatechin gallate (EGCG) with collagen chainsCitation29. This was visual evidence of such binding in these studies whereby the collagen fiber became more stable on the addition of TA prior to the addition of ChC. It is likely that the inhibition of collagen degradation observed in this study arose from the stabilization of collagen by TA and the reduction of access of ChC to potential cleavage sites on the collagen chains. It is also possible that penta-m-digalloyl groups on TA (either free or bound to collagen) might as well bind the ChC, rendering it inactive. Hence, it could be inferred that the inhibition of ChC activity on collagen could be most likely due to the blocking of the reactive sites in collagen by TA, thereby ChC unable to degrade the collagen.
ChC-TA interactions
The inhibitory effects of TA on ChC activity against degradation of collagen have been investigated. In general, the TA was the stronger inhibitor than the other polyphenolics, and this implied that the hydroxyl group is important for the compounds to have higher inhibitory activity. To the best of our knowledge, this is the first report showing the ChC inhibitory activity by the TA. The TA-treated ChC exhibited a dose-dependent inhibition on the collagenolytic activity. Under the experimental condition of the present study, the range of low concentrations of TA that showed significant ChC inhibition was revealed. The inhibition of ChC increasing with increasing concentration of TA has been observed against RTT collagen degradation, which is indicative of effectiveness of the TA in direct inhibition of ChC compared to the inhibition exhibited through the binding with collagen. However, TA was found to effectively inhibit ChC-induced degradation of the collagen. These results suggest that the steric structure of TA containing digalloyl units is important for the inhibition of ChC activity. The green tea polyphenol, which also contains many galloyl groups, behaved similarly to TA, inhibiting ChC activity at low concentrations. These polyphenols have been previously reported to inhibit ChC degradation of collagen using lower concentrations. Binding studies also confirmed a strong binding interaction between both catechin and EGCG with collagenCitation28. It is likely that the mechanism of inhibition of collagen degradation is similar to that described for TA, which induced cross linking of collagen chains and protection of potential cleavage sites from ChC attack. The strong binding interaction of digalloyl containing TA arises from both H-bonding and hydrophobic interactions with the collagen chains. The particularly strong binding observed for TA arises from the high levels of digalloyl moieties flexibility on the TA molecule allowing for optimal molecular conformation to potential binding sites. Our results showed the efficacy of TA on blocking collagen degradation and inhibition of ChC against collagenolytic degradation. These results demonstrated that TA containing digalloyl radical possesses the ability to inhibit ChC.
ChC inhibition kinetics
The mode of ChC inhibition by TA was examined from different methods of analyzing the kinetics of the enzyme. The inhibition of ChC occurs through binding of TA to the active part of the enzyme or inhibiting the activation by binding to the ChC. The ability of different concentrations of TA to function as an inhibitor is deduced from the values of Vmax, Km and Ki, obtained for the hydrolysis of FALGPA by ChC. Double reciprocal or Lineweaver–Burk plots of reaction velocities against FALGPA concentrations in the presence and absence of TA () show a typical competitive inhibition profile where only the slopes are affected without any effect on the intercepts. These kinetic parameters influencing the structure of TA on inhibition of ChC activity against hydrolysis of FALGPA provides an insight into possible roles of the TA in the stabilization of collagen. The most convincing evidence in favor of TA was pure competitive inhibitors of ChC came from the graphs of the slopes of the relationship between 1 = Vmax and 1/FALGPA plotted against the inhibitor concentrations and replots of slopes from Dixon plots for each substrate concentration vs. corresponding reciprocal concentrations of FALGPA, which was a straight line passing through the origin. The Dixon and Lineweaver–Burk plots for TA, which are characteristic of competitive inhibition, seem to directly compete with FALGPA; the ChC could not bind FALGPA and inhibitor simultaneously because of the small FALGPA-binding pocket. We found that TA is a competitive mode of inhibition of ChC activity, with a Ki of 20 µM against FALGPA degradation. Previously, we reported the kinetics of inhibition of ChC by green tea polyphenolicsCitation27. Based on the accepted hydrolysis scheme for FALGPA by ChCCitation16, TA can interact with ChC at site of the free ChC to form ChC–TA complex. This results in decrease in hydrolysis of FALGPA with increasing inhibitor concentration. The observations of the present study on the behavior of TA may be interpreted as follows: the TA may compete with the substrate binding site (isosteric), which induces conformational changes in the substrate binding site (allosteric). It has been shown experimentally that the side chain carboxyl groups of aspartic (Asp) and glutamic acid (Glu) participate in conjunction with tyrosine residues in the stabilization of the active conformation of the native ChC. The competitive mode of ChC inhibition exhibited by TA may arise from the binding to the side chain carboxyl residues of aspartic (Asp) or glutamic acids (Glu), with the net result of change in the conformation of ChC. The hydroxyl groups of TA can act as hydrogen bond acceptor/donor with the backbone amide and other side chain functional groups viz., hydroxyl, amino and carboxyl groups of ChC. Based on the results of these studies, it can be concluded that the deactivation of ChC by TA takes place. Discussion on the observed data in the inhibition kinetics studies needs to take into account the structure-activity relationship of ChC with TA. Amino acid composition of ChC shows that the side chain carboxylic groups viz., Aps and Glu and their amides constitute nearly a fifth of the total amino acid content and also a similar position is observed in case of mammalian collagenase. The presence of Glu at the active sites of collagenase has been reported for MMPs-collagenase and bacterial collagenase. These ChC are distinguished by their ability to digest native triple-helical collagens (such as Types I, II and III) into small peptides. ChC degrades the helical regions in native collagen preferentially at the Y-Gly bond in the sequence -Pro-Y-Gly-Pro- where Y is most frequently a neutral amino acid. This bond in synthetic peptide substrates may also be split. Based on the reported molecular docking evidence, TA efficiently inhibit the ChC can be evinced. These molecular docking representations clearly show the possible anchoring points of digalloyl moieties with the ChC active site. Most importantly, electrostatic or hydrophobic interactions of TA greatly impair ChC activity. Recently, natural occurring polyphenol as the template for drug design have been studiedCitation30.
These studies have provided the convincing evidence to inhibition of ChC by TA against collagen degradation. Therefore, TA provides a selective competitive mode of inhibition of ChC seems to be influenced strongly by structure and nature of TA and could constitute an important aid in the prevention of arthritis as well as being part of an effective therapy in the treatment of joint diseases.
Acknowledgment
The first author acknowledges the Council of Scientific & Industrial Research (CSIR), New Delhi, Government of India, for his CSIR-SRF fellowship. The assistance of S. Muthukumar, Research Scholar, Marine Bioinformatics Centre, National Institute of Ocean Technology, Chennai, for molecular docking work is acknowledged.
Declaration of interest
The authors declare no conflicts of interest.
References
- Wang X, Li KF, Adams E, Van Schepdael A. Matrix metalloproteinase inhibitors: A review on bioanalytical methods, pharmacokinetics and metabolism. Curr Drug Metab 2011;12:395–410.
- Chen SC, Chung KT. Mutagenicity and antimutagenicity studies of tannic acid and its related compounds. Food Chem Toxicol 2000;38:1–5.
- Aewsiri T, Benjakul S, Visessanguan W, Wierenga PA, Gruppen H. Antioxidative activity and emulsifying properties of cuttlefish skin gelatin–tannic acid complex as influenced by types of interaction. Innov Food Sci Emerg Technol 2010;12:712–720.
- Isenburg JC, Karamchandani NV, Simionescu DT, Vyavahare NR. Structural requirements for stabilization of vascular elastin by polyphenolic tannins. Biomaterials 2006;27:3645–3651.
- Isenburg JC, Simionescu DT, Vyavahare NR. Elastin stabilization in cardiovascular implants: Improved resistance to enzymatic degradation by treatment with tannic acid. Biomaterials 2004;25:3293–3302.
- Bedran-Russo AK, Yoo KJ, Ema KC, Pashley DH. Mechanical properties of tannic-acid-treated dentin matrix. J Dent Res 2009;88:807–811.
- Tanimura S, Kadomoto R, Tanaka T, Zhang YJ, Kouno I, Kohno M. Suppression of tumor cell invasiveness by hydrolyzable tannins (plant polyphenols) via the inhibition of matrix metalloproteinase-2/-9 activity. Biochem Biophys Res Commun 2005;330:1306–1313.
- Gagliano N, Moscheni C, Torri C, Magnani I, Bertelli AA, Gioia M. Effect of resveratrol on matrix metalloproteinase-2 (MMP-2) and Secreted Protein Acidic and Rich in Cysteine (SPARC) on human cultured glioblastoma cells. Biomed Pharmacother 2005;59:359–364.
- Kim MM, Ta QV, Mendis E, Rajapakse N, Jung WK, Byun HG et al. Phlorotannins in Ecklonia cava extract inhibit matrix metalloproteinase activity. Life Sci 2006;79:1436–1443.
- Scozzafava A, Ilies MA, Manole G, Supuran CT. Protease inhibitors. Part 12. Synthesis of potent matrix metalloproteinase and bacterial collagenase inhibitors incorporating sulfonylated N-4-nitrobenzyl-beta-alanine hydroxamate moieties. Eur J Pharm Sci 2000;11:69–79.
- Scozzafava A, Supuran CT. Protease inhibitors: Synthesis of matrix metalloproteinase and bacterial collagenase inhibitors incorporating 5-amino-2-mercapto-1,3,4-thiadiazole zinc binding functions. Bioorg Med Chem Lett 2002;12:2667–2672.
- Scozzafava A, Supuran CT. Protease inhibitors: Synthesis of Clostridium histolyticum collagenase inhibitors incorporating sulfonyl-L-alanine hydroxamate moieties. Bioorg Med Chem Lett 2000;10:499–502.
- Scozzafava A, Supuran CT. Carbonic anhydrase and matrix metalloproteinase inhibitors: Sulfonylated amino acid hydroxamates with MMP inhibitory properties act as efficient inhibitors of CA isozymes I, II, and IV, and N-hydroxysulfonamides inhibit both these zinc enzymes. J Med Chem 2000;43:3677–3687.
- Mandl I, Zipper H, Ferguson LT. Clostridium histolyticum collagenase: Its purification and properties. Arch Biochem Biophys 1958;74:465–475.
- Miller EJ, Harris ED Jr, Chung E, Finch JE Jr, McCroskery PA, Butler WT. Cleavage of Type II and III collagens with mammalian collagenase: Site of cleavage and primary structure at the NH2-terminal portion of the smaller fragment released from both collagens. Biochemistry 1976;15:787–792.
- Vencill CF, Rasnick D, Crumley KV, Nishino N, Powers JC. Clostridium histolyticum collagenase: Development of new thio ester, fluorogenic, and depsipeptide substrates and new inhibitors. Biochemistry 1985;24:3149–3157.
- Krishnamoorthy G, Madhan B, Sadulla S, Raghava Rao J, Madhulatha W. Stabilization of collagen by the plant polyphenolics Acacia mollissima and Terminalia chebula. J Appli Polym Sci 2008;108:199–205.
- Ryan JN, Woessner JF Jr. Mammalian collagenase: Direct demonstration in homogenates of involuting rat uterus. Biochem Biophys Res Commun 1971;44:144–149.
- Woessner JF Jr. The determination of hydroxyproline in tissue and protein samples containing small proportions of this imino acid. Arch Biochem Biophys 1961;93:440–447.
- Van Wart HE, Steinbrink DR. A continuous spectrophotometric assay for Clostridium histolyticum collagenase. Anal Biochem 1981;113:356–365.
- Espenson JH, In: Chemical kinetics and reaction mechanisms, McGraw Hill, New York, 1981.
- Marques SM, Nuti E, Rossello A, Supuran CT, Tuccinardi T, Martinelli A et al. Dual inhibitors of matrix metalloproteinases and carbonic anhydrases: Iminodiacetyl-based hydroxamate-benzenesulfonamide conjugates. J Med Chem 2008;51:7968–7979.
- Supuran CT. Carbonic anhydrase inhibitors: Possible anticancer drugs with a novel mechanism of action. Drug development research 2008;69:297–303.
- Supuran CT, Winum JY. Chapter 30. Clostridium histolyticum collagenase inhibitors in the drug design. Drug design of zinc-enzyme inhibitors: Functional, structural, and disease applications: 2009.
- Riihonen R, Supuran CT, Parkkila S, Pastorekova S, Väänänen HK, Laitala-Leinonen T. Membrane-bound carbonic anhydrases in osteoclasts. Bone 2007;40:1021–1031.
- Innocenti A, Beyza Oztürk Sarikaya S, Gülçin I, Supuran CT. Carbonic anhydrase inhibitors. Inhibition of mammalian isoforms I-XIV with a series of natural product polyphenols and phenolic acids. Bioorg Med Chem 2010;18:2159–2164.
- Madhan B, Krishnamoorthy G, Rao JR, Nair BU. Role of green tea polyphenols in the inhibition of collagenolytic activity by collagenase. Int J Biol Macromol 2007;41:16–22.
- Liao XP, Lu ZB, Shi B. Selective adsorption of vegetable tannins onto collagen fibers. Ind Eng Chem Res 2003;42:3397–3402.
- Brown EM, QI PX. Exploring a role in tanning for the gap region of the collagen fibril: Catechin-collagen interactions. J Am Leather Chem Assoc 2001;103:290–297.
- Cuccioloni M, Mozzicafreddo M, Bonfili L, Cecarini V, Eleuteri AM, Angeletti M. Natural occurring polyphenols as template for drug design. Focus on serine proteases. Chem Biol Drug Des 2009;74:1–15.