Abstract
The highly similar aldehyde dehydrogenase isozymes (ALDH1A1 and ALDH2) have been implicated in the metabolism of toxic biogenic aldehydes such as 3,4-dihydroxyphenylacetaldehyde (DOPAL) and 4-hydroxy-2E-nonenal. We report the down-regulation of ALDH1A1 mRNA found in substantia nigra tissue of human Parkinson’s disease (PD) samples using the Genome-Wide SpliceArray™ (GWSA™) technology. Since DOPAL can rapidly inactivate ALDH1A1 in vitro, we set up a DOPAL-induced ALDH1A1 inactivation assay and used this assay to demonstrate that Alda-1, a compound originally identified as an activator of ALDH2, can also activate ALDH1A1. We carried out a virtual screening of 19,943 compounds and the top 21 hits from this screen were tested in the DOPAL inactivation assay with ALDH1A1 which led to identification of an activator as well as two inhibitors among these hits. These findings represent an attractive starting point for developing higher potency activator compounds that may have utility in restoring the metabolism of DOPAL in PD.
Introduction
The aldehyde dehydrogenase (ALDH) superfamily includes nicotinamide adenine dinucleotide phosphate (NAD+)-dependent enzymes that catalyze the oxidation of a wide spectrum of aldehydes to their corresponding carboxylic acidsCitation1. Endogenous aldehydes are formed during metabolism of alcohols, amino acids, biogenic amines, vitamins, steroids, and lipids. Two such endogenous biogenic aldehydes are 3,4-dihydroxyphenylacetaldehyde (DOPAL) and (±)-4-hydroxy-2E-nonenal (4-HNE).
Cytosolic aldehyde dehydrogenase including ALDH1 has been implicated in the metabolism of both DOPAL and 4-HNE. DOPAL is formed from the deamination of cytoplasmic dopamine by monoamine oxidase A in catecholaminergic neuronsCitation2. The metabolism of DOPAL to 3,4-Dihydroxyphenylacetic acid (DOPAC) in human brain has been reported to be mediated primarily by cytosolic aldehyde dehydrogenaseCitation3. The ALDH of retinal pigmented epithelial cells mediates one of three pathways of detoxification of 4-HNE by converting it to 4-hydroxy-2-nonenoic acidCitation4. Recently, it has been shown that overexpression of ALDH1A1 reduces 4-HNE induced toxicity supporting a role for this enzyme in 4HNE detoxification mechanisms in SH-SY5Y neuroblastoma cellsCitation5
A few independent studies have reported the down-regulation of ALDH1A1 mRNA and protein in Parkinson’s disease (PD) tissuesCitation6–8. In addition to regulation of ALDH gene expression in disease states such as PD, there is in vitro evidence indicating that ALDH activity is regulated by their endogenous substrates. For example, DOPAL-induced inactivation of ALDH1 and 4-HNE induced inactivation of ALDH2, the mitochondrial isoenzyme, have been reportedCitation9,Citation10.
It is thus conceivable that down-regulation of ALDH1A1 mRNA and protein in PD in conjunction with DOPAL-induced inactivation of its enzymatic activity would lead to accumulation of DOPAL. Hence, ALDH1A1 activators that can enhance the clearance of toxic DOPAL would be of interest in PD. In support of this idea, DOPAL accumulation has been shown to be toxic in rats. DOPAL injection into the substantia nigra of rats resulted in dopaminergic neuron loss and the accumulation of high molecular weight oligomers of α-synuclein, a feature of PDCitation11
The isozymes, ALDH1A1 and ALDH2, are 68% identical at the amino acid level and both form heterotetramers suggesting a similar three-dimensional structureCitation12. A small molecule activator of ALDH2, Alda-1 (), was identified in a high-throughput screen, and, more recently, the crystal structure of Alda-1 bound to ALDH2 has been publishedCitation10,Citation13.
Figure 1. Structures of Alda-1, activator (1) and inhibitors (2) and (3) and their EC50 values in the DOPAL inactivation assay. Compounds (1–3) were among the 21 hits identified in the virtual screening.
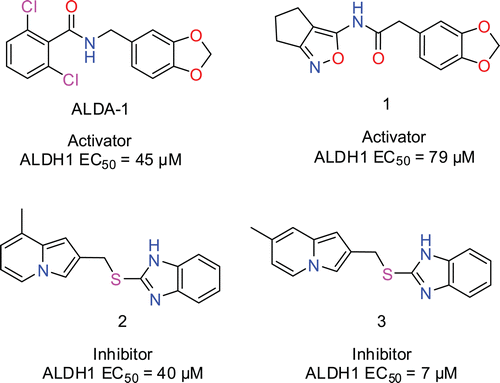
In this report, we confirm the down-regulation of ALDH1A1 mRNA in PD tissues and report the activity of Alda-1 in an in vitro ALDH1A1 activity assay. In addition, we present novel modulators of ALDH1A1 that were identified among the hits from a virtual screening approach that was based on the structure of the Alda-1 binding site in ALDH2.
Methods
Genome-Wide SpliceArray™ experiment and analysis
Total RNA from substantia nigra tissue of 4 control and 4 PD patients were hybridized on the human Genome-Wide SpliceArray™ (GWSA™). The human GWSA™ allows the detection of alternatively spliced events within the human transcriptome through the use of exon body and exon junction probes to provide a direct measure of each transcript as well as changes in overall gene expression between two samplesCitation14. The array and data analysis procedures for identifying differentially expressed probes and genes between the control and PD samples were done as previously describedCitation14.
Recombinant protein
The ALDH1A1 cDNA was amplified from pCMV6-XL5-ALDH1A1 (Origene; SC119729) with the primers ALDH1A1-F (TATTTAGCTAGCATGTCATCCTCAGGCACGCCAGA) and ALDH1A1-R (GCGGAATTCCTATGAGTTCTTCTGAGAGATTTTCACTGTG). The resulting 1527 bp PCR amplicon was digested with Nhe1 and EcoR1 and inserted into Nhe1—EcoR1 cut pTrcHis B vector (Invitrogen). The resulting N-terminal six Histidine tagged protein was induced in E.coli and purified under nondenaturing conditions by nickel-NTA affinity chromatography using the His-Bind Purification Kit (Novagen; 70239-3) according to the manufacturer’s instructions. The eluted fraction containing ALDH1A1 was dialyzed against 50mM Tris-HCl pH 8 at 4°C overnight and stored as aliquots at −80°C.
DOPAL inactivation assay
DOPAL was synthesized as described in supplementary material. A 100 mM solution of DOPAL in DMSO was prepared and stored as aliquots at −80°C until use. Recombinant ALDH1A1 protein (60 nM) was preincubated with various DOPAL concentrations or DMSO in 50 mM HEPES pH 7.5; 0.005% Tween-20; 12.5mM Tris-HCl pH7.5; 12.5 mM Tris-HCl pH 8 for 20 min. The reaction was initiated by the addition of a mixture of NAD+ and propanal to a final concentration of 1 mM each. The increase in fluorescence (λexcitation = 355 nm/ λemission = 460 nm) due to formation of NADH was measured 12 times with an interval of 2 min between reads. The initial reading was subtracted from every subsequent reading to obtain the background-subtracted readings. Background-subtracted readings were plotted against the reading number to compare the progression of the DOPAL preincubated enzyme reaction with that of the corresponding DMSO control.
Virtual screening: ligand preparation and molecular docking
A list of 19,943 molecules available for immediate delivery in the Life Chemical fragment library that contains molecules with a molecular weight lower than 300 g/mole and a cLogP lower than 3 was chosen. The selection of this initial pool of compounds was made with the idea that it could lead to the identification of molecules that would be used as a starting point of a hit to lead medicinal chemistry program. The list of molecules depicted as 2D structures in the sdf format was imported into OpenBabel 2.2.3 and a quick local optimization was carried out with 50 steps using the MMFF94 force field to obtain a list of 19,943 molecules possessing atomic 3D coordinates with acceptable bond lengths generating a single ligand_3D.sdf file. The ligand_3D.sdf file was used as the data source in the program Molegro Virtual Docker 4.0.0 (MVD)Citation15 where assignment of bonds, bond order/hybridization, Tripos atom type, and creation of explicit hydrogen was performed only if missing. The assignment of charges was calculated by MVD and the docking wizard defined the detection of flexible torsions in ligands systematically. The binding site was defined with a 10-Å sphere centered on the carbon atom of the carbonyl function of the ligand ALDA-1 in the ALDA-1/ALDH2 complex (PDB code: 3INL) obtained from the PDB databaseCitation13. A rigid receptor/flexible ligand protocol was used in the docking process. The MolDock score with a grid resolution of 0.30 Å was used as the docking scoring function using 1 run of iterated simplex as the search algorithm with a maximum of 2,000 steps to return one pose. All the other parameters were used using their default value. The docking was executed using the Virtual Grid Module installed on a Dell 64 bit Server with Red Hat Linux using 16 processors and 16 GB of RAM. The 140 best MolDock scored molecules were ordered from low to high energy using the reranking score function, filtered to keep only molecules with less than five rotatable bounds. From this set, 21 molecules were purchased from Life Chemicals and are referred to as hits from the virtual screening described above.
Compound testing
Alda-1 was obtained from TimTec catalog # ST085712 and 2-(benzo[d][1,3]dioxol-5-yl)-N-(5,6-dihydro-4H-cyclopenta[c]isoxazol-3-yl)acetamide 1 catalog # F2413-0181, 2-((8-methylindolizin-2-yl)methylthio)-1H-benzo[d]imidazole 2 catalog # F0318-0070, 2-((7-methylindolizin-2-yl)methylthio)-1H-benzo[d]imidazole 3 F1754-0048 were obtained from Life Chemicals. Alda-1 and the 21 hits were dissolved in DMSO. The stock concentrations ranged from 13.3 mM to 20.0 mM depending on their solubility. The compounds were serially diluted 3-fold and tested in the enzyme assay at 10 concentrations over a 4-log scale. The final concentration of DMSO in the reactions was 2%. Recombinant ALDH1A1 protein was preincubated with 0.0125 mM DOPAL in the presence of various concentrations of Alda-1 or the screening hits in 50mM HEPES pH7.5 0.005% Tween-20; 12.5 mM Tris-HCl pH7.5; 12.5 mM Tris-HCl pH8 for 20 min. The reaction was initiated by the addition of a mixture of NAD+ and propanal to a final concentration of 1mM each. The increase in fluorescence (λexcitation = 355 nm/ λemission = 460 nm) due to formation of NADH was measured 12 times with an interval of 2 min between reads. The initial and the final readings were used to calculate percent Activity. The solvent control reaction in the equation below is the 2% DMSO reaction and represents the 0.0125 mM DOPAL-inactivated enzyme. The effect of the compounds is determined relative to the solvent control reaction as shown below.
BSR (background subtracted reading) = final reading− initial reading.
Some % activity scenarios are as follows:
0% activity = no effect of compound on DOPAL inactivation of enzyme
Positive 100% = 2x increase in activity over DOPAL inactivated enzyme
Negative 50% = 2x decrease in activity over DOPAL inactivated enzyme
The % activity values were plotted against compound concentrations and activation or inhibition EC50 values were determined by fitting the concentration-% activity data to four-parameter sigmoidal dose–response curves (GraphPad Prism 5.04, GraphPad Software Inc.).
Results and discussion
We carried out whole transcriptome profiling on the GWSA™ to identify genes that are differentially expressed in PD samples and found that expression of the ALDH1A1 gene was down-regulated 4-fold (p value = 0.00125) in substantia nigra tissue of PD patients. We went on to examine the individual fold change values of 48 differentially expressed probes (p value < 0.01) on the GWSA™ that cover the ALDH1A1 transcript (NCBI RefSeq Database ID NM_000689) from the 5′ end to the 3′ end and found that they were all down-regulated to a similar extent (about 3-fold) as at the gene level. Both exon body probes and exon junction probes were represented among these 48 probes (). Thus, the fold changes at the probe-level ruled out any PD-specific deregulation of alternative splicing in the coding region of ALDH1A1 transcript and provided greater confidence in the overall down-regulation of this entire transcript. In fact, our observation of ALDH1A1 down-regulation is consistent with previous observations that showed that ALDH1A1 is down-regulated 3-fold in a gene expression study of substantia nigra tissues from 6 Parkinsonian patients and 6 age-matched controlsCitation6. In another study, cytosolic aldehyde dehydrogenase mRNA levels were found to be lower in substantia nigra in PD (n = 13) compared with controls (n = 14) using in situ hybridizationCitation7. In addition, a proteomic analysis of human substantia nigra in PD, revealed that ALDH1 was among the proteins whose level was lower in PD substantia nigraCitation8. These consistent observations make it likely that ALDH1 activity is decreased in PD tissues which could lead to accumulation of DOPAL, a toxic substrate. Hence we formulated a ALDH1A1 activation hypothesis () that is of interest for the treatment of PD.
Figure 2. Generic workflow from PD tissue to the discovery of small molecule modulators of ALDH1. (a) Expression profiling of PD tissues using the Genome-Wide SpliceArray™ led to identification of ALDH1A1 down-regulation together with published literature and pathway analysis formed the basis for the ALDH1 activation hypothesis. Values in parenthesis represent the number of each probe type monitoring the gene transcript deregulation. For the letter code definition of each probe type see supplementary material. (b) Virtual screening workflow from structure to hits. A hit from the virtual screening was validated as an activator in vitro and could serve as starting point for hit to lead medicinal chemistry optimization program.
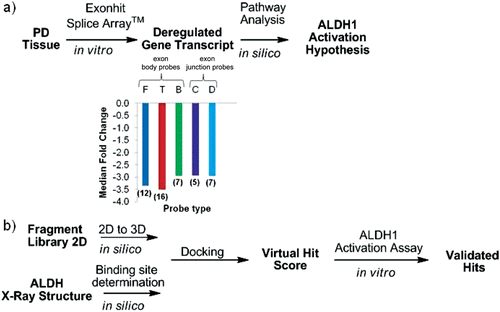
To identify activators that can enhance the clearance of toxic DOPAL, we decided to implement a DOPAL based in vitro assay using rALDH1A1. DOPAL has been shown to rapidly inactivate both cytoplasmic and mitochondrial isozymes of ALDH that were purified from human liverCitation9. So we first tested whether recombinant histidine-tagged ALDH1A1 (rALDH1A1) can also be inactivated by DOPAL. In this experiment, rALDH1A1 was preincubated with DOPAL for 20 min and residual activity was measured by adding an excess of NAD+ and propanal. As shown in , preincubation with DOPAL led to a concentration-dependent inactivation of rALDH1A1 with complete inactivation observed at 0.05 mM and 0.1 mM DOPAL when compared with the solvent preincubated enzyme. Similarly, liver cytosolic ALDH exposed to 0.045 mM DOPAL had only 12% residual activity compared with enzyme exposed to an equal concentration of acetaldehydeCitation9. In our experiment, preincubation with 0.0125 mM DOPAL led to a roughly 50% inactivation of rALDH1A1 (). This DOPAL concentration was used in subsequent experiments designed to test the ability of Alda-1 and the virtual screening hit compounds to activate ALDH1A1. In these experiments, rALDH1A1 was preincubated with 12.5 µM DOPAL in the presence of either 2% DMSO or various concentrations of the compound being tested for 20 min. The residual activity of the enzyme was measured by initiating the reaction with NAD+ and propanal and following the increase in fluorescence. The % activity for the compound reaction in this assay was calculated using the background subtracted readings for the compound reaction as well as the 2% DMSO reaction as explained in the Methods section. We first tested the effect of Alda-1 in this assay and found that Alda1 can activate rALDH1A1 in the DOPAL inactivation assay described above with an EC50 of 45 µM and greater than 100% activity at 100 µM (). This is the first report of an activator of rALDH1A1 identified using a physiologically relevant aldehyde, DOPAL. Alda-1 was identified previously as an activator of ALDH2 using a high-throughput assay with acetaldehyde as substrate and a library of 63,500 compoundsCitation10. Alda-1 at 100 µM was shown to increase the activity of ALDH2 with acetaldehyde as the substrate, 2.1-fold over basal activity. Similar to the low potency of Alda-1 observed in our DOPAL inactivation assay, a median EC50 for ALDH2 activation of approximately 20 µM has been reported for this compoundCitation10. Alda-1 (20 µM) was subsequently shown to prevent the rapid inactivation of ALDH2 by 200 µM 4-HNE similar to our finding that this compound can activate rALDH1A1 in the DOPAL inactivation assay. To understand whether Alda-1 can directly activate rALDH1A1 when DOPAL is the only aldehyde substrate, we preincubated rALDH1A1 with either 2% DMSO or different concentrations of Alda-1 and then initiated the reaction with 0.0125 mM DOPAL and 1 mM NAD+. However, this experiment was inconclusive because it was difficult to reliably detect enzyme activity due to rapid leveling off of the fluorescence signal within the first few minutes of the assay (data not shown). The rapid leveling off appears to be consistent with DOPAL-mediated inactivation of enzyme activity. Hence, we cannot make any conclusions as to the mechanism of Alda-1 activation of rALDH1A1 in the DOPAL inactivation assay.
Figure 3. Recombinant ALDH1A1 is rapidly inactivated by DOPAL. rALDH1A1 was preincubated with different concentrations of DOPAL (shown in mM) for 20 min and residual activity was followed after adding an excess of NAD+ and propanal. The residual activity was measured 12 times with an interval of 2 min between reads. Preincubation with DOPAL led to a concentration-dependent inactivation of rALDH1A1 with complete inactivation observed at 0.05 mM (black squares)and 0.10 mM (black circles) when compared to the solvent preincubated enzyme (open triangles).
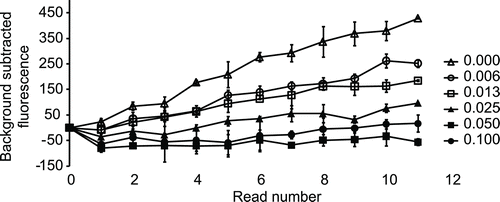
Figure 4 (a). Alda-1 activates rALDH1A1 in the DOPAL inactivation assay with an EC50 of 45 µM. Percent activity was calculated as explained in Methods section. (b) Active hits from the virtual screening in the DOPAL inactivation assay. Compound 1 activated rALDH1A1 in this assay with an activation EC50 = 79 µM whereas, compounds 2 and 3 inhibited rALDH1A1 with inhibition EC50 values of 40 µM and 7 µM, respectively. Percentage activity was calculated as explained in the methods section.
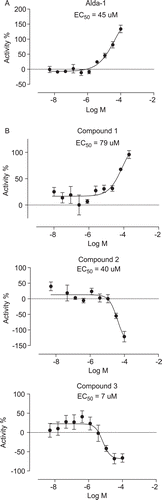
It was reported that Alda-1 specifically activates ALDH2 but not ALDH1 and ALDH5Citation10. However, in this report, acetaldehyde was used as the aldehyde substrate. As mentioned above, we find that Alda-1 can activate ALDH1A1 in the DOPAL inactivation assay. These conflicting findings regarding the specificity of Alda-1 may be due to the different aldehyde substrates used in the two assays. In support of this argument, a strong dependence of ALDH2 activation on the length and nature of the substrate aldehydes has been noted, with linear aliphatic aldehydes activated by Alda-1 but not aromatic aldehydesCitation13.
To determine if ALDH1 activation using small molecules could be targeted as a mechanism of action to treat PD, we designed a strategy to identify small ALDH activators from commercially available chemical libraries (). Since Alda-1 activates ALDH1A1 in the DOPAL assay and ALDH1A1 and ALDH2 are very similar, we decided to carry out a virtual screen using the recently published structure of Alda-1 bound ALDH2 with the intention of identifying additional activators of ALDH1A1. This strategy takes advantage of commercially available small molecules as well as the structure of ALDH1 and ALDH2 determined using X-ray crystallography. The sdf file containing the 2D structures of 19,943 compounds in the Life Chemicals Fragment Library was downloaded from the vendor’s websiteCitation16, and the structures were converted to obtain small molecule representations possessing atomic 3D coordinates with acceptable bound distances. The small molecules were docked individually in the binding site of the ALDH2 activator Alda-1 and the binding energy score was used as the ranking process to obtain virtual hits. However, the restriction of small molecule’s motion upon protein–ligand binding results in an entropic loss that is not calculated in the PLP binding energy scoring function of Molegro Virtual Docker. Therefore, assuming that the loss of ligand entropy upon binding correlates with the loss of torsional entropy estimated as 0.3–0.54 kcal/mol per torsional degree of freedom (rotatable bound)Citation17, the selection of molecules with few rotatable bonds can help to identify molecules that will exhibit the smallest loss of entropy upon binding. Consequently and because we wanted to test only a limited set of compounds, a list of the best 21 virtual hits was created after filtering for compounds with less that 5 rotatable bounds. The compounds were purchased from Life Chemicals and tested in the ALDH1 activation assay to evaluate their effect on ALDH1 activity. Among the prioritized 21 hits, we discovered 3 compounds that had an effect on ALDH1A1 activity in the DOPAL inactivation assay (). Compound 1 activated ALDH1A1 with an activation EC50 of 79 µM. Although the potency of 1 is quite low, we think this result demonstrates the success of our virtual screening approach in being able to rapidly identify a novel activator of ALDH1A1 from commercial chemical providers. It should be noted that a HTS screen of 65,000 compounds yielded just one activator compound for ALDH2Citation10. The identification of Alda-1 and compound 1 should enable the optimization of more potent ALDH1A1 activators. Since compound 1 violates none of Lipinski’s rule of five (MW ≤ 500, numbers of H-bond donors ≤ 5, numbers of H-bond donors ≤ 10, ClogP ≤ 5), it represents a valid alternative retroamide analogue of Alda-1 that can be used as a starting point in a hit to lead medicinal chemistry program to improve its potency. Alternatively, compounds 2 and 3 inhibited ALDH1A1 with an inhibition EC50 of 40 µM and 7 µM, respectively. Potential utility of ALDH inhibitors in several disease areas such as alcoholism, diet-induced obesity, and cocaine addiction has been suggested by recent publicationsCitation18–20. The identification of activators as well as novel inhibitors in our virtual screen was not unexpected because the virtual screening was designed only to identify molecules with favorable ligand–protein energies. The impact of binding could result in the stabilization of the binding site as previously observed with Alda-1Citation13 and produce an activation or interfere with the binding of the substrate and produce an inhibition.
As the experiment to test whether Alda-1 could directly activate rADH1A1 in an assay with DOPAL as the only substrate was inconclusive, we cannot speculate about the direct utility of activators reported here in testing the ALDH1 activation hypothesis. Some innovative modifications to the in vitro assay such as monitoring DOPAL to DOPAC conversion will be required to prove that Alda-1 and compound 1 can directly activate DOPAL metabolism by ALDH1A1. Alternatively, more potent leads derived from these compounds could be tested in vivo or in a suitable cell model to see whether they can ameliorate DOPAL-mediated neurotoxicity. Along these lines, the ALDH2 activator, Alda-1, was shown to be able to protect rat hearts from ischemic damageCitation10 and this is possibly due to reduced accumulation of 4-HNE since 4HNE-modified proteins have been shown to accumulate during cardiac ischemiaCitation21.
Declaration of interest
The authors report no conflicts of interest.
Supplementary Material
Download PDF (71.8 KB)References
- Black W, Vasiliou V. The aldehyde dehydrogenase gene superfamily resource center. Hum Genomics 2009;4:136–142.
- Westlund KN, Denney RM, Kochersperger LM, Rose RM, Abell CW. Distinct monoamine oxidase A and B populations in primate brain. Science 1985;230:181–183.
- Maring JA, Deitrich RA, Little R. Partial purification and properties of human brain aldehyde dehydrogenases. J Neurochem 1985;45:1903–1910.
- Choudhary S, Xiao T, Srivastava S, Zhang W, Chan LL, Vergara LA et al. Toxicity and detoxification of lipid-derived aldehydes in cultured retinal pigmented epithelial cells. Toxicol Appl Pharmacol 2005;204:122–134.
- Zhang M, Shoeb M, Goswamy J, Liu P, Xiao TL, Hogan D et al. Overexpression of aldehyde dehydrogenase 1A1 reduces oxidation-induced toxicity in SH-SY5Y neuroblastoma cells. J Neurosci Res 2010;88:686–694.
- Mandel S, Grunblatt E, Riederer P, Amariglio N, Jacob-Hirsch J, Rechavi G et al. Gene expression profiling of sporadic Parkinson’s disease substantia nigra pars compacta reveals impairment of ubiquitin-proteasome subunits, SKP1A, aldehyde dehydrogenase, and chaperone HSC-70. Ann N Y Acad Sci 2005;1053:356–375.
- Galter D, Buervenich S, Carmine A, Anvret M, Olson L. ALDH1 mRNA: presence in human dopamine neurons and decreases in substantia nigra in Parkinson’s disease and in the ventral tegmental area in schizophrenia. Neurobiol Dis 2003;14:637–647.
- Werner CJ, Heyny-von Haussen R, Mall G, Wolf S. Proteome analysis of human substantia nigra in Parkinson’s disease. Proteome Sci 2008;6:8.
- MacKerell AD Jr, Pietruszko R. Chemical modification of human aldehyde dehydrogenase by physiological substrate. Biochim Biophys Acta 1987;911:306–317.
- Chen CH, Budas GR, Churchill EN, Disatnik MH, Hurley TD, Mochly-Rosen D. Activation of aldehyde dehydrogenase-2 reduces ischemic damage to the heart. Science 2008;321:1493–1495.
- Burke WJ, Kumar VB, Pandey N, Panneton WM, Gan Q, Franko MW et al. Aggregation of alpha-synuclein by DOPAL, the monoamine oxidase metabolite of dopamine. Acta Neuropathol 2008;115:193–203.
- Moore SA, Baker HM, Blythe TJ, Kitson KE, Kitson TM, Baker EN. Sheep liver cytosolic aldehyde dehydrogenase: the structure reveals the basis for the retinal specificity of class 1 aldehyde dehydrogenases. Structure 1998;6:1541–1551.
- Perez-Miller S, Younus H, Vanam R, Chen CH, Mochly-Rosen D, Hurley TD. Alda-1 is an agonist and chemical chaperone for the common human aldehyde dehydrogenase 2 variant. Nat Struct Mol Biol 2010;17:159–164.
- Zhou W, Calciano MA, Jordan H, Brenner M, Johnson S, Wu D et al. High resolution analysis of the human transcriptome: detection of extensive alternative splicing independent of transcriptional activity. BMC Genet 2009;10:63.
- Thomsen R, Christensen MH. MolDock: a new technique for high-accuracy molecular docking. J Med Chem 2006;49:3315–3321.
- Life Chemicals Inc., Burlington, Canada.
- Lazaridis T, Masunov A, Gandolfo F. Contributions to the binding free energy of ligands to avidin and streptavidin. Proteins 2002;47:194–208.
- Arolfo MP, Overstreet DH, Yao L, Fan P, Lawrence AJ, Tao G et al. Suppression of heavy drinking and alcohol seeking by a selective ALDH-2 inhibitor. Alcohol Clin Exp Res 2009;33:1935–1944.
- Ziouzenkova O, Orasanu G, Sharlach M, Akiyama TE, Berger JP, Viereck J et al. Retinaldehyde represses adipogenesis and diet-induced obesity. Nat Med 2007;13:695–702.
- Yao L, Fan P, Arolfo M, Jiang Z, Olive MF, Zablocki J et al. Inhibition of aldehyde dehydrogenase-2 suppresses cocaine seeking by generating THP, a cocaine use-dependent inhibitor of dopamine synthesis. Nat Med 2010;16:1024–1028.
- Eaton P, Li JM, Hearse DJ, Shattock MJ. Formation of 4-hydroxy-2-nonenal-modified proteins in ischemic rat heart. Am J Physiol 1999;276:H935–H943.