Abstract
Severe acute respiratory syndrome coronavirus (SARS-CoV) papain-like protease (PLpro) is a key enzyme that plays an important role in SARS virus replication. The ethanol extract of the seeds of Psoralea corylifolia showed high activity against the SARS-CoV PLpro with an IC50 of value of 15 µg/ml. Due to its potency, subsequent bioactivity-guided fractionation of the ethanol extract led to six aromatic compounds (1–6), which were identified as bavachinin (1), neobavaisoflavone (2), isobavachalcone (3), 4′-O-methylbavachalcone (4), psoralidin (5) and corylifol A (6). All isolated flavonoids (1–6) inhibited PLpro in a dose-dependent manner with IC50 ranging between 4.2 and 38.4 µM. Lineweaver–Burk and Dixon plots and their secondary replots indicated that inhibitors (1–6) were mixed inhibitors of PLpro. The analysis of KI and KIS values proved that the two most promising compounds (3 and 5) had reversible mixed type I mechanisms.
Severe acute respiratory syndrome coronavirus (SARS-CoV) is a zoonotic RNA virus that is highly contagious and causes fatal respiratory illness. SARS-CoV has been controlled by inhibiting a number of significant targets involved in viral replication. SARS-CoV has been controlled by inhibiting a number of significant targets involved in viral replication. An early and essential process of SARS-CoV replication is the cleavage of a multidomain viral polyprotein into 16 mature components required for viral RNA synthesisCitation1. It is well known that two cysteine proteases, a papain-like protease (PLpro) and a 3 C-like protease are the key players in this maturation processCitation2. These two proteases catalyze their own release and liberate other nonstructural proteins from the polyprotein. Recent structural and functional studies directed at PLpro have suggested potential roles for this protease beyond viral peptide cleavage, including deubiquitination, deISGylation and involvement in virus evasion of the innate immune responseCitation3–5. The numerous functions of PLpro in viral replication and pathogenesis suggest that PLpro may serve as an attractive target for antiviral drugs. Many rationally designed compounds have been developed as inhibitors of SARS-CoV PLpro. However, natural compounds exhibiting SARS-CoV PLpro inhibition activity are yet to be reported.
During an intensive research program into biologically active metabolites from Psoralea corylifolia, we found that the ethanol extract showed significant inhibition against SARS-CoV PLpro. Psoralea corylifolia belongs to the Leguminosae family, the seeds of which are a permitted food additive in many countries, especially South Korea. The major bioactive components of the seeds of P. corylifolia are flavonoids and chalcones, including bavachinin, psoralidin, and isobavachalconeCitation6. Moreover, the constituents of P. corylifolia have been found to exhibit antioxidant, antibacterial, anti-inflammatory and antidepressant activitiesCitation7–9. Psoralea corylifolia has also been reported to have inhibitory activities of baculovirus-expressed BACE-1, DNA polymerase and topoisomerase IICitation10,Citation11. We also reported glycosidase inhibitory phenolic compounds from this speciesCitation12.
In this study, we isolated six aromatic compounds targeting SARS-CoV PLpro from the seeds of P. corylifolia. The isolated compounds were evaluated separately for their inhibitory activities against PLpro. Their inhibition mechanisms were ascertained using Lineweaver–Burk and Dixon plots. The literature revealed that no such work has been reported on the seeds of P. corylifolia.
Materials and methods
Plant material, extraction and isolation
The dried seeds (1 kg) of P. corylifolia were purchased from the local market and extracted with ethanol (2 l) three times at room temperature. The ethanol soluble portion was concentrated to give the crude extract (87 g), which was partitioned between H2O and n-hexane. The aqueous layer was further partitioned with EtOAc to give the EtOAc extract (59 g), which was subjected to MPLC on a silica gel column with a gradient solvent system of n-hexane–CHCl3–MeOH to provide six fractions (A–F). Potent inhibition targeting to PLpro was found on fraction C that was further chromatographed on a Sephadex LH-20 (Piscataway, NJ) column with MeOH as the eluent to give five main fractions (Fr1–Fr5). The Fr2 was purified by silica gel column (230–400 mesh) chromatography with a gradient solvent system of n-hexane–acetone (100:0–10:1) to afford psoralidin (18 mg, 5) and corylifol A (15 mg, 6). The Fr3 was chromatographed with a gradient solvent system of n-hexane–EtOAc (50:1–5:1) to yield four sub-fractions (Fr3-1–Fr3-4). The Fr3-2 was separated to more six fractions (Fr3-2-1–Fr3-2-6) by ODS column chromatography with 80% MeOH as the eluent. The Fr3-2-1 was further purified on a silica gel column with CHCl3–EtOAc (100:1–30:1) as the eluent to afford isobavachalcone (42 mg, 3) and 4′-O-methylbavachalcone (31 mg, 4). The Fr3-2-3 was purified on a Sephadex LH-20 column with MeOH as eluent to give isobavachromene neobavaisoflavone (18 mg, 2). Another main fraction (Fr6) was subject to silica gel column chromatography eluting with CHCl3–acetone (50:1–10:1) to yield seven sub-fractions (Fr6-1–Fr6-7). The Fr6-1 was chromatographed on a Sephadex column with CH2Cl2–MeOH (1:4) as the eluent and more five fractions (Fr6-1-1–Fr6-1-5) were obtained. The Fr6-1-4 was separated by ODS with 70% MeOH in H2O as the solvent system to give bavachinin (13.2 mg, 1). The chemical structures of these compounds were determined by comparison of their spectroscopic data with those previously reportedCitation13.
Expression and purification of SARS-CoV PLpro from Escherichia coli
The PLpro gene (945 bp) was PCR amplified from the plasmid pSARS-REP and plasmid pPRoEx HT expression vector (Invitrogen, Carlsbad, CA) and transformed into DH5α competent cells. The expression plasmid was constructed such that PLpro carried an N-terminal His6-tag followed by a tobacco etch virus protease (TEV) site. Correct clones containing the PLpro gene in a pPRoEX HT vector were identified and verified by PCR and restriction digestion with BamHI and XhoI. The plasmid pPRoEx HT harboring the PLpro gene was transformed into BL21(DE3) E. coli (Novagen, Madison, WI). A 10 ml aliquot of an overnight culture was seeded into 1000 ml of fresh Luria–Bertani medium containing 50 mg/ml ampicillin and cells were grown to an OD600 nm of 0.6 at 37 °C. The cells were cooled on ice for 30 min and protein expression was induced for 5 h with 0.4 mM isopropyl β-d-1-thiogalactopyranoside at 30 °C. The cells were harvested by centrifugation at 6000 rpm for 6 min at 4 °C. The harvested cells were washed twice in phosphate-buffered saline. The cell pellet was used directly for purification or stored at −80 °C until use.
The cell pellet was suspended in binding buffer (50 mM NaH2PO4 pH 8.0, 500 mM NaCl, 5 mM imidazole and 5 mM β-mercaptoethanol) and cells were disrupted by sonication. After centrifugation at 15 000 rpm for 1 h, the clear supernatant was collected, filtered (Advantec, Saijyo, Ehime, Japan) and applied onto a column of Nickel Sepharose 6 Fast Flow (GE Healthcare, Stockholm, Sweden) beads pre-equilibrated with the binding buffer. The column was washed first with 20 column volumes of binding buffer and then with 2 column volumes of washing buffer (50 mM Tris–HCl pH 8.0, 500 mM NaCl and 30 mM imidazole). Recombinant PLpro was eluted with elution buffer (50 mM Tris–HCl pH 8.0, 100 mM NaCl and 300 mM imidazole). Eluted PLpro was further purified by ion-exchange chromatography using a salt gradient with a SOURCE 15Q column (GE Healthcare, Piscataway, NJ) in 50 mM Tris–HCl pH 8.5 and 2 mM DTT. PLpro was finally purified by size exclusion chromatography on a Superdex 200 column (GE Healthcare, Piscataway, NJ) in 20 mM Tris–HCl pH 8.0, 150 mM NaCl and 2 mM DTT. The fractions containing PLpro were pooled, exchanged into 20 mM Tris–HCl pH 8.0 and 10 mM DTT and concentrated to a final concentration of 10 mg/mL by ultrafiltration (Microcon YM-30, Millipore Corporation, Bedford, MA). The protein purity was examined by SDS-PAGE and native-PAGE. The protein concentration was determined by Bradford assayCitation14 using bovine serum albumin as the standard. The N-terminal his-tag was removed by TEV digestion prior to activity assays.
SARS-CoV PLpro inhibition assay
IC50 values for all inhibitors were determined using a 96-well plate-based assay similar to our previously reported proceduresCitation15,Citation16. The substrate used in the assay was the fluorogeneic peptide Z-Arg-Leu-Arg-Gly-Gly-7-amido-4-methylcoumarin (Z-RLRGG-AMC), which was purchased from ENZO Life Sciences (Farmingdale, NY). The substrate contains the five C-terminal residues of human ubiquitin with a C-terminal AMC group. Hydrolysis of the AMC–peptide bond dramatically increases the fluorescence of the AMC moiety, allowing conversion to be accurately determined. Reactions were performed in a total volume of 200 µL, which contained the following components: 20 mM Tris-buffer, pH 8.0, 10 mM DTT, 30 µM Z-RLRGG-AMC, 2% DMSO and varying concentrations of inhibitor (0–200 µM). Assays were initiated with the addition of PLpro to produce a final enzyme concentration of 60 nM. Reaction progress was monitored continuously on a SpectraMax M3Multi-Mode Microplate Reader (Sunnyvale, CA) (γexcitation = 360 nm; γemission = 460 nm and gain = 40) and the reaction rate was calculated by the equation vi = vo/(1 + [I]/IC50) using the enzyme kinetics module of Sigma Plot (v. 9.01 Systat Software, Inc., Chicago, IL) where vi is the reaction rate in the presence of inhibitor, vo the reaction rate in the absence of inhibitor and [I] the inhibitor concentration.
Enzyme kinetic assay and progress linear determination
The inhibition kinetics of the enzyme by the isolated compounds were analyzed by Lineweaver–Burk plots and compared to data obtained in the absence of inhibitor. To determine the kinetic parameters associated with inhibition mechanism of PLpro, steady-state rates were obtained at several inhibitor concentrations and varying substrate concentrations. The two inhibition constants for inhibitor binding with either free or enzyme–substrate complex, KI or KIS, were obtained from secondary plots of the slopes of the straight lines or vertical intercept respectively, versus the concentration of inhibitors. KI and KIS are represented by Equations (1)–(3), respectivelyCitation17,Citation18:
Statistical analysis
All measurements were made in triplicate. The results were subject to variance analysis using Sigma plot. Differences were considered significant at p < 0.05.
Results and discussion
In preliminary screening, we observed that the ethanol extract of P. corylifolia seeds showed significant inhibition (IC50 =15 µg/ml) of SARS-CoV PLpro. Activity-guided fractionation of the ethanol extract gave six flavonoids (1–6), which were purified over silica gel, Sephadex LH-20, and octadecyl-functionalized silica gel as delineated above. Isolated compounds (1–6) were identified as the known species bavachinin (1), neobavaisoflavone (2), isobavachalcone (3), 4′-O-methylbavachalcone (4), psoralidin (5) and corylifol A (6) through analysis of spectroscopic data and comparison with previous studies (). Especially, molecular formulas, degrees of unsaturation and number of rings for each compounds were confirmed by 13C-NMR and HREIMS: m/z 338.1520 [M]+ for 1; m/z 322.1204 [M]+ for 2; m/z 324.1635 [M]+ for 3; m/z 338.1519 [M]+ for 4; m/z 336.0995 [M]+ for 5 and m/z 390.1836 [M]+ for 6.
The SARS-CoV PLpro (residues 154–1855) was expressed in E. coli and purified by successive nickel affinity, ion-exchange and gel filtration chromatography. The last step was used to separate the desired monomer from larger oligomers. The mass of the isolated PLpro was confirmed by MALDI-TOF. The His6-tag used for purification was removed by TEV cleavage. Activity of the purified PLpro was demonstrated by its ability to cleave a fluorogeneic peptide substrate (supplementary materials). The apparent Michaelis–Menten constant (Km = 30 ± 0.9 µM) was determined by plotting the initial rates corrected for the enzyme concentration (60 nM) versus substrate concentration (5–320 µM) and fitting the data to the Michaelis–Menten model (supplementary materials).
All isolated flavonoids (1–6) inhibited PLpro in a dose-dependent manner with IC50s ranging between 4.2 and 38.4 µM ( and ). However, two representative secondary metabolites of P. corylifolia, psoralen and isopsoralen (both unfunctionalized furocoumarins) were inactive against PLpro up to 150 µM. Coumestrol (5) was found to be the most potent inhibitor with an IC50 of 4.2 µM. Chalcones (3 and 4) exhibited a significant degree of inhibition (IC50 = 7.3−10.1 µM) in comparison to flavanones (1) and isoflavones (2 and 6). The KI values of all compounds were determined to fall within the range 1.7–18.4 µM, from the common x-axis intercept of lines on the corresponding Dixon plots (). Kinetic assays were repeated in the presence of different concentrations (1–150 µM) of compounds (1–6) to characterize inhibition of Z-RLRGG-AMC hydrolysis. The inhibition of PLpro by compound 5 (the most effective species) is shown in , representatively. Plots of the initial velocity versus enzyme concentration in the presence of different concentrations of compound 5 gave a family of straight lines, all of which passed through the origin. Increasing the inhibitor concentration resulted in lowering of the slope of the line, indicating that compound 5 is a reversible inhibitor (). Other tested inhibitors (1–4 and 6) showed a similar profile to 5.
Figure 2. (A) Effects of isolated compounds 1–6 on SARS-CoV PLpro for the hydrolysis of Z-RLRGG-AMC, (B) the catalytic activity of SARS-CoV PLpro as a function of enzyme concentration at different concentrations of compound 5 and (C–H) Dixon plots for the inhibition of compounds (1–6), respectively, on the hydrolysis activity of PLpro in the presence of different concentrations of substrate.
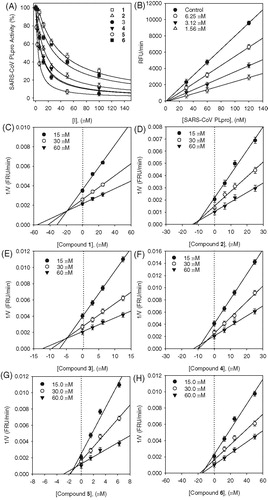
Figure 3. (A–B) Kinetic assays of SARS-CoV PLpro inhibition by isobavachalcone (3) and psoralidin (5). Lineweaver–Burk plots were constructed for the inhibition of SARS-CoV PLpro by compounds (3 and 5). The plot is expressed as 1/velocity versus 1/PLpro (nM−1) with or without inhibitor. Insets (I) and (II) represent the secondary plot of the slope and the intercept of the straight lines versus concentration of compounds (3 and 5), respectively.
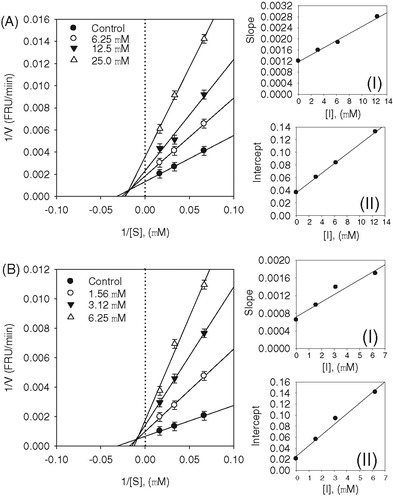
Table 1. Inhibitory effects of compounds 1–6 on PLpro activity.
The enzyme inhibition properties of these derivatives were modeled using double-reciprocal plots (Lineweaver–Burk and Dixon analyses). As shown in , the inhibition kinetics analyzed by Lineweaver–Burk plots show that compounds 3 and 5 are mixed-type inhibitors because increasing inhibitor concentration resulted in a family of lines which intersected at a nonzero point on both x- and y-axes. The equilibrium constant for inhibitor binding, KI was obtained from the value at the intersection of three lines from Dixon plots (). The KI values of inhibitors are presented in .
Since the inhibition was mixed, this means that the inhibitor has different affinities for the substrate bound and free enzyme. We thus sought to parse out the respective inhibition constants for the two states, which are referred to as type I, where inhibitor preferably binds to the free enzyme, or type II, where the inhibitor preferentially binds to the enzyme substrate complex. This analysis is carried out by varying both inhibitor and substrate concentration, as shown by Equations (1)–(3). The equilibrium constants for the two different binding events, inhibitor binding to free enzyme (KI) and enzyme–substrate complex (KIS) were obtained from secondary plots of Km/Vmax and 1/Vmax versus concentration of compounds 3 and 5, respectively. We thus established the following constants: compound 3, KI = 4.9 µM, and KIS = 9.5 µM; compound 5, KI = 1.7 µM and KIS = 4.2 µM ( insets). These data show that the affinity of the inhibitor for free enzyme is marginally stronger than the affinity of inhibitor for the enzyme–substrate complex. Thus, compounds 3 and 5 are mixed type I inhibitors.
This study demonstrates that the ethanol extract of P. corylifolia seeds shows potent inhibitory activity toward SARS-CoV PLpro. Purification of this fraction gave six phenolic phyotchemicals that displayed good PLpro inhibitory activities. These data validate P. corylifolia as rich source of potent PLpro inhibitors. Two compounds, isobavachalcone (3), and psoralidin (5) were the principal contributors to the PLpro inhibition. The analysis of KI and KIS values proved that the two most promising compounds (3 and 5) had a reversible mixed type I behavior.
Declaration of interest
The authors have declared that there is no conflict of interest.
This study was supported by National Research Foundation Grant founded by Korea government (MEST; no. 2012-0001112) and Technology Development Program for Agriculture and Forestry (308025-05-4-HD110), Ministry for Food, Agriculture, Forestry and Fisheries, Republic of Korea. All students were supported by BK21 program.
References
- Ziebuhr J. The coronavirus replicase. Curr Top Microbiol Immunol 2005;287:57–94
- Ratia K, Pegan S, Takayama J, et al. A noncovalent class of papain-like protease/deubiquitinase inhibitors blocks SARS virus replication. Proc Natl Acad Sci USA 2008;105:16119–24
- Devaraj SG, Wang N, Chen Z, et al. Regulation of IRF-3-dependent innate immunity by the papain-like protease domain of the severe acute respiratory syndrome coronavirus. J Biol Chem 2007;282:32208–21
- Ratia K, Saikatendu KS, Santarsiero BD, et al. Severe acute respiratory syndrome coronavirus papain-like protease: structure of a viral deubiquitinating enzyme. Proc Natl Acad Sci USA 2006;103:5717–22
- Barretto N, Jukneliene D, Ratia K, et al. The papain-like protease of severe acute respiratory syndrome coronavirus has deubiquitinating activity. J Virol 2005;79:15189–98
- Xiao G, Li G, Chen L, et al. Isolation of antioxidants from Psoralea corylifolia fruits using high-speed counter-current chromatography guided by thin layer chromatography-antioxidant autographic assay. J Chromatogr A 2010;1217:5470–6
- Guo JM, Weng XC, Wu H, et al. Antioxidants from a Chinese medicinal herb – Psoralea corylifolia L. Food Chem 2005;91:287–92
- Khatune NA, Islam ME, Haque ME, et al. Antibacterial compounds from the seeds of Psoralea corylifolia. Fitoterapia 2004;75:228–30
- Xu Q, Pan Y, Yi LT, et al. Antidepressant-like effects of psoralen isolated from the seeds of Psoralea corylifolia in the mouse forced swimming test. Biol Pharm Bull 2008;31:1109–14
- Choi YH, Yon GH, Hong KS, et al. In vitro BACE-1 inhibitory phenolic components from the seeds of Psoralea corylifolia. Planta Med 2008;74:1405–8
- Sun NJ, Woo SH, Cassady JM, Snapka RM. DNA polymerase and topoisomerase II inhibitors from Psoralea corylifolia. J Nat Prod 1998;61:362–6
- Oh KY, Lee JH, Curtis-Long MJ, et al. Glycosidase inhibitory phenolic compounds from the seed of Psoralea corylifolia. Food Chem 2010;121:940–5
- Hisashi M, Sachie K, Sachiko S, et al. Inhibition from the seeds of Psoralea corylifolia on production of nitric oxide in lipopolysaccharide-activated macrophages. Biol Pharm Bull 2009;32:147–9
- Bradford MM. A rapid and sensitive method for the quantization of microgram quantities of protein utilizing the principle of protein-dye binding Anal Biochem 1976;72:248–54
- Sulea T, Lindner HA, Purisima EO, Ménard, R. Deubiquitination, a new function of the severe acute respiratory syndrome coronavirus papain-like protease? J Virol 2005;79:4550–1
- Lindner HA, Fotouhi-Ardakani N, Lytvyn V, et al. The papain-like protease from the severe acute respiratory syndrome coronavirus is a deubiquitinating enzyme. J Virol 2005;79:15199–208
- Zhang JP, Chen QX, Song KK, Xie JJ. Inhibitory effects of salicylic acid family compounds on the diphenolase activity of mushroom tyrosinase. Food Chem 2006;95:579–84
- Chiari ME, Vera DMA, Palacios SM, Carpinella MC. Tyrosinase inhibitory activity of a 6-isoprenoid-substituend flavanone isolated from Dalea elegans. Bioorg Med Chem 2011;19:3474–82