Abstract
Cholinesterases are associated with pathology characteristic of conditions such as Alzheimer’s disease and are therefore, considered targets for neuroimaging. Ester derivatives of N-methylpiperidinol are promising potential imaging agents; however, methodology is lacking for evaluating these compounds in vitro. Here, we report the synthesis and evaluation of a series of N-methylpiperidinyl thioesters, possessing comparable properties to their corresponding esters, which can be directly evaluated for cholinesterase kinetics and histochemical distribution in human brain tissue. N-methylpiperidinyl esters and thioesters were synthesized and they demonstrated comparable cholinesterase kinetics. Furthermore, thioesters were capable, using histochemical method, to visualize cholinesterase activity in human brain tissue. N-methylpiperidinyl thioesters can be rapidly evaluated for cholinesterase kinetics and visualization of enzyme distribution in brain tissue which may facilitate development of cholinesterase imaging agents for application to conditions such as Alzheimer’s disease.
Abbreviations | ||
4-[11C]AMP,4-(N-[11C], | = | methyl)piperidinyl acetate |
4-[11C]PMP, | = | 4-(N-[11C]methyl) piperidinyl propionate |
11C-MP4B, | = | 4-(N-[11C]methyl) piperidinyl n-butyrate |
Aβ, | = | β-amyloid; AChE, acetylcholinesterase |
AD, | = | Alzheimer’s disease |
AV, | = | anteroventral nucleus |
BuChE, | = | butyrylcholinesterase |
DAB, | = | 3, 3′-diaminobenzidine tetrahydrochloride |
Km, | = | affinity constant |
MMFF, | = | Merk Molecular Force Field |
NFT, | = | neurofibrillary tangles. |
Introduction
The cholinergic system is affected in a number of disorders, such as Alzheimer’s disease (AD)Citation1–3, multiple sclerosisCitation4,Citation5 and primary brain tumorsCitation6. In the brain cholinergic system, two enzymes, acetylcholinesterase (AChE, EC 3.1.1.7) and butyrylcholinesterase (BuChE, EC 3.1.1.8), catalyze the hydrolysis of acetylcholine, thereby regulating the actions of this neurotransmitterCitation7. Changes in the activity and location of cholinesterases in neurological disorders render these enzymes suitable targets for neuroimaging in the living brain, to facilitate early diagnosis and treatment monitoring. In AD, cholinesterases, particularly BuChE, are associated with characteristic pathological hallmarks of the disease, β-amyloid (Aβ) plaques and neurofibrillary tangles (NFTs)Citation8–16.
In earlier attempts to realize cholinesterase imaging, several 11C-radiolabelled alkyl esters of N-methylpiperidinol were synthesized as AChE-specific imaging agents. Two such compounds, 4-(N-[11C]methyl)piperidinyl acetate (4-[11C]AMP) and 4-(N-[11C]methyl) piperidinyl propionate (4-[11C]PMP), were examinedCitation17–21 and provided some reproduction of the known brain distributions of AChECitation18,Citation21. Specific BuChE visualization was also attempted with 4-(N-[11C]methyl) piperidinyl n-butyrate (11C-MP4B)Citation22 but was unsuccessfulCitation23. Alternate types of BuChE imaging ligands, derived from N-methylpiperidinol and N-methyl pyrrolidinol, were subsequently synthesized and tested in a normal rat model systemCitation24. Using autoradiographic analysis these para-123iodobenzoate esters were found to have entered the brain and their distribution recapitulated, for the most part, the known distribution of BuChE therein. Based on these observations, N-methylpiperidinyl compounds remain promising agents for the imaging of cholinesterases in the living brain.
In vivo testing of the N-methylpiperidinyl cholinesterase imaging agents, 4-[11C]AMP, 4-[11C]PMP and 11C-MP4B, has been extensive. However, methodology for determination of their specificity and direct measurement of their actual affinity constants for AChE and BuChE has been difficult due to the lack of a suitable chromophore that would allow the use of sensitive spectrophotmetric methods. Tracking hydrolysis by radiolabelling reactants and productsCitation25 or by employing the chromophore of m-nitrophenol to tag reactants in a spectrophotometric approachCitation22 have both proved cumbersome for screening of compounds as potential imaging agents. Thus, a rapid in vitro evaluation method would be beneficial for the continued development of imaging agents derived from N-methylpiperidinol.
The Ellman spectrophotometric methodCitation26, or variations thereofCitation27, remains the most rapid, reliable and sensitive means to measure AChE or BuChE activity. The principle on which this assay is based is that, thioesters, such as acetylthiocholine and butyrylthiocholine, should be kinetically analogous to their corresponding esters with respect to cholinesterase catalysis. A kinetic analysisCitation28 comparing benzoylcholine and benzoylthiocholine supports this notion. In addition to their use in Ellman cholinesterase assays, thioesters are employed for in vitro histochemical detection of cholinesterase activity in tissues. One of the commonly used histochemical techniques for detection of AChE and BuChE is the Karnovsky-Roots methodCitation29. In this procedure, hydrolysis of acetylthiocholine and butyrylthiocholine generates a thiolate anion that leads to a precipitate in the area of enzyme activity. This precipitation facilitates detection of enzyme distribution under the microscope. Thus, in theory, any thioester susceptible to cholinesterase hydrolysis could be characterized using Ellman enzyme kinetics and should enable histochemical visualization of areas containing enzyme activity in brain tissue using the Karnovsky-Roots method.
Herein is described the synthesis, enzyme kinetics and histochemical evaluation, as cholinesterase substrates, of alkyl and aryl thioesters of N-methyl-4-piperidinethiol as an indirect means to evaluate potential cholinesterase imaging agents, such as the corresponding N-methyl-4-piperidinol esters.
Materials and methods
Materials and compound characterization
Materials used for synthesis and enzymatic evaluation of compounds are found in Supplementary Material. Compound characterization methods are also presented therein.
Brain tissues
Brain tissues used in this study were provided by the Maritime Brain Tissue Bank, (Halifax, Nova Scotia, Canada), following approval from the Capital Health Ethics Board, from the brain of a 90 year old female, removed within 20 h of death. The brain was immersion fixed in 10% formalin in 0.1 M phosphate buffer (pH 7.4) for approximately 94 h and cut in 1–2 cm slabs. These slabs were cryoprotected by immersion in increasing concentrations of sucrose, ranging from 10% to 40% in 0.1 M phosphate buffer (pH 7.4). The brain tissue was in each concentration of sucrose solution for approximately 48 h and was stored in 40% buffered sucrose (pH 7.4) with 0.6% sodium azide until used.
Synthesis of compounds
Synthesis of N-methylpiperidin-4-yl 4-cyanobenzoate (1)
Under an argon atmosphere, N-methylpiperidin-4-ol (0.92 g, 8.00 mmol) was dissolved in THF (15 mL). To this solution was added 1.6 M butyl lithium in hexanes (5.00 mL, 8.00 mmol) at −78°C followed by a solution of 4-cyanobenzoyl chloride (1.21 g, 7.30 mmol) in THF (20 mL) and the mixture was stirred at −78 °C for 16 h. Water (20 mL) was added to the reaction mixture and extracted with ethyl acetate (3 × 20 mL). The combined organic layers were dried over Na2SO4. The solvent was removed in vacuo to produce a yellow solid. The product was purified by silica gel chromatography (10:90 MeOH/CH2Cl2) to give a white solid (1.06 g, 59%). The solid was recrystallized from hexanes to afford white crystals. Analytical data: MP (hexanes): 119–120˚C. IR (Nujol): 2228, 1718, 1278, 1122, 1030 cm−1. 1H NMR (CDCl3): δ 1.92–2.03 (m, 2H), 2.10–2.18 (m, 2H), 2.44–2.56 (m, 2H), 2.42 (s, 3H), 2.72–2.87 (m, 2H), 5.08–5.19 (m, 1H), 7.77–7.79 (m, 2H), 8.15–8.17 (m, 2H). 13C NMR (CDCl3): δ 30.9 (2), 46.2 (3), 52.9 (2), 71.4 (1), 116.4 (0), 118.0 (0), 130.1 (1), 132.2 (1), 134.5 (0), 164.3 (0). EI-MS m/z: 244 (M+, 44%), 130 (22%), 114 (20%), 102 (29%, 98 (80%), 97 (100%), 96 (80%), 82 (32%), 70 (14%), 57 (10%), 55 (29%). HRMS (EI): M+ found 244.1216, calcd for C14H16N2O2+ = 244.1206.
Synthesis of N-methylpiperidin-4-yl 4-fluorobenzoate (2)
Under an argon atmosphere, N-methylpiperidin-4-ol (0.94 g, 8.16 mmol) was dissolved in THF (15 mL). To this solution was added 1.6 M butyl lithium in hexanes (5.00 mL, 8.00 mmol) at −78 °C followed by a 4-fluorobenzoyl chloride (0.88 g, 7.30 mmol) and the mixture was stirred at −78 °C for 16 h. Water (20 mL) was added to the reaction mixture and extracted with ethyl acetate (3 × 20 mL). The combined organic layers were dried over Na2SO4. The solvent was removed in vacuo to produce a yellow solid. The product was purified by silica gel chromatography (10:90 MeOH/CH2Cl2) to give a pale yellow solid (0.72 g, 42%). The solid was recrystallized from hexanes to afford white crystals. Analytical data: MP (hexanes): 48–49˚C. IR (Nujol): 1726, 1274, 1114, 852 cm−1. 1H NMR (CDCl3): δ 1.83–1.88 (m, 2H), 2.00-2.05 (m, 2H), 2.32 (s, 3H), 2.33–2.40 (m, 2H), 2.63–2.77 (m, 2H), 4.96–5.10 (m, 1H), 7.08–7.13 (m, 2H), 8.04–8.08 (m, 2H). 13C-NMR (CDCl3): δ 30.9 (2), 46.2 (3), 52.9 (2), 70.3 (1), 115.4 (JCF = 22 Hz, 1) 126.9 (0), 132.0 (JCF = 10 Hz, 1), 164.7 (JCF = 254 Hz, 0), 166.7 (0). EI-MS m/z: 237 (MH+, 30%), 123 (30%), 114 (15%), 98 (53%), 97 (100%), 96 (82%), 95 (31%), 82 (35%), 75 (12%), 70 (14%), 55 (28%). HRMS (EI): M+ found 237.1173, calcd for C13H16FNO2+ = 237.1160.
Synthesis of N-methylpiperidin-4-yl 4-iodobenzoate (3)
Synthesized according to a previously described procedureCitation24 and the analytical data was consistent and purity greater than 98%. Analytical data: MP: 128–130°C (Lit MP = 130°C26). IR (Nujol): 1711, 1585, 1283, 1268, 1118, and 754 cm−1. 1H NMR (CDCl3): δ 1.84–1.91 (m, 2H), 2.01–2.08 (m, 2H), 2.31–2.39 (m, 5H), 2.67–2.73 (m, 2H), 5.02–5.08 (m, 1H), 7.75 (d, J = 8.5 Hz, 2H), and 7.81 (d, J = 8.5 Hz, 2H). 13C NMR (CDCl3): δ 31 (2), 46 (3), 53 (2), 70 (1), 100 (1), 130, 131, 138, and 166. EI-MS m/z: 345(M+, 16%), 231 (12%), 203 (9%), 114 (11%), 97 (100%), 82 (22%), 70 (7%), 55 (14%). HRMS (EI): M+ found 345.0233, calcd for C13H16NO2I = 345.0226.
Synthesis of N-methyl-4-piperidinethiol
N-Methyl-4-piperidone (52.92 g, 469.7 mmol) was dissolved in isopropanol (200 mL). Hydrogen sulfide was bubbled through the solution using a glass frit at 0°C resulting in the precipitation of white solid, which was collected by suction filtration after 1 hour. The filtrate was again treated with hydrogen sulfide for an additional 30 min and all further white solid was collected by suction filtration for a total of 80.47 g. Sodium borohydride (16.62g, 439.3 mmol) was suspended in isopropanol (150 mL) followed by the slow addition of the white solid at 0˚C. The reaction mixture was returned to room temperature and stirred under an argon atmosphere for 16 h. Excess solvent was removed under reduced pressure to produce a white paste which was partitioned between 150 mL of both water and ether. The aqueous layer was extracted with ether (4 × 50 mL) and the combined organic layers washed with brine (2 × 30 mL) and dried over anhydrous sodium sulfate. The solvent was removed in vacuo to yield a colourless liquid which was distilled (BP: 168–172°C, lit. BP = 62°C at 0.8mm30); to yield the product as a clear colourless oil (21.22 g, 34%). IR (Neat): 3338, 2944, 1278, 1132 cm-1. 1H NMR (CDCl3): δ 1.55 (d, J = 7.0, 1H), 1.64–1.71 (m, 2H), 1.99–2.04 (m, 4H), 2.26 (s, 3H), 2.67–2.80 (m, 3H). 13C NMR (CDCl3): δ 35.5 (1), 36.9 (2), 46.1 (3), 55.2 (2). EI-MS m/z: 131 (M+ 32%), 98 (100%), 96 (12%), 70 (18%), 55 (27%). HRMS (ESI): MH+ found 132.0841, calcd for C6H14NS+ = 132.0841.
Synthesis of (N-methylpiperidin-4-yl) 4-cyanobenzenecarbothioate (4)
Under an argon atmosphere, triethylamine (0.53 mL, 3.80 mmol) was added to dichloromethane (20 mL), followed by N-methyl-4-piperidinethiol (0.45 mL, 3.80 mmol) and 4-cyanobenzoyl chloride (0.629 g, 3.80 mmol). The reaction mixture was stirred for 24 h at room temperature. The reaction was extracted with saturated NaHCO3 (3 × 20 mL) and the organic layer dried over Na2SO4. The solvent was removed in vacuo to yield a light yellow solid which was recrystallized from hexanes to afford pale yellow crystals (0.24 g, 24%). Analytical data: MP (hexanes): 152–153°C. IR (Nujol): 2229, 1655, 1208, 860 cm-1. 1H NMR (CDCl3): δ 1.82–1.89 (m, 2H), 2.10–2.15 (m, 2H), 2.25–2.33 (m, 2H), 2.35 (s, 3H), 2.74-2.85 (m, 2H), 3.72-3.82 (m, 1H), 7.74-7.76 (m, 2H), 8.02-8.04 (m, 2H). 13C NMR (CDCl3): δ 32.1 (2), 40.4 (1), 46.3 (3), 55.1 (2), 116.5 (0), 117.8 (0), 127.6 (1), 132.4 (1), 140.3 (0), 190.2 (0). EI-MS m/z: 260 (M+, 4%), 130 (54%), 102 (20%), 98 (100%), 97 (81%), 96 (30%), 70 (15%), 55 (22%), 44 (10%), 42 (18%). HRMS (ESI): MH+ found 261.1056, calcd for C14H17N2OS+ = 261.1056.
Synthesis of (N-methylpiperidin-4-yl) 4-fluorobenzenecarbothioate (5)
Under an argon atmosphere, triethylamine (0.53 mL, 3.80 mmol) was added to dichloromethane (20 mL), followed by N-methyl-4-piperidinethiol (0.45 mL, 3.80 mmol) and 4-fluorobenzoyl chloride (0.18 mL, 3.80 mmol). The reaction mixture was stirred for 4 d at reflux temperature. The reaction was extracted with saturated NaHCO3 (3 × 20 mL) and the organic layer dried over Na2SO4. The solvent was removed in vacuo to yield a light yellow solid which was purified by silica gel chromatography (7% MeOH in CH2Cl2) and recrystallized from hexanes to afford pale yellow crystals (0.11 g, 28%). Analytical data: MP (hexanes): 66–68°C. IR (Nujol): 1655, 1225, 1202, 1155, 916, 843 cm-1. 1H NMR (CDCl3): δ 1.88–1.95 (m, 2H), 2.11–2.20 (m, 2H), 2.41 (s, 3H), 2.81–2.94 (m, 2H), 3.69–3.81 (m, 1H), 7.10–7.15 (m, 2H), 7.95–7.99 (m, 2H). 13C NMR (CDCl3): δ 32.5 (2), 40.2 (1), 46.6 (3), 55.5 (2), 116.2 (JCF = 22 Hz, 1), 130.2 (JCF = 9 Hz, 1), 134.0 (0), 166.4 (JCF = 255 Hz, 0), 190.4 (0). EI-MS m/z: 253 (M+, 3%), 130 (39%), 123 (24%), 98 (82%), 97 (100%), 96 (36%), 95 (24%), 82 (10%), 75 (10%), 70 (17%), 55 (21%), 42 (17%). HRMS (ESI): MH+ found 254.1009, calcd for C13H17FNOS+ = 254.1009.
Synthesis of (N-methylpiperidin-4-yl) 4-iodobenzenecarbothioate (6)
Under an argon atmosphere, triethylamine (0.53 mL, 3.80 mmol) was added to dichloromethane (20 mL), followed by N-methyl-4-piperidinethiol (0.45 mL, 3.80 mmol) and 4-iodobenzoyl chloride (1.01 g, 3.80 mmol). The reaction mixture was stirred for 24 h at reflux temperature. The reaction was extracted with saturated NaHCO3 (3 × 20 mL) and the organic layer dried over Na2SO4. The solvent was removed in vacuo to yield a light yellow solid which was recrystallized from hexanes to afford pale yellow crystals (0.41 g, 30%). Analytical data: MP (hexanes): 136–137°C. IR (Nujol): 1650, 1208, 824 cm-1. 1H NMR (CDCl3): δ 1.80–1.87 (m, 2H), 2.09–2.14 (m, 2H), 2.25–2.32 (m, 2H), 2.34 (s, 3H), 2.74–2.83 (m, 2H), 3.67–3.78 (m, 1H), 7.64–7.67 (m, 2H), 7.79–7.82 (m, 2H). 13C NMR (CDCl3): δ 31.9 (2), 39.6 (1), 46.0 (3), 54.9 (2), 100.8 (0), 128.2 (1), 136.2 (0), 137.6 (1), 190.6 (0). EI-MS m/z: 361 (M+, 1%), 230 (12%), 130 (30%), 98 (60%), 97 (100%), 96 (22%), 76 (14%), 70 (10%), 55 (12%), 42 (10%). HRMS (ESI): MH+ found 362.0070, calcd for C13H17INOS+ = 362.0070.
Synthesis of (N-methylpiperidin-4-yl) ethanethioate (7)
N-Methyl-4-piperidinethiol (0.5 mL, 4.2 mmol) was dissolved in dry CH2Cl2 (5 mL) under an argon atmosphere. To this was added acetyl chloride (0.90 mL, 13 mmol) and the reaction refluxed for 1.5 h. The solvent was removed in vacuo and the resulting oil dissolved in saturated NaHCO3 (20 mL). This solution was extracted with CH2Cl2 (4 × 20 mL), dried over Na2SO4 and the solvent removed in vacuo to produce a clear colourless oil. This oil was purified by silica gel column chromatography (1:19 MeOH/CH2Cl2) to produce a clear colourless viscous liquid (0.69 g, 96%). Analytical Data: IR (Neat): 2941, 1686, 1448, 1219, 915 cm-1. 1H NMR (CDCl3): δ 1.58–1.65 (m, 2H), 1.88–1.92 (m, 2H), 2.08–2.18 (m, 2H), 2.21 (s, 3H), 2.24 (s, 3H), 2.59–2.70 (m, 2H), 3.33–3.48 (m, 1H). 13C NMR (CDCl3): δ 30.6 (3), 31.8 (2), 39.3 (1), 46.0 (3), 54.9 (2), 195.2 (0). EI-MS m/z: 173(M+, 17%), 130 (52%), 98 (100%), 97 (30%), 96 (33%), 70 (26%), 55 (45%). HRMS (ESI): MH+ found 174.0947, calcd for C8H16NOS+ = 174.0947.
Synthesis of (N-methylpiperidin-4-yl) propanethioate (8)
N-Methyl-4-piperidinethiol (0.5 mL, 4.2 mmol) was dissolved in dry CH2Cl2 (5 mL) under an argon atmosphere. To this was added propionyl chloride (0.94 mL, 11 mmol) and the reaction refluxed for 2 h. The solvent was removed in vacuo and the resulting oil dissolved in saturated NaHCO3 (20 mL). This solution was extracted with CH2Cl2 (4 × 20 mL), dried over Na2SO4 and the solvent removed in vacuo to produce a clear colourless viscous liquid. This oil was purified by silica gel column chromatography (1:19 MeOH/CH2Cl2) to produce a clear colourless viscous liquid (0.45 g, 57%). Analytical data: IR (Neat): 2939, 2782, 1689, 1463, 1129, 937 cm-1. 1H NMR (CDCl3): δ 1.19 (t, J = 7.5, 3H), 1.65-1.72 (m, 2H), 1.95–1.98 (m, 2H), 2.13–2.25 (m, 2H), 2.28 (s, 3H), 2.55 (q, J = 7.5, 2H), 2.63–2.83 (m, 2H), 3.41–3.54 (m, 1H). 13C NMR (CDCl3): δ 9.6 (3), 32.1 (2), 37.5 (2), 39.1 (1), 46.2 (3), 55.1 (2), 199.8 (0). EI-MS m/z: 187 (M+, 17%), 130 (61%), 98 (100%), 97 (27%), 96 (28%), 70 (17%), 55 (20%). HRMS (ESI): MH+ found 188.1104, calcd for C9H18NOS+ = 188.1104
Synthesis (N-methylpiperidin-4-yl) butanethioate (9)
N-Methyl-4-piperidinethiol (0.5 mL, 4.2 mmol) was dissolved in dry CH2Cl2 (5 mL) under an argon atmosphere. To this was added butyryl chloride (1.3 mL, 13 mmol) and the reaction refluxed for 1.5 h. The solvent was removed in vacuo and the resulting oil dissolved in saturated NaHCO3 (20 mL). This solution was extracted with CH2Cl2 (4 × 20 mL), dried over Na2SO4 and the solvent removed in vacuo to produce a clear colourless viscous liquid. This oil was purified by silica gel column chromatography (1:19 MeOH/ CH2Cl2) to produce a clear colourless oil (0.56 g, 67%). Analytical data: IR (Neat): 2938, 2782, 1686, 1447, 1129, 994 cm-1. 1H NMR (CDCl3): δ 0.95 (t, J = 7.3, 3H), 1.62-1.71 (m, 4H), 1.89–2.01 (m, 2H), 2.08–2.22 (m, 2H), 2.25 (s, 3H), 2.49 (t, J = 7.3, 2H), 2.59–2.81 (m, 2H), 3.38–3.53 (m, 1H). 13C NMR (CDCl3): δ 13.3 (3), 19.0 (2), 32.1 (2), 39.1 (1), 45.9 (2), 46.20 (3), 55.1 (2), 198.9 (0). EI-MS m/z: 201 (M+, 16%), 130 (65%), 98 (100%), 97 (28%), 96 (26%), 70 (17%), 55 (20%). HRMS (ESI): MH+ found 202.1260, calcd for C10H20NOS+ = 202.1260.
Enzyme kinetics
Measurement of aryl ester hydrolysis
Cholinesterase specificity and maximum absorbance change during hydrolysis for each benzoyl ester was determined spectrophotometrically, making use of differences in the aryl chromophores of substrates and products determined through repetitive absorbance scans (e.g. ). Briefly, 15 μL of AChE (2.5 U) or BuChE (5.4 U) dissolved in 0.1% gelatin(aq), containing 0.01% sodium azide, and 1.44 mL of 0.1 M phosphate buffer (pH 7.4) were placed in a quartz cuvette of 1-cm path length. The reaction was commenced with the addition of 50 μL of 5 mM ester in 50% acetonitrile(aq). The absorbance was scanned from 200–300 nm every 2 min for a total of 30 min using a Ultrospec 2100 pro UV/Visible Spectrophotometer (Biochrom) with Swift II software (Amersham). The wavelength corresponding to the maximum absorbance change during hydrolysis (235 nm for compounds 1-3) was used for the subsequent determination of BuChE affinity constants (Km values, ) using Lineweaver-Burk double reciprocal plots.
Table 1. Affinity constants (Km) and maximum velocity values (Vmax) for N-methylpiperidinyl alkyl thioesters, N-methylpiperidinyl aryl esters and corresponding thioesters with butyrylcholinesterase (BuChE) and acetylcholinesterase (AChE). None of the aryl compounds (1–6) were hydrolyzed by AChE under the conditions used (X).
Figure 1. Repetitive absorbance scans for N-methylpiperidin-4-yl 4-cyanobenzoate in the presence of butyrylcholinesterase (BuChE) or acetylcholinesterase (AChE). Note change in absorbance over time when the compound was incubated with BuChE (left) reflecting hydrolysis of the compound by this enzyme. No change in absorbance with AChE (right) indicates no hydrolysis by this enzyme. The absorbance was measured every 2 min for 30 min.
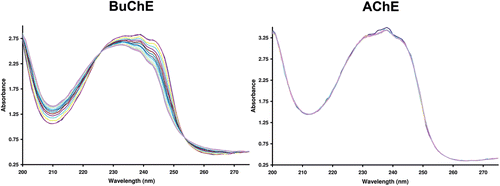
Determination of affinity constants (Km) and maximum velocity values (Vmax) was accomplished by measuring change in absorbance per min (ΔA/min) at 235 nm, using a fixed amount of enzyme AChE (8.3 U) or BuChE (5.9 U) and varying amounts of compounds (1.67 × 10−4− 1.67 × 10−5 M), using a Spectronic 1001 (Milton Roy) UV-visible spectrophotometer. The plot of 1/v against 1/s gave Km as the negative reciprocal of the intercept on the 1/s-axis and gave Vmax as the reciprocal of the intercept of the 1/v-axis. As defined previouslyCitation31, 0.1 U is the amount of cholinesterase that gives a ΔA/min of 1.0 in the presence of 1.6 × 10−4 M substrate (acetylthiocholine for AChE, butyrylthiocholine for BuChE).
Measurement of thioester hydrolysis
The kinetics of both alkyl and aryl thioester hydrolysis by AChE or BuChE was determined using a modificationCitation27 of the method described by Ellman et al.Citation26. Briefly, 1.40 mL of buffered 5, 5′-dithio-bis(2-nitrobenzoic acid) solution (pH 7.4) and 0.05 mL of AChE (8.3 units) or BuChE (5.9 units), in 0.1% aqueous gelatin, were mixed in a quartz cuvette of 1-cm path-length and zeroed at 412 nm. The reaction was initiated by the addition of thioester in a 50% aqueous acetonitrile solution to give a final substrate concentration from 1.7 × 10−4 to 3.3 × 10−6 M. The reactions were performed at 23˚C. The rate of change of absorbance (ΔA/min), reflecting the rate of hydrolysis of the thioester, was recorded every 5 s for 1 min, using a Milton-Roy 1201 UV–vis spectrophotometer. The molar extinction coefficient for the Ellman product, 5-thio-2-nitrobenzoic acid, used to convert the change in absorbance at λ = 412 nm to moles of product was 14,150 M−1 cm−1. These experiments were performed at least in triplicate and the values averaged.
Structural analysis
Computational chemistry studies, to determine the most stable geometries of compounds, were carried out at the molecular mechanics level of theory using the Merck Molecular Force Field (MMFF), employing Spartan’0632.
Cholinesterase histochemistry with thioester substrates
Brain tissue containing the thalamus was cut on a Leica SM2000R microtome with a Physitemp freezing stage in 50 μm thick coronal sections. Sections were stored in 40% sucrose in 0.1 M phosphate buffer (pH 7.4) at −20˚C until used. A modifiedCitation33 Karnovsky-Roots method was employed for AChE and BuChE staining. Substrates used to provide staining were synthesized thioesters and the standards, butyrylthiocholine and acetylthiocholine in the absence of cholinesterase inhibitors. Briefly, tissue sections were rinsed in 0.1 M maleate buffer (pH 7.4) for 30 min and then placed in 0.1 M maleate buffer, pH 7.4, containing 0.15% hydrogen peroxide for 30 min to quench endogenous peroxidase activity. Following a second rinse in 0.1 M maleate buffer, pH 7.4, for 30 min, sections were incubated for 1 h (acetylthiocholine, [N-methylpiperidin-4-yl] ethanethioate (7)) or 18 h (butyrylthiocholine, [N-methylpiperidin-4-yl] butanethioate (9) and [N-methylpiperidin-4-yl] 4-cyanobenzenecarbothioate (4)) in a medium containing 0.5 mM sodium citrate, 0.47 mM cupric sulfate, 0.05 mM potassium ferricyanide and thioester substrate in 0.1 M maleate buffer (pH 7.4). Substrate concentrations were 4 mM for acetylthiocholine, butyrylthiocholine, (N-methylpiperidin-4-yl) ethanethioate, (N-methylpiperidin-4-yl) butanethioate and 0.5 mM for the less soluble (N-methylpiperidin-4-yl) 4-cyanobenzenecarbothioate. Sections were then rinsed for 30 min in distilled water and placed in 0.1% cobalt (II) chloride in water for 10 min. After a further rinse in distilled water, sections were placed in a 3, 3′-diaminobenzidine tetrahydrochloride (DAB) solution consisting of 1.39 mM in 0.1M phosphate buffer (pH 7.4). After 5 min, a solution of 0.15% hydrogen peroxide in distilled water was added at a ratio of 10:1 (DAB solution: hydrogen peroxide solution) and the reaction was carried out for approximately 2 min. Sections were then washed in 0.01 M acetate buffer, pH 3.3, mounted on slides, coverslipped and examined with brightfield microscopy.
Sections were photographed with an AxioCam HRc camera on a Zeiss Axioplan II microscope. The photographic plates were assembled using Adobe Photoshop 7.0. The images were colour balanced, contrast enhanced and brightness adjusted to match the background from different images.
Results and discussion
Organic synthesis
To synthesize N-methyl-4-piperidinethiol, N-methyl-4-piperidone was mixed with hydrogen sulfide and the resulting reaction produced a dithiol. This intermediate was then reduced with sodium borohydride (), in a reaction adapted from an earlier procedureCitation34. Thioesters were subsequently generated by esterification of this thiol with an acid chloride (). Benzoate esters were also synthesized, as previously describedCitation24, (, , 1–3) in order to directly compare cholinesterase kinetic parameters for aryl thioesters and esters. Two classes of thioesters were synthesized, those from aryl acid chlorides (, 4–6), analogous to the benzoate esters, and those from alkyl acid chlorides (, 7–9), analogous to previously developed alkyl ester imaging agentsCitation17,Citation22.
Kinetic analysis
Thioester analogues of aryl esters such as benzoylcholine have been foundCitation28 to be kinetically comparable to their ester counterparts for cholinesterase hydrolysis. Therefore, thioesters can provide a rapid, indirect enzyme kinetic screening method for analysis of potential ester imaging agents. All aryl and alkyl thioesters, producing a thiol product, upon hydrolysis, are amenable to kinetic analysis as cholinesterase substrates via an Ellman assay. Furthermore, since all aryl esters showed distinct UV spectral differences between reactant and product (), they could be analyzed for comparison with thioester kinetic parameters by an alternate method employing changes in absorbance. Esters and thioesters of substituted benzoic acids gave comparable affinity constants and maximum velocity values (), in agreement with the earlier observation comparing benzoylcholine and benzoylthiocholine analoguesCitation28. In general, the thioester derivatives had a slightly greater affinity towards BuChE than did the esters. In addition, none of the aryl thioesters, as observed earlier for aryl estersCitation24, were susceptible to hydrolysis by AChE ().
Although it was not possible to directly obtain affinity constants for the esters of N-methylpiperidinol derived from acetic acid, propionic acid and butyric acid, it is reasonable to expect, from comparable studies with aryl derivatives (Citation28), these values to be similar to those for the comparable thioesters (). This is supported by the observation that for AChE, like the analogous thiocholine esters, the acetyl ester of N-methyl-4-piperidinethiol (7, ) had the greatest affinity, followed by the propionyl ester (8), while the butyryl derivative (9) was not hydrolyzed by AChE under these conditions. In addition, BuChE affinity increased with longer substrate side chains, consistent with the known preference of this enzyme for longer acyl chainsCitation27,Citation35. The observation that the thioester analogue of MP4B is BuChE-specific is also in keeping with the earlier reportCitation25 that the ester is only hydrolyzed by BuChE. Of note is that the affinities of the acetyl (7) and propionyl (8) thioesters for BuChE are greater than for AChE. Even though the corresponding radiolabelled esters, 4-[11C]AMP and 4-[11C]PMP, have been developed as AChE imaging agents, these kinetic results suggest that BuChE is also capable of efficiently hydrolyzing these compounds and thus, both AChE and BuChE may be detected in molecular imaging studies with these agents, rendering them to be non-specific.
Structural analysis
The preferred geometries of the synthetic thioesters and their ester counterparts were computed at the molecular mechanics level in order to further explore any subtle differences observed in the cholinesterase kinetic affinities. In general, the preferred conformations for each oxygen and sulfur analogous pair were comparable (Figure S1). However, slight differences in molecular shape, because of the presence of the bulkier and electronically distinct sulfur atom, most likely contributed to observed differences in relative affinity constants for BuChE () in each analogous pair. The structural similarity further suggests that thioesters are acceptable kinetic surrogates for corresponding ester imaging agents.
Histochemical evaluation of N-methyl piperidinyl thioesters
Distribution of an imaging agent within the living brain is dependent upon a number of factors. These include cerebral blood flow, penetration of the blood–brain barrier, interaction with the target molecules and fate of the metabolites. Thus, determining the in vitro distribution of the imaging agent in brain tissues would be valuable before advancing to in vivo radioimaging studies. The cholinesterases are commonly stained in brain tissues using thioesters such as butyrylthiocholine or acetylthiocholineCitation29,Citation36. In order to explore cholinesterase specificity and to determine their potential to detect the distribution of cholinesterases in the brain, several of the thioesters prepared in this study were tested as histochemical substrates and compared with choline thioesters for staining human thalamus brain tissue (). This region of the brain was chosen because it has been precisely mapped for cholinesterase activity and certain nuclei, such as the anteroventral (AV) nucleus (), exhibit distinct patterns of AChE and BuChE activityCitation36. In the AV nucleus of the thalamus, AChE activity is found only in the neuropil while BuChE activity is observed only in neurons. As can be seen in , acetylthiocholine () and N-methylpiperidinyl acetylthioester (7; ; ) show comparable neuropil staining typical of AChE activity in this nucleus. That is, both the choline and N-methylpiperidinethiol acetyl thioester substrates provided histochemical staining consistent with the previously described distribution of AChE in this region of the brainCitation36. This observation is also in agreement with the in vitro enzyme kinetic results indicating that AChE is capable of efficiently hydrolyzing the N-methylpiperidinyl acetylthioester (). N-methylpiperidinyl acetylthioester (7) is hydrolyzed by both AChE and BuChE (), similar to acetylthiocholineCitation37, using Ellman method. However, the modified Karnovsky-Roots method for histochemical staining requires shorter (1 h) incubation for staining AChE and longer (18 h) for BuChE. Based on this difference in the incubation time, staining in is predominantly for AChE. Similarly, to evaluate specific histochemical visualization of BuChE activity in the human thalamus, comparative staining with butyrylthiocholine, N-methylpiperidinyl butyrylthioester (9; ) and cyanobenzoate thioester (4; ) was carried out (, , ). BuChE staining using butyrylthiocholine as substrate () was comparable to that obtained with the butyryl () and 4-cyanobenzoyl () derivatives of N-methylpiperidinethiol. Furthermore, the BuChE staining profiles (, , ) were distinct from those for AChE observed with the acetyl thioesters ( and ) in the same region of the brain. High (>1 mM) thioester concentrations are typically required in the staining medium to achieve adequate visualization of cholinesterase activity. Because of this, solubility limitations prohibited use of the iodo- and fluorobenzoate thioesters in similar histochemical analysis to that provided by the cyanobenzoate derivative (). However, successful histochemical recapitulation of enzyme activity with an aryl thioester provides a critical proof-of-principle that analogous radiolabelled compounds are potential imaging agents to be highly sensitive detectors of cholinesterase activity in brain tissue.
Figure 2. Histochemical staining of human brain tissue at the level of the thalamus in the coronal plane. (A) Parcellation of the thalamus in the region used to compare histochemical staining by various cholinesterase substrates; (B) Acetylthiocholine. (C) (N-methylpiperidin-4-yl) ethanethioate. Note that both of these substrates produced a similar pattern of staining recapitulating the known distribution of acetylcholinesterase in this region. (D) Butyrylthiocholine. (E) (N-methylpiperidin-4-yl) butanethioate. (F) (N-methylpiperidin-4-yl) 4-cyanobenzenecarbothioate. Note that these substrates produced a similar pattern of distribution that reflected the known distribution of butyrylcholinesterase in this region. AV: anteroventral nucleus; CL: central lateral nucleus; MD: mediodorsal nucleus; R: reticular nucleus; V: ventral nuclei. Scale bar = 200 µm.
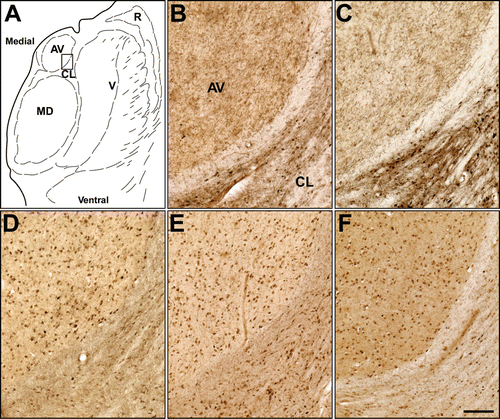
Conclusion
This study indicates that N-methylpiperidinyl thioesters are effective surrogate substrates for the evaluation and development of corresponding ester compounds as AChE and BuChE imaging agents. These thioester analogues have comparable affinities towards AChE and BuChE to their ester counterparts. These similarities permit screening of corresponding ester substrates as potential radioligands for specific detection of AChE or BuChE activity. These thioester compounds can also be employed for histochemical detection of enzyme activity in brain tissue, which could then be used for comparison with the in vivo images generated by the analogous radiolabelled molecules. Development of compounds, screened in this manner, could facilitate discovery of suitable molecular imaging agents for the detection of AChE and BuChE in the living brain for application to neurological disorders involving the cholinergic system, such as AD.
Supplementary Material
Download PDF (216.2 KB)Acknowledgements
The authors thank Eric Joy for technical assistance.
Declaration of interest
Portions of this work were supported by grants from Canadian Institutes of Health Research, Canada Foundation for Innovation, Natural Sciences and Engineering Research Council of Canada, Vascular Health and Dementia Initiative (through Canadian Institutes of Health Research, Heart & Stroke Foundation of Canada, the Alzheimer Society of Canada and Pfizer Canada Inc.), Capital District Health Authority Research Fund, Nova Scotia Health Research Foundation, Brain Tumour Foundation of Canada, Multiple Sclerosis Society of Canada, National Institutes of Health, Dalhousie Medical Research Foundation, Faculty of Medicine of Dalhousie University and the Committee on Research and Publications of Mount Saint Vincent University.
References
- Davies P, Maloney AJ. Selective loss of central cholinergic neurons in Alzheimer’s disease. Lancet 1976;2:1403.
- Bartus RT,Dean RL 3rd, Beer B, Lippa AS. The cholinergic hypothesis of geriatric memory dysfunction. Science 1982;217:408–414.
- Whitehouse PJ, Price DL, Struble RG, Clark AW, Coyle JT, Delon MR. Alzheimer’s disease and senile dementia: loss of neurons in the basal forebrain. Science 1982;215:1237–1239.
- Nizri E, Hamra-Amitay Y, Sicsic C, Lavon I, Brenner T. Anti-inflammatory properties of cholinergic up-regulation: A new role for acetylcholinesterase inhibitors. Neuropharmacology 2006;50:540–547.
- Darvesh S, Leblanc AM, Macdonald IR, Reid GA, Bhan V, Macaulay RJ et al. Butyrylcholinesterase activity in multiple sclerosis neuropathology. Chem Biol Interact 2010;187:425–431.
- Barbosa M, Rios O, Velásquez M, Villalobos J, Ehrmanns J. Acetylcholinesterase and butyrylcholinesterase histochemical activities and tumor cell growth in several brain tumors. Surg Neurol 2001;55:106–112.
- Silver A. (1974). The biology of cholinesterases. Amsterdam, The Netherlands: Elsevier.
- Friede RL. Enzyme histochemical studies of senile plaques. J Neuropathol Exp Neurol 1965;24:477–491.
- Geula C, Mesulam M. Special properties of cholinesterases in the cerebral cortex of Alzheimer’s disease. Brain Res 1989;498:185–189.
- Carson KA, Geula C, Mesulam MM. Electron microscopic localization of cholinesterase activity in Alzheimer brain tissue. Brain Res 1991;540:204–208.
- Morán MA, Mufson EJ, Gómez-Ramos P. Colocalization of cholinesterases with beta amyloid protein in aged and Alzheimer’s brains. Acta Neuropathol 1993;85:362–369.
- Gómez-Ramos P, Bouras C, Morán MA. Ultrastructural localization of butyrylcholinesterase on neurofibrillary degeneration sites in the brains of aged and Alzheimer’s disease patients. Brain Res 1994;640:17–24.
- Mesulam MM, Geula C. Butyrylcholinesterase reactivity differentiates the amyloid plaques of aging from those of dementia. Ann Neurol 1994;36:722–727.
- Geula C, Mesulam MM. Cholinesterases and the pathology of Alzheimer disease. Alzheimer Dis Assoc Disord 1995;9 Suppl 2:23–28.
- Guillozet AL, Smiley JF, Mash DC, Mesulam MM. Butyrylcholinesterase in the life cycle of amyloid plaques. Ann Neurol 1997;42:909–918.
- Darvesh S, Reid GA, Martin E. Biochemical and histochemical comparison of cholinesterases in normal and Alzheimer brain tissues. Curr Alzheimer Res 2010;7:386–400.
- Irie T, Fukushi K, Akimoto Y, Tamagami H, Nozaki T. Design and evaluation of radioactive acetylcholine analogs for mapping brain acetylcholinesterase (AchE) in vivo. Nucl Med Biol 1994;21:801–808.
- Kilbourn MR, Snyder SE, Sherman PS, Kuhl DE. In vivo studies of acetylcholinesterase activity using a labeled substrate, N-[11C]methylpiperdin-4-yl propionate ([11C]PMP). Synapse 1996;22:123–131.
- Kilbourn MR, Nguyen TB, Snyder SE, Sherman P. N-[11C]methylpiperidine esters as acetylcholinesterase substrates: an in vivo structure-reactivity study. Nucl Med Biol 1998;25:755–760.
- Snyder SE, Tluczek L, Jewett DM, Nguyen TB, Kuhl DE, Kilbourn MR. Synthesis of 1-[11C]methylpiperidin-4-yl propionate ([11C]PMP) for in vivo measurements of acetylcholinesterase activity. Nucl Med Biol 1998;25:751–754.
- Irie T, Fukushi K, Namba H, Iyo M, Tamagami H, Nagatsuka S et al. Brain acetylcholinesterase activity: validation of a PET tracer in a rat model of Alzheimer’s disease. J Nucl Med 1996;37:649–655.
- Snyder SE, Gunupudi N, Sherman PS, Butch ER, Skaddan MB, Kilbourn MR et al. Radiolabeled cholinesterase substrates: in vitro methods for determining structure-activity relationships and identification of a positron emission tomography radiopharmaceutical for in vivo measurement of butyrylcholinesterase activity. J Cereb Blood Flow Metab 2001;21:132–143.
- Kuhl DE, Koeppe RA, Snyder SE, Minoshima S, Frey KA, Kilbourn MR. In vivo butyrylcholinesterase activity is not increased in Alzheimer’s disease synapses. Ann Neurol 2006;59:13–20.
- Macdonald IR, Reid GA, Joy EE, Pottie IR, Matte G, Burrell S, Mawko G, Martin E, Darvesh S. Synthesis and preliminary evaluation of piperidinyl and pyrrolidinyl iodobenzoates as imaging agents for butyrylcholinesterase. Mol Imaging Biol 2010. doi: 10.1007/s11307-010-0448-0.
- Kikuchi T, Fukushi K, Ikota N, Ueda T., Nagatsuka S., Arano Y, Irie T. Synthesis of piperidinyl and pyrrolidinyl butyrates for potential In Vivo measurement of cerebral butyrylcholinesterase activity. J Labelled Comp Rad 2001;44:31–41.
- Ellman GL, Courtney KD, Andres V Jr,Feather-Stone RM. A new and rapid colorimetric determination of acetylcholinesterase activity. Biochem Pharmacol 1961;7:88–95.
- Pottie IR, Higgins EA, Blackman RA, Macdonald IR, Martin E, Darvesh S. Cysteine thioesters as myelin proteolipid protein analogues to examine the role of butyrylcholinesterase in myelin decompaction. ACS Chem Neurosci 2011;2:151–159.
- Masson P, Froment MT, Gillon E, Nachon F, Lockridge O, Schopfer LM. Hydrolysis of oxo- and thio-esters by human butyrylcholinesterase. Biochim Biophys Acta 2007;1774:16–34.
- Karnovsky MJ, Roots L. A “Direct-coloring” thiocholine method for cholinesterases. J Histochem Cytochem 1964;12:219–221.
- Barrera H, Lyle RE. Piperidine derivatives with a sulfur-containing function in the 4-position. J Org Chem 1962;27:641–643.
- Darvesh S, Kumar R, Roberts S, Walsh R, Martin E. Butyrylcholinesterase-Mediated enhancement of the enzymatic activity of trypsin. Cell Mol Neurobiol 2001;21:285–296.
- Spartan’06 Program. (2006). Irvine, CA: Wavefunction Inc.
- Darvesh S, Grantham DL, Hopkins DA. Distribution of butyrylcholinesterase in the human amygdala and hippocampal formation. J Comp Neurol 1998;393:374–390.
- Meanwell NA, Hewawasam P, Thomas JA, Wright JJ, Russell JW, Gamberdella M et al. Inhibitors of blood platelet cAMP phosphodiesterase. 4. Structural variation of the side-chain terminus of water-soluble 1,3-dihydro-2H-imidazo[4,5-b]quinolin-2-one derivatives. J Med Chem 1993;36:3251–3264.
- Darvesh S, McDonald RS, Darvesh KV, Mataija D, Mothana S, Cook H et al. On the active site for hydrolysis of aryl amides and choline esters by human cholinesterases. Bioorg Med Chem 2006;14:4586–4599.
- Darvesh S, Hopkins DA. Differential distribution of butyrylcholinesterase and acetylcholinesterase in the human thalamus. J Comp Neurol 2003;463:25–43.
- Darvesh S, Walsh R, Kumar R, Caines A, Roberts S, Magee D et al. Inhibition of human cholinesterases by drugs used to treat Alzheimer disease. Alzheimer Dis Assoc Disord 2003;17:117–126.