Abstract
A series of novel carbazole chalcones has been synthesised and evaluated for radical scavenging activity, cytotoxicity and antimicrobial activities. Compounds 12m, 12o and 12c exhibited good 2,2-diphenyl-1-picrylhydrazyl (DPPH) radical scavenging activity, compounds 12e, 12m and 12d were excellent hydroxyl radical scavengers and compounds 12a, 12e, 12g, 12n and 12m have shown inhibition of oxidative DNA damage induced by 2,2′-azobis (2-amidinopropane hydrochloride). Compounds 12j, 12i, 12n, 12c, 12m and 12e were most active against the selected cancer cell lines. Compounds 12a, 12e and 12m showed good antibacterial activity and compounds 12h and 12m have shown good antifungal activity. All the compounds were subjected for absorption, distribution, metabolism and excretion (ADME) predictions by computational method and found that these molecules could be considered as potential candidates for oral drug development.
Introduction
Antioxidant substances prevent or inhibit the natural phenomena of oxidation. In the recent years, “antioxidant research” has attracted several researchers because of its broad applications in the management and amelioration of a variety of human ailmentsCitation1,Citation2. The formation of reactive oxygen species (ROS) is characteristic of aerobic organisms that can normally defend themselves against these highly reactive species using enzymes like superoxide dismutase and glutathione peroxidase, and naturally occurring antioxidants such as α-tocopherol (vitamin E), ascorbic acid (vitamin C) and β-caroteneCitation3. However, in pathophysiological conditions, the excessive production of ROS overwhelms the natural antioxidant defence mechanisms. This imbalance is termed as oxidative stress. High levels of free radicals can cause damage to biomolecules such as lipids, proteins, enzymes and DNA in cells and tissues, resulting in mutations that can lead to malignancy. Damage to DNA by oxidative stress has been widely accepted as a major cause of cancerCitation4. DNA mutation is a critical step in carcinogenesis and elevated levels of oxidative DNA lesions have been observed in various tumours. The discovery of the role of free radicals in cancer, diabetes, cardiovascular diseases, autoimmuno diseases, neurodegenerative disorders, aging and other diseases has lead to new medical insightCitation5,Citation6. Minimum oxidative damage may be an important approach to the primary prevention or treatment of these diseases. Antioxidants may stop the free radical formation or interrupt an oxidising chain reaction. This has attracted a great deal of research interest in therapeutic antioxidant-based drug formulations. The development of synthetic compounds capable of scavenging free radicals has been a great success.
Carbazole and its derivatives are the important class of heterocyclic compounds endowed with various pharmacological activities such as anticancer, antimicrobial, antiviral, anti-inflammatory and antioxidant activityCitation7. Most of carbazole heterocycles are of natural and synthetic origins and have wide spread distribution in terrestrial plants and micro-organisms. From the bioassay-guided approach, various carbazole alkaloids have been isolated and are subjected for biological evaluation. Naturally occurring carbazole derivatives, murrayafoline A 1 (), carbazomycin A and B 2, have shown potent antimicrobial activityCitation8. Ellipticine 3, a pyridocarbazole, shows potent antiproliferative activity; rebaccamycin 4, an indolocarbazole derivative, acts as an antibiotic and shows inhibition of topoisomerase I and carazostatin 5 is a potent natural antioxidantCitation9–11. The isolation of these molecules spurred the research to synthesise these molecules and its analogues to optimise these natural lead molecules into a clinical molecule. Among the various biological activities exhibited by carbazole derivatives of the particular interest is anticancer activityCitation12. The majority of carbazole derivatives exert cytotoxicity via interaction with DNA. Because these derivatives possess structural features such as flat polycyclic and aromatic chromophore, the optimal size for intercalating chromophore is built by a tricyclic or tetracyclic ring system. Therefore, tricyclic planar carbazole chromophore is predestined for intercalation into DNA. In addition to the DNA binding, carbazole derivatives present antiproliferative effect by other mechanisms, such as inhibition of angiogenesisCitation13, inhibition of cyclin-dependent kinase 414, inhibition of microtubulinCitation15, inhibition of check point kinase 116 and inhibition of topoisomerase I and IICitation17,Citation18. Carvedilol 6, an antihypertensive drug, acts as a nonspecific β-adrenergic antagonistCitation19. Carprofen 7, a nonsteroidal anti-inflammatory drug, is a selective COX-2 inhibitorCitation20.
In continuation of our studies in synthesising various biologically active compoundsCitation21, in this study, we have synthesised and characterised the novel carbazole chalcones and evaluated for antioxidant, cytotoxicity and antimicrobial activities. We also subjected all synthesised compounds for ADME predictions by computational method.
Results and discussion
Chemistry
Chalcone is a unique template that is associated with several biological activities. The biological activities of chalcones are equally wide ranging. In fact, not many structural templates can claim association with a diverse range of pharmacological activities such as antitumor, anti-inflammatory, antiplasmodial, immunosuppression and antioxidant activitiesCitation22,Citation23. With the great biological importance of carbazole derivatives, we synthesised a series of carbazole chalcones from 3-formyl-9-methylcarbazole and various acetophenones (). Carbazole (9) on methylation with methyl iodide gave 9-methyl carbazole (10), which on Vilsmeier–Haack formylation gave 3-formyl-9-methylcarbazole (11). This 3-formyl-9-methylcarbazole (11) on Claisen–Schimdt condensation with various aromatic and heteroaromatic acetophenones in aqueous sodium hydroxide afforded titled compound (12a-o) in good to excellent yields (Table 3, Supplementary information). All the synthesised compounds were characterised by IR, 1H NMR, 13C NMR, CHN analysis and MS .
Biological evaluation
All the synthesised compounds were evaluated for DPPH radical scavenging activity (). The DPPH radical scavenging method is a well-known method for determining antioxidant activityCitation24,Citation25. Among the tested chalcones, compounds 12m, 12o, 12c, 12g, 12n and 12i have shown good radical scavenging activity (89.53%–82.30%) as compared with the standard ascorbic acid (95.76%). Whereas, compounds 12f, 12h, 12k, 12l, 12a and 12e have shown moderate DPPH radical scavenging activity (79.03%–70.76%). The structure activity relationship (SAR) study reveals electron-withdrawing substituents on B ring increases DPPH radical scavenging activity, while electron-donating substituents decreases the activity with the exception of compound 12c. Replacement of aromatic B ring by heteroaromatic ring enhances the DPPH radical scavenging activity.
Table 1. Radical scavenging activity of carbazole chalcones.
Hydroxyl radical is known to react with all components of DNA molecule, damaging both the purine and pyrimidine bases and also the deoxyribose backboneCitation26. Hydroxyl radical scavenging ability of the synthesised chalcones is presented in . The results reveal that compounds 12e, 12m and 12d have shown excellent hydroxyl radical scavenging activity (91.72%–87.59%) as compared with the standard ascorbic acid (88.34%). While compounds 12a, 12j, 12l, 12g, 12i and 12n have shown good hydroxyl radical scavenging activity (86.84%–71.42%) and compounds 12k, 12h, 12f and 12o are moderate OH radical scavengers (69.54%–54.51%). In general, an electron-withdrawing substituent on B ring increases hydroxyl radical scavenging activity, whereas electron-donating substituent on B ring reduces the activity except in case of 12d. Replacing aromatic B ring of 12a by heterocyclic ring, in particular thiophene, decreases the OH radical scavenging activity. The highest occupied molecular orbital (HOMO), a parameter representing the molecular electron-donating ability, is also a good index predicting antioxidant activityCitation27. The compounds possessing higher energy of HOMO (EHOMO) and energy of lowest unoccupied molecular orbital (ELUMO) were found to be effective agents for stabilising the DPPH and hydroxyl radicals; the antioxidant effect of carvedilol mainly resides in carbazole moietyCitation28 and N-ethyl carbazole derivatives acts as electron donorsCitation29; all these properties will explain the high antioxidant activity of the compounds under investigation.
The IC50 values for radical scavenging activities of the tested compounds are listed in . The compounds 12m, 12o and 12c exhibited low IC50 values (47.10–52.49 μg/mL) for DPPH radical scavenging activity. However, the tested compounds did not show DPPH radical scavenging activity as effective as the standard ascorbic acid (IC50 = 45.94 μg/mL). In addition, IC50 values for hydroxyl radical scavenging activity, compounds 12e, 12m and 12d, have shown low IC50 values (35.15–38.60 μg/mL) as compared with the standard ascorbic acid (IC50 = 48.45 μg/mL). IC50 value is defined as the concentration of the sample causing 50% decrease in absorbance; a lower IC50 value would reflect greater radical scavenging activity of the sample.
Permanent modification of genetic material resulting from oxidative damage incidents represents the first step involved in mutagenesis, carcinogenesis and aging. In fact, it is well established that free radical-mediated DNA damage occurs in various cancer tissues. Till date, more than 100 products have been identified from the oxidation of DNA. ROS-induced DNA damage involves single- or double-stranded DNA breaks, purine, pyrimidine or deoxyribose modifications and DNA cross links. DNA damage can result either in arrest or induction of transcription, induction of signal transduction pathways, replication errors and genomic instability, all of which are associated with carcinogenesisCitation30,Citation31. In case of plasmid, damage of DNA results in a cleavage of one of the phosphodiester chains of the supercoiled DNA and produces a relaxed open circular form. Further cleavage near the first breakage results in linear double-stranded DNA molecules. The formation of circular form of DNA is indicative of single-strand breaks and the formation of linear form of DNA is indicative of double-strand breaksCitation32. We have evaluated antioxidant activity of novel carbazole chalcones against 2,2′-azobis (2-amidinopropane hydrochloride) (AAPH)-induced plasmid pUC18 DNA strand breakage using agarose gel electrophoresis analysis ()Citation33. Compounds 12a, 12e, 12g, 12n and 12m have shown inhibition of AAPH-induced DNA breakage. The DNA protection is in the order 12g > 12e > 12n > 12a > 12m. These results confirm the antioxidant activity exhibited by these compounds by DPPH, OH methods as well as AAPH-induced DNA damage.
Figure 2. Agarose gel electrophoresis pattern of pUC 18 DNA strand breakage by 2,2′-azobis (2-amidinopropane hydrochloride) (AAPH) and inhibited by carbazole chalcones. Supercoiled plasmid DNA was incubated with AAPH and/or carbazole chalcones in phosphate-buffered saline (pH 7.4) at 37°C for 1 h. Lane 1: DNA strand breakage induced by AAPH at 10 mM; Lane 2: control super coiled DNA; Lane 3: compound 12g; Lane 4: 12a; Lane 5: 12e; Lane 6: 12n and lane 7: 12m.
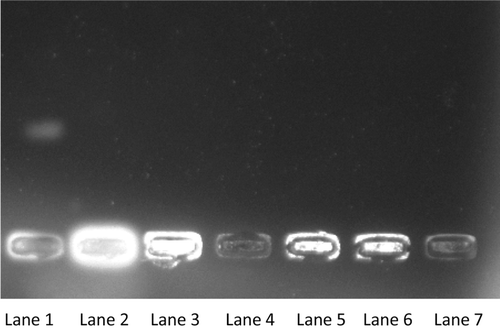
Cytotoxicity of the synthesised compounds was tested against selected three cancer cell lines such as Hep 3BPN7 (liver cancer cell line), HL60 P58 (leukaemia cancer cell line), HeLa-B75 (cervix cancer cell line) and one normal cell line PN-15C-12 (a normal chang liver cell line). The results obtained are summarised in . Compounds 12j, 12i, 12n, 12c, 12m and 12e were found to be most effective against selected cell lines. These compounds inhibit the proliferation of selected three cancer cell lines with highest selectivity (3.85–2.06) than the normal cell lines. Whereas compounds 12f, 12k and 12o showed preferential cytotoxicity against the Hep 3BPN7 and HL60 P58. The SAR studies with respect to cytotoxicity revealed that compounds with electron-withdrawing groups on aromatic ring B show higher cytotoxicity than compounds containing electron-donating groups with the exception of compound 12c. Position of substituents on aromatic ring B influences the cytotoxicity; compound with monofluoro substituent (12e) shows cytotoxicity against all the three cancer cell lines. However, compounds with difluoro substituent (12f and 12g) were cytotoxic to only liver and leukaemia cell line. The bioisosteric replacement of aromatic ring (12a) by heteroaromatic ring (12n) increases cytotoxicity.
Table 2. Cytotoxicity of carbazole chalcones.
Cancer chemoprevention is regarded as a promising avenue for cancer control and has become increasingly important in recent years. Tumourigenesis is a multistep process that begins with cellular transformation, progresses to hyperproliferation and culminates in the acquisition of invasive potential, angiogenic properties and establishment of metastatic lesions. Chemoprevention was described as the use of natural or synthetic chemicals allowing suppression, retardation or inversion of carcinogenesis. Substantial evidence exists to support the role of both oxygen and organic-free radical intermediates in the bimolecular interactions, which contribute to the initiation, promotion and/or progression stages of chemical carcinogenesisCitation34. There are many reports on the synthetic chalcones as chemopreventive agents. First report on the chalcones as chemopreventive agents by Anto et al.,Citation35 described that compounds with good antioxidant activity showed good antitumor activity. In the present case, compounds 12m, 12e and 12a exhibited good antioxidant activity, and these compounds also presented significant cytotoxicity, which reinforces the idea of designing antioxidant-based cancer chemoprevention agents and these compounds could be considered as chemopreventive agents.
The newly synthesised compounds were screened for their antibacterial activity against two Gram-negative strains Escherichia coli (NCIM 2065), Proteus vulgaris (NCIM 2813) and one Gram-positive strain Staphylococcus aureus (NCIM 2120) (Table 4, Supplementary information). Most of the compounds are active against P. vulgaris and E. coli, some of the compounds are inactive against S. aureus. Results show that compounds 12a, 12e and 12m inhibited all the three bacterial growth. Whereas compounds 12b, 12g and 12h inhibited P. vulgaris and E. coli selectively, but compounds 12c and 12o are active against S. aureus. The SAR study indicates that the presence of electron-withdrawing groups on B ring is necessary for antibacterial activity; furthermore, replacement of aromatic B ring by heteroaromatic ring slightly decreases the antibacterial activity.
Pathogenic yeasts of the genus Candida can cause life-threatening infections in immuno-deficient patients. This is because of the higher number of patients with malignancies treated with intensive immunosuppressive therapy regimens as well as their improved survival from formerly fatal bacterial infections and the rising number of patients undergoing allogenic hematopoietic stem cell or organ transplantation. There is an urgent need for novel fungicidal compounds with low toxicity. Synthesised compounds were screened for antifungal activity against Candida albicans (NCIM 3100) using fluconazole as standard, and the results are given in Table 4, Supplementary information. The antifungal screening data revealed that compounds 12h and 12m showed good antifungal activity, while the rest of the compounds were inactive against C. albicans.
About 30% of oral drugs fail in development due to poor pharmacokineticsCitation36. Among the pharmacokinetic properties, a low and highly variable bioavailability is indeed the main reason for stopping further development of the drug. An in-silico model for predicting oral bioavailability is very important, both in the early stage of drug discovery to select the most promising compounds for further optimisation and in the later stage to identify candidates for further clinical development. In-silico prediction of oral bioavailability is pioneered by LipinskiCitation37 “rule of five”; if two out of the following parameters are out of the range, then poor absorption or permeability is possible: the molecular weight is over 500, hydrogen bond donors are more than 5, the calculated octanol–water partition coefficient is over 5 (c Log P), hydrogen bond acceptors are more than 10. Drug likeness can be deduced as a delicate balance among the molecular properties of compound that directly influence its pharmacodynamics and pharmacokinetics and ultimately affect their absorption, distribution, metabolism and excretion in human body like a drugCitation38. Thus, to obtain a good oral drug candidate, we have subjected a series of novel carbazole chalcones to the prediction of Lipinski rule of five and other properties and results are shown in Table 5 (Supplementary information). The results revealed that all the compounds satisfy the Lipinski rule of five. Compounds 12h, 12i, 12j, 12k and 12l have c Log P value more than 5; however, these compounds have molecular weight, hydrogen bond donor, hydrogen bond acceptor within the range of Lipinski rule of five, and these compounds have low polar surface area (PSA). Hence, these compounds do not pose absorption and permeation difficulty.
Hydrogen bonding capacity has also been identified as an important parameter for drug permeability. The results (Table 5, Supplementary information) reveal that compounds under study possessing less number of hydrogen bond donor and hydrogen bond acceptor; PSA provides good correlation with experimental transport data. It has been successfully applied for the prediction of intestinal absorption. PSA equal to or less than 140 will have high probability of good oral bioavailabilityCitation39. Compound having low PSA could be a good oral drug candidate. Navia et al.,Citation40 has postulated the desirability of molecular flexibility for membrane permeation. Molecular flexibility is measured by number of rotatable bonds. Compounds with 10 or fewer rotatable bonds will have probability of good oral bioavailability. The compounds under study have the number of rotatable bonds less than 10. Overall, all the synthesised carbazole chalcones meet the requirement of the Lipinski rule and other physicochemical properties, and may considered as good candidates for oral drug development.
Conclusion
In summary, we have synthesised a series of novel carbazole chalcones and evaluated for cytotoxicity, antioxidant and antimicrobial activities. ADME prediction revealed that the compounds could be considered as good candidates for drug development. Compounds 12m, 12o and 12c exhibited good DPPH radical scavenging activity, compounds 12e, 12m and 12d were excellent hydroxyl radical scavengers, compounds 12g, 12e, 12n, 12a and 12m inhibited the oxidative DNA damage induced by AAPH. Compounds 12j, 12i, 12n, 12c, 12m and 12e have shown promising cytotoxicity against selected cancer cell lines, and they were highly selective to cancer cell line than the normal cell line; SAR study revealed that electron-withdrawing groups increase cytotoxicity. Compounds 12a, 12e and 12m showed good antibacterial activity and compounds 12h and 12m have shown good antifungal activity. Overall, compound 12m, 12e and 12a can be considered as novel carbazole chalcones as lead compounds for the development of novel chemopreventive agents.
Experimental
General
Melting points were recorded in open capillaries with electrical melting point apparatus and were uncorrected. IR spectra (KBr disks) were recorded using a Perkin–Elmer 237 spectrophotometer. 1H NMR (300 MHz) and 13C NMR (100 MHz) spectra were recorded on a Bruker Avance spectrometer using DMSO-d6 as solvent. Mass spectra were recorded on a Shimadzu LCMS-QP 1000 EX. Elemental microanalysis was performed on Carlo Erba 1108 (CHN) Analyser. All the reagents and solvents used were of analytical grade and were used as supplied unless otherwise stated. Thin layer chromatography (TLC) was performed on silica gel-coated plates for monitoring the reactions.
Synthesis of 9-methyl carbazole (10)
Carbazole 9 (4.1 g, 25 mmol) was dissolved in 50 mL DMF and NaH (1.8 g, 75 mmol) was added to it. The solution was stirred for 10 min and methyl iodide (7.05 g, 50 mmol) was added with constant stirring and stirring continued at room temperature for 3 h. After completion of reaction (TLC), the reaction mass was poured over crushed ice. The obtained pale yellow solid of 9-methyl carbazole 10 was filtered, washed with water and dried. Thus, the 9-methyl carbazole 10 (mp: 97°C, yield 94%) obtained was sufficiently pure and used further without purification.
Synthesis of 3-formyl-9-methyl carbazole (11)
9-methyl carbazole 10 (1.81 g, 10 mmol) was dissolved in dry DMF (20 mL) under anhydrous condition. It was cooled to 0°C, and POCl3 (1.87 mL) was added drop wise for 30 min and stirring continued for 4 h at 80°C. After completion of reaction (TLC), reaction mass was poured over crushed ice (50 g), basified with NaOH, extracted with chloroform and dried over anhydrous Na2SO4. Organic layer was concentrated and purified through silica gel column using chloroform as eluting solvent to yield product 11 (1.15 g). Yield: 55%; off white solid; mp: 76°C–78°C; IR (cm−1): 3035, 2704, 1685, 1626, 1591, 1570, 1050, 750; 1H NMR (300 MHz, DMSO-d6, δ in ppm): 3.91 (s, 3H, NCH3), 7.35 (t, 1H, J = 8.2 Hz, ArH), 7.40–7.60 (m, 3H, ArH), 7.98 (d, 1H, J = 8.2 Hz, ArH), 8.17 (d, 1H, J = 8.2 Hz, ArH), 8.60 (s, 1H, ArH), 10.1 (s, 1H, −CHO); m/z = 210.20 (m + 1); Anal. Calcd for C14H11NO: C, 80.36; H, 5.30; N, 6.69%. Found: C, 80.34; H, 5.31; N, 6.71%.
Synthesis of chalcones (13a–o)
A mixture of 3-formyl-9-methyl carbazole 7 (1 mmol) and substituted acetophenones (1 mmol) was dissolved in 20 mL ethanol. To this mixture, sodium hydroxide (20%, 1 mL) was added at 0°C–10°C. The reaction mixture was stirred at room temperature for 24–36 h. Then, this reaction mixture was poured over crushed ice and stirred. The solid thus obtained was filtered, washed with water and dried. The solid products were purified by recrystallisation using methanol to afford pure compounds 12a-o.
3-(9-Methyl-9H-carbazol-3-yl)-1-phenyl-propenone (12a)
MP: 148°C–149°C; IR (cm−1): 3062, 2933, 2780, 1699, 1644, 1279, 1050, 750; 1H NMR (300 MHz, DMSO-d6, δ in ppm): 3.93 (s, 3H, NCH3), 7.27 (t, 1H, J = 7.5 and 7.8 Hz, ArH), 7.47–7.72 (m, 6H, ArH and CH=CH), 7.96 (s, 2H, ArH), 8.02 (d, 1H, J = 8.7 Hz, ArH), 8.15–8.26 (m, 3H, ArH), 8.74 (s, 1H, ArH); 13C NMR (100 MHz, DMSO-d6, δ in ppm): 189.8, 144.4, 139.5, 138.2, 137.0, 135.2, 131.1, 130.2, 128.5, 127.0, 125.5, 122.0, 120.6, 111.3, 109.8, 29.3; m/z = 312 (m + 1); Anal. Calcd for C22H17NO: C, 84.86; H, 5.50; N, 4.50%. Found: C, 84.89; H, 5.53; N, 4.47%.
DPPH radical scavenging activity
The DPPH radical scavenging activity of chalcones was measured according to the procedure described by Blois with minor modificationCitation41. The reaction mixture contained test compound with equal volume of DPPH radical (10−4 M in absolute ethanol) solution. After 20 min reaction time, the absorbance was recorded at 517 nm using UV–visible spectrophotometer. Ascorbic acid was used as a standard antioxidant agent. For determination of IC50 value, various concentrations of test compounds were taken, and the concentration required for 50% decrease in the absorbance of a control solution of DPPH was expressed as IC50.
Hydroxyl radical scavenging activity
Hydroxyl radical scavenging activity was determined by the earlier reported methodCitation42. The reaction cocktail contained 60 μL of 1 mM FeCl3, 90 μL of 1 mM 1,10-phenanthroline, 2.4 mL of 0.2 M phosphate buffer (pH 7.8), 150 μL of 0.17 M H2O2 and 1.5 mL of test compound in DMSO. Reaction mixture was kept at room temperature for 5 min incubation, and absorbance was measured at 560 nm using spectrophotometer. Ascorbic acid was used as a reference compound.
Inhibition of AAPH-induced oxidative DNA damage
The inhibition of AAPH-induced oxidative DNA damage by novel carbazole chalcones was assessed by measuring the conversion of super coiled pUC 18 plasmid DNA to open circular forms by gel electrophoresisCitation33. pUC 18 DNA (2 μL) was incubated with test compounds (8 μL) at 100 μg concentration, and AAPH in phosphate-buffered saline (PBS) (8 μL) at pH 7.4 and 37°C for 1 h. After incubation, the sample (10 μL) was mixed with 2 μL of gel-loading buffer (0.13% bromophenol blue and 30% [W/V] glycerol) and immediately loaded onto prestained 0.8% agarose gel with ethidium bromide (10 μg/mL) and the gel electrophoresis was performed for 1 h. Clear fluorescent plasmid DNA bands were observed and photographed under gel documentation system. (UVItec Cambridge, UK).
Cytotoxicity of novel carbazole chalcones
The cytotoxicity assay was performed as per the earlier reported method with slight modificationCitation43. The cells were harvested (2.5–3 × 104 cells/well) and inoculated in 96-well microtiter plate. The cells were washed with PBS and the cultured cells were then incubated in with and without test compound (100 μg). After 72 h incubation, the medium is aspirated. About 10 μL of MTT solution (5 mg/mL in PBS, pH 7.2) was added to each well, and the plates were incubated for 4 h at 37°C. After incubation, 100 μL of DMSO (<0.5%) was added to the wells followed by gentle shaking to solubilise the formation of dye for 15 min. Absorbance was read at 540 nm and surviving cell fraction was calculated. Methotrexate was used as reference drug. The inhibition of cell viability was calculated as follows:
Where T - absorbance of treated cells, C - absorbance of untreated cells
Antimicrobial activity (agar diffusion method)
The antimicrobial activity was tested by agar diffusion methodCitation44. The test organism was inoculated on nutrient agar plates and spread uniformly using a sterile glass spreader. Wells of 6 mm in diameter were made on the nutrient agar using a sterile cork borer. In each well, the test compound (100 μg) was added. The plates were incubated at 37°C for 24 h for bacteria. The above procedure is followed for fungal assays; the media used was yeast peptone dextrose agar medium incubated at 30°C for 24 h. Each test was performed in duplicate and the results were shown as means. The zone of inhibition was measured in millimeter, while standard antibiotics were used as positive control.
Calculation of drug-likeness properties
The physicochemical properties such as molecular weight, c Log P, hydrogen bond acceptors (HBA), hydrogen bond donors (HBD) and drug likeness of the synthesised carbazole chalcones are studied from online Osiris property explorer for drug bioavailability of chemical compoundsCitation45. PSA, molecular volume and quantum chemical descriptors such as EHOMO and ELUMO of synthesised compounds were calculated by using a Bio Med CaChe 6.1 (BioMed CAChe 6.1, Fujitsu Limited, Chiba, Japan).
Declaration of interest
The authors report no conflicts of interest.
Supplementary Material
Download PDF (84.6 KB)References
- Aruoma O J, Halliwell B. Free Radicals and Food Additives (Eds) Talylor and Francis, London, 1991.
- Halliwell B, Murcia MA, Chirico S, Aruoma OI. Free radicals and antioxidants in food and in vivo: what they do and how they work. Crit Rev Food Sci Nutr 1995;35:7–20.
- Bursal E, Gülçin I. Polyphenol contents and in vitro antioxidant activities of lyophilised aqueous extract of kiwifruit (Actinidia deliciosa). Food Research International. 2011;44: 1482–1489
- Dröge W. Free radicals in the physiological control of cell function. Physiol Rev 2002;82:47–95.
- Ratnam DV, Ankola DD, Bhardwaj V, Sahana DK, Kumar MN. Role of antioxidants in prophylaxis and therapy: A pharmaceutical perspective. J Control Release 2006;113:189–207.
- Suzen S. Bioactive Heterocycles V, Chapter: “Antioxidant Activities of Synthetic Indole Derivatives and Possible Activity Mechanisms. Top Heterocycl Chem 2007;11:145–178.
- Knölker HJ, Reddy KR. Isolation and synthesis of biologically active carbazole alkaloids. Chem Rev 2002;102:4303–4427.
- Zhang FF, Gan LL, Zhou CH. Synthesis, antibacterial and antifungal activities of some carbazole derivatives. Bioorg Med Chem Lett 2010;20:1881–1884.
- Asche C, Frank W, Albert A, Kucklaender U. Synthesis, antitumour activity and structure-activity relationships of 5H-benzo[b]carbazoles. Bioorg Med Chem 2005;13:819–837.
- Long BH, Rose WC, Vyas DM, Matson JA, Forenza S. Discovery of antitumor indolocarbazoles: rebeccamycin, NSC 655649, and fluoroindolocarbazoles. Curr Med Chem Anticancer Agents 2002;2:255–266.
- Knolker H-J, Reddy KR. Chemistry and biology of carbazole alkaloids The alkaloids: Chemistry and Biology 2008;65:181–193.
- Asche C, Demeunynck M.Antitumor carbazoles. Anticancer Agents Med Chem 2007;7:247–267.
- Arbiser JL, Govindarajan B, Battle TE, Lynch R, Frank DA, Ushio-Fukai M et al. Carbazole is a naturally occurring inhibitor of angiogenesis and inflammation isolated from antipsoriatic coal tar. J Invest Dermatol 2006;126:1396–1402.
- Fousteris MA, Papakyriakou A, Koutsourea A, Manioudaki M, Lampropoulou E, Papadimitriou E et al. Pyrrolo[2,3-a]carbazoles as potential cyclin dependent kinase 1 (CDK1) Inhibitors. Synthesis, biological evaluation, and binding mode through docking simulations. J Med Chem 2008;51:1048–1052.
- Hu L, Li ZR, Li Y, Qu J, Ling YH, Jiang JD et al. Synthesis and structure-activity relationships of carbazole sulfonamides as a novel class of antimitotic agents against solid tumors. J Med Chem 2006;49:6273–6282.
- Conchon E, Anizon F, Aboab B, Prudhomme M. Synthesis and biological activities of new checkpoint kinase 1 inhibitors structurally related to granulatimide. J Med Chem 2007;50:4669–4680.
- Lampropoulou E, Manioudaki M, Fousteris M, Koutsourea A, Nikolaropoulos S, Papadimitriou E. Pyrrolo[2,3-a]carbazole derivatives as topoisomerase I inhibitors that affect viability of glioma and endothelial cells in vitro and angiogenesis in vivo. Biomed Pharmacother 2011;65:142–150.
- Joseph B, Facompré M, Da Costa H, Routier S, Mérour JY, Colson P et al. Synthesis, cytotoxicity, DNA interaction and topoisomerase II inhibition properties of tetrahydropyrrolo[3,4-a]carbazole-1,3-dione and tetrahydropyrido-[3,2-b]pyrrolo[3,4-g]indole-1,3-dione derivatives. Bioorg Med Chem 2001;9:1533–1541.
- Noguchi N, Nishino K, Niki E. Antioxidant action of the antihypertensive drug, carvedilol, against lipid peroxidation. Biochem Pharmacol 2000;59:1069–1076.
- Zall A, Kieser D, Höttecke N, Naumann EC, Thomaszewski B, Schneider K et al. NSAID-derived ?-secretase modulation requires an acidic moiety on the carbazole scaffold. Bioorg Med Chem 2011;19:4903–4909.
- Bandgar BP, Sarangdhar RJ, Viswakarma S, Ahamed FA. Synthesis and biological evaluation of orally active prodrugs of indomethacin. J Med Chem 2011;54:1191–1201.
- Dimmock JR, Elias DW, Beazely MA, Kandepu NM. Bioactivities of chalcones. Curr Med Chem 1999;6:1125–1149.
- Go ML, Wu X, Liu XL. Chalcones: an update on cytotoxic and chemoprotective properties. Curr Med Chem 2005;12:481–499.
- Gülçin I, Topal F, Cakmakci R, Bilsel M, Goren A C, Erdogan U. Pomological Features, Nutritional Quality, Polyphenol Content Analysis, and Antioxidant Properties of Domesticated and 3 Wild Ecotype Forms of Raspberries (Rubus idaeusL.). J Food Sci 2011;76:585–593.
- Gülçin I. Antioxidant Activity of Eugenol: A Structure–Activity Relationship Study. J Medicinal Food 2011;14:975–985.
- Halliwell B, Gutteridge J.M.C. Free radicals in biology and medicine, 3rd ed. Oxford university press. oxford, UK. 1999.
- Kancheva VD, Saso L, Boranova PV, Khan A, Saroj MK, Pandey MK et al. Structure-activity relationship of dihydroxy-4-methylcoumarins as powerful antioxidants: correlation between experimental & theoretical data and synergistic effect. Biochimie 2010;92:1089–1100.
- Kumar R, Ramachandran U, Srinivasan K, Ramarao P, Raichur S, Chakrabarti R. Design, synthesis and evaluation of carbazole derivatives as PPAR alpha/gamma dual agonists and antioxidants. Bioorg Med Chem 2005;13:4279–4290.
- Shi HP, Xu L, Cheng Y, He JY, Dai JX, Xing LW et al. Experimental and theoretical study of three new benzothiazole-fused carbazole derivatives. Spectrochim Acta A Mol Biomol Spectrosc 2011;81:730–738.
- Marnett LJ. Oxyradicals and DNA damage. Carcinogenesis 2000;21:361–370.
- Cooke MS, Evans MD, Dizdaroglu M, Lunec J. Oxidative DNA damage: mechanisms, mutation, and disease. FASEB J 2003;17:1195–1214.
- Burrows CJ, Muller JG. Oxidative Nucleobase Modifications Leading to Strand Scission. Chem Rev 1998;98:1109–1152.
- Rahman A, Fazal F, Greensill J, Ainley K, Parish JH, Hadi SM. Strand scission in DNA induced by dietary flavonoids: role of Cu(I) and oxygen free radicals and biological consequences of scission. Mol Cell Biochem 1992;111:3–9.
- Trush MA, Kensler TW. An overview of the relationship between oxidative stress and chemical carcinogenesis. Free Radic Biol Med 1991;10:201–209.
- Anto RJ, Sukumaran K, Kuttan G, Rao MN, Subbaraju V, Kuttan R. Anticancer and antioxidant activity of synthetic chalcones and related compounds. Cancer Lett 1995;97:33–37.
- van de Waterbeemd H, Gifford E. ADMET in silico modelling: towards prediction paradise? Nat Rev Drug Discov 2003;2:192–204.
- Lipinski C A, Lombardo F, Dominy B W, Feeney P J. Experimental and computational approaches to estimate solubility and permeability in drug discovery and development settings Adv. Drug Delivery Rev. 1997;23:3–25.
- Hou T, Wang J, Zhang W, Xu X. ADME evaluation in drug discovery. 6. Can oral bioavailability in humans be effectively predicted by simple molecular property-based rules? J Chem Inf Model 2007;47:460–463.
- Veber DF, Johnson SR, Cheng HY, Smith BR, Ward KW, Kopple KD. Molecular properties that influence the oral bioavailability of drug candidates. J Med Chem 2002;45:2615–2623.
- Navia M A, Chaturvedi P R. Design Principles for Orally. Bioavailable Drugs. Drug Discovery Today 1996;1:179–189.
- Blois M S. Antioxidant Determinations by the Use of a Stable Free Radical. Nature. 1958;181:1199–1200.
- Yu W, Zhao Y, Shu B. The radical scavenging activites of radix puerariae isoflavanoids: A chemiluminescence study. Food Chem 2004;86:525–529.
- Wahab S I A, Abdul A B, Mohan S M, Al-Zubairi A S, Elhassan M M, Ibrahim M Y. Biological activities of Pereskia bleo extracts. Int J Pharmacol 2009;5:71–75.
- Vadlapudi V, Bobbarala V, Penumajji S, Naidu K C. Excoecaria agallocha L. Antimicrobial Properties against Important Pathogenic Microorganisms. Int J Pharm Tech Res. 2009;4:865–867.
- Miteva MA, Violas S, Montes M, Gomez D, Tuffery P, Villoutreix BO. FAF-Drugs: free ADME/tox filtering of compound collections. Nucleic Acids Res 2006;34:W738–W744.