Abstract
The biomimetic approach represents an interesting strategy for carbon dioxide (CO2) capture, offering advantages over other methods, due to its specificity for CO2 and its eco-compatibility, as it allows concentration of CO2 from other gases, and its conversion to water soluble ions. This approach uses microorganisms capable of fixing CO2 through metabolic pathways or via the use of an enzyme, such as carbonic anhydrase (CA, EC 4.2.1.1). Recently, our group cloned and purified a novel bacterial α-CA, named SspCA, from the thermophilic bacteria, Sulfurihydrogenibium yellowstonense YO3AOP1 living in hot springs at temperatures of up to 110 °C. This enzyme showed an exceptional thermal stability, retaining its high catalytic activity for the CO2 hydration reaction even after being heated at 70 °C for several hours. In the present paper, the SspCA was immobilized within a polyurethane (PU) foam. The immobilized enzyme was found to be catalytically active and showed a long-term stability. A bioreactor containing the “PU-immobilized enzyme” (PU-SspCA) as shredded foam was used for experimental tests aimed to verify the CO2 capture capability in conditions close to those of a power plant application. In this bioreactor, a gas phase, containing CO2, was put into contact with a liquid phase under conditions, where CO2 contained in the gas phase was absorbed and efficiently converted into bicarbonate by the extremo-α-CA.
Introduction
The increase of gases with greenhouse effect in the atmosphere including carbon dioxide (CO2) coming from the combustion of fossil materials, represents one of the leading factors of high impact on environmental stress and it is considered a major cause of climate changeCitation1. The production of “eco-compatible” combustible materials and/or the reduction of CO2 accumulation in the atmosphere represent the highest priority for a better quality of human life. A number of CO2 sequestration methods have been proposed in order to capture and concentrate CO2 from combustion gases, for its transit and storageCitation2. One of them is based on the chemical absorption of CO2 by alkanolamines and its subsequent stripping with steam, to produce a gas that can be transported to a geologic or marine sequestration siteCitation3,Citation4. This process is expensive and not convenient in all situations. The sequestration of CO2 as a salt is also interesting since the carbonate minerals represent the largest reserve of CO2 on earthCitation4. The latest years have seen the development of a biomimetic approach as an interesting strategy in CO2 capture process. The method offers important advantages if compared to the other methods for CO2 capturing since it is specific for CO2, is an eco-compatible process allowing the specific concentration of CO2 from other gases, its solubilization as ions and its further use for the growth of algae or other microorganisms. The biomimetic approach uses microorganisms capable of fixing CO2 through metabolic pathways or via the use of an enzyme, namely carbonic anhydrase (CA, EC 4.2.1.1)Citation5. Carbonic anhydrases are enzymes present in all domains of life, as they catalyze the interconversion between carbon dioxide and bicarbonate at neutral pHCitation6–16. Carbonic anhydrases (CAs, EC 4.2.1.1) are zinc-containing enzymes that catalyze the reversible conversion of carbon dioxide to bicarbonate and protons (CO2 + H2O⇋HCO + H+). The zinc ion is localized in the active site. Various classes (α, β, γ, δ and ζ) and isoforms of CA are described in literatureCitation17–19. Mammalian α-CAs are among the most active enzymes ever described with a turnover higher than 106 molecules of CO2 per secondCitation17–19. The use of carbonic anhydrases, either free or immobilized, has been reported in several technical approaches for capture of CO2 from combustion or from gases mixtures, for its subsequent utilization or disposalCitation5. The utilization of CA requires stable enzymes, even at high temperature, and the possibility of re-using the enzyme in many subsequent cycles for an efficient sequestration process. Recently, our group cloned and purified novel bacterial α-CAs from thermophilic bacteria, belonging to the genus Sulfurihydrogenibium living in hot springs all over the world, at temperatures up to 110 °CCitation20–25. The CAs of these bacteria are probably involved in the CO2 fixation and biosynthetic processes, as for other bacteria or as for algae and plants, in which this role of the various classes of CAs is well established. One of the discovered α-CA identified in the bacterial species Sulfurihydrogenibium yellowstonense YO3AOP1 and denominated SspCA, showed an exceptional thermal stability, retaining its high catalytic activity for the CO2 hydration reaction even after being heated at 70 °C for several hoursCitation20. SspCA was immobilized within a polyurethane (PU) foam and was found to be unalterably active and stable up to 50 h at 100 °CCitation20. In the present paper a bioreactor containing the “PU-immobilized enzyme” as shredded foam was realized, and experimental runs were carried out aimed to verify the CO2 absorption performance in working conditions typical of a power plant application. In the bioreactor, a gas phase, containing CO2, was put in contact with a liquid phase under conditions where the CO2 contained in the gas phase is absorbed by the liquid phase and converted into bicarbonate by carbonic anhydrase. The results obtained in this work strongly suggest that the thermostable SspCA is an almost ideal candidate for hydration of CO2 in biomimetic sequestration.
Materials and methods
Preparation of the recombinant protein
Competent E. coli BL21 (DE3) cells were transformed with pET15b (Novagen) containing the Sulfurihydrogenibium yellowstonense gene encoding α-CA lacking the signal peptide (first 21 amino acid of the peptide sequence) and with a polyhistidine-tag at N-terminus of the protein. Cells were grown at 37 °C and induced with 1 mM IPTG. After additional growth for 5 h, the cells were harvested and disrupted by sonication at 4 °C. Following centrifugation, the cell extract was heated at 90 °C for 30 min and centrifuged. The supernatant was loaded into His-select HF Nickel affinity gel and the protein was eluted with 250 mM imidazole. At this stage of purification the enzyme was at least 95% pure. All the details concerning the expression and purification of SspCA have been described by Capasso et al.Citation20.
Enzyme immobilization
The enzyme was immobilized following the procedure described by OzdemirCitation26. Briefly, 10 mg of SspCA were dissolved in 3 mL of distilled water and poured onto about 3 g of viscous HYPOL2060 prepolymer (a gift from Dow Chemical Co., Italy) in a 50 mL falcon tube. The solution was mixed vigorously for 30 s to achieve a homogeneous distribution of the enzyme within the prepolymer resulting in the enzyme immobilization of SspCA (PU-SspCA). The solution started to polymerize as a result of a CO2 release during the polymerization, which developed in 3–5 min. The polymer solution was left at room temperature for an additional 15 min before being used. After completion of the enzyme immobilization, a piece of foam containing the immobilized enzyme was cut and assayed for CA activity.
Activity assay for immobilized enzyme
CA activity was determined on a piece of CA-immobilized foam (3 mg of foam containing 10 μg of SspCA), which was cut from the middle of the whole product. The assay was based on the monitoring of pH variation due to the catalyzed conversion of CO2 to bicarbonate and protons. The reaction was performed at 0 °C adding 1.0 mL ice-cold CO2-saturated water to 1.0 mL mixtures of 25 mM Tris-SO4 buffer. CO2 conversion was started by adding different amounts of immobilized enzyme or free enzyme or foam without enzyme. The CO2-satured solution was prepared by bubbling CO2 into 100 mL distilled water for approximately 3 h. The CO2 solution was chilled in an ice water bath. One milliliter of CO2 solution was added very quickly and simultaneously a stopwatch was started. The time required for the solution to change from blue to yellow was recorded (transition point of bromothymol blue is pH 6–7.6). The time required for the color change is inversely related to the quantity of carbonic anhydrase present in the sample. Wilbur-Anderson units were calculated according to the following definition: One Wilbur-Anderson unit (WAU) of activity is defined as (T0 − T)/T, where T0 (uncatalyzed reaction) and T (catalyzed reaction) are recorded as the time (in seconds) required for the pH to drop from 8.3 to the transition point of the dye in a control buffer and in the presence of the immobilized enzyme, respectively.
CO2 absorption capability
The CO2 absorption capability of the immobilized SspCA was verified by experimental tests carried out in conditions closer to those of a post-combustion power plant application. The experiments were realized using a laboratory plant based on a three-phase trickle-bed reactor (). The feeding section was composed of gas cylinders for N2 and CO2/N2 mixture (20% by volume); a KNF SIMDOS pump for bi-distilled water delivering; Teflon pipelines (4 mm i.d.) for all reactants. The reactor (length 60 cm, i.d. 4 cm), realized in Pyrex glass, was designed according to the three-phase trickle-bed configuration, and was able to operate in both cocurrent downflow and countercurrent flowCitation27. Two porous septa (40–100 μm) were used to separate gas and liquid currents at both inlet and outlet, with the lower septum working also as a packed bed support. In the countercurrent operation () the measuring pump allowed the bi-distilled water to be fed from the top, while two regulating valves located at the reactor outlet were used to collect the CO2 aqueous solution and to assure the presence of liquid at the reactor bottom, necessary to balance the pressure drop through the catalytic bed during countercurrent operation. In the concurrent down flow procedure the gaseous stream came out from the reactor bottom together with the CO2 aqueous solution. In both cases the CO2 concentration analysis was performed by an on-line ABB URAS 17 analyzer, equipped with IR measuring cell, which made necessary the utilization of a condenser at 0 °C (2.5 l) to remove water before the analyzer. Two mass flow controllers were adopted for gaseous streams, a pressure sensor was located upstream the reactor, and a temperature sensor upstream the condenser. The spongy nature of the catalytic solid (carbonic anhydrase immobilized on polyurethane foam) did not permit the catalytic bed to be packed with a predefined grain size, then in order to limit the pressure droop through the bed the amount of absorbent was mixed with a similar volume of an inert material (glass spheres of about 2 mm e.d.). The catalytic bed was then loaded inside the reactor between two layers of the same material. A gaseous mixture of CO2 in N2 was fed to the reactor in both cocurrent downflow and countercurrent flow with bi distilled water, the CO2 concentration was fixed at 20% by volume since this value corresponds to the average concentration present at the exhaust of power plants.
Results
The recombinant SspCA was isolated and purified to homogeneity at room temperature from Escherichia coli (DE3) cell extract. Most of the carbonic anhydrase activity was recovered in the soluble fraction of E. coli cell extract after sonication and centrifugation. The heterologously expressed SspCA enzyme was purified three-fold with the thermoprecipitation step. Using the affinity column (His-select HF Nickel affinity gel), the SspCA was purified 16-fold to apparent homogeneityCitation20. Enzyme immobilization was performed as described in “Materials and methods” section. The calculated specific activity of the PU-SspCA was similar to that of the free enzyme (). Moreover, the dry polyurethane foam containing the immobilized SspCA or the free enzyme were stored at 25 °C for several days. The free enzyme was stored in a buffer solution of 10 mM Tris/HCl, pH 8.3. The PU-SspCA specific activity was measured after 1, 6 and 30 days and compared with that of the free enzyme (). After one month, PU-SspCA specific activity remained constant while that of the free enzyme decreased slightly as expected for an enzyme from a thermophilic bacterium.
Table 1. Comparison of the hydratase activity for the immobilized and free enzyme incubated at 25°C for several days.
The three-phase reactor was filled with shredded foam composed of PU-SspCA and PU, in order to evaluate the absorption capability in presence and absence of the catalytic effect. The CO2 conversion efficiency for the uncatalyzed reaction resulted 2.3% in a test performed in the following conditions: 50 g of foam, gas flow rate = 1 l/min, water flow rate = 50 ml/min, T = 25 °C, P = 1.1 bar. This efficiency value corresponds to the CO2 distribution between gaseous and aqueous phases, regulated by the Henry law (keq = 10–1.5), in the given conditions, while the successive hydration reaction needs the intervention of a catalyst. The results of experimental tests carried out on the immobilized carbonic anhydrase are reported in –, where the CO2 conversion efficiency is reported as function of time on stream. The absorption capability was characterized varying both gas and water flow rate in the two reactor working modalities. Because of dead volumes present between reactor and analysis section (in particular condenser and pipelines, ) a stationary value of CO2 concentration at the reactor outlet was reached at different times on stream, in dependence of flow conditions. The details of experimental conditions relating to each test are specified in legends. shows the effect of water flow rate on CO2 conversion efficiency for a gas flow rate of 0.5 l/min in cocurrent downflow. The positive effect of the catalyst on CO2 conversion did not result very significant up to 50 ml/min (conversion increased from 8% at 25 ml/min up to 15% at 50 ml/min), while an evident performance improvement was observed at water flow rate of 75 and 100 ml/min (30 and 45%, respectively). The effect of gas flow rate was then evaluated for a water flow rate of 50 ml/min in cocurrent downflow, and the relating results are shown in . For the fixed water flow rate the CO2 conversion efficiency increased from 4 to 28% lowering the gas flow rate from 1.5 to 0.1 l/min. The overall results of and show that the CO2 capture performance of the immobilized carbonic anhydrase, when tested by a three-phase trickle-bed reactor, can be optimized by regulation of the reactor feed ratio. In particular the increase of water flow rate improves the CO2 conversion by affecting the mass transfer process of CO2 from gas to liquid phase, which is the rate limiting step in the presence of a catalystCitation28, while the lowering of gas flow rate improves the contact time between solid and gas phase. The effect of the reactor working modality is shown in , where the CO2 conversion is plotted versus time on stream for experiments carried out in both cocurrent downflow and countercurrent flow. It can be noticed that in spite of a water flow rate lower than those used for the experiments of (10 ml/min), the CO2 conversion in countercurrent modality resulted significantly higher with respect to the cocurrent operation (38% and 6%, respectively), confirming the role of feed ratio and water flow rate on performance obtainable by a three-phase trickle-bed reactor.
Figure 2. Test of CO2 biomimetic absorption by three-phase trickle-bed reactor. Effect of water flow rate on CO2 conversion efficiency as function of time on stream. Amount of catalyst = 25 g. Gas flow rate = 0.5 l/min. T = 25 °C. P = 1.1 bar.
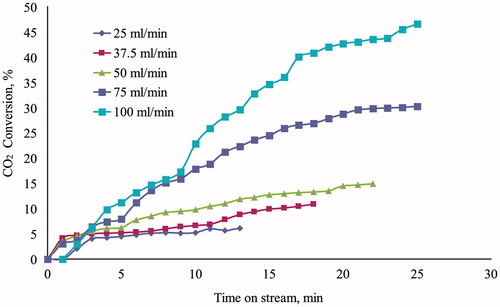
Figure 3. Test of CO2 biomimetic absorption by three-phase trickle-bed reactor. Effect of gas flow rate on CO2 conversion efficiency as function of time on stream. Amount of catalyst = 25 g. Water flow rate = 50 ml/min. T = 25 °C. P = 1.1 bar.
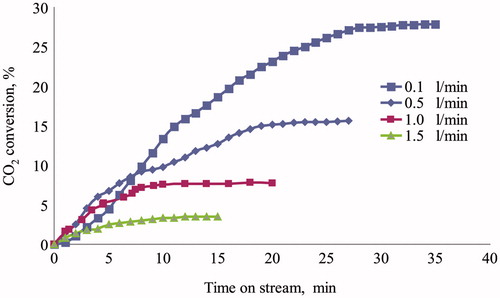
Figure 4. Test of CO2 biomimetic absorption by three-phase trickle-bed reactor. Effect of reactor operative condition (cocurrent downflow vs countercurrent flow) on CO2 conversion efficiency as function of time on stream. Amount of catalyst = 25 g. Gas flow rate = 0.1 l/min. Water flow rate = 10 ml/min. T = 25 °C. P = 1.1 bar.
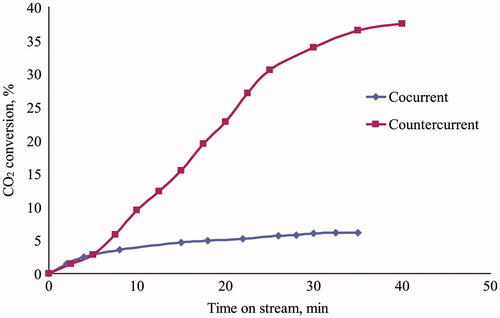
Discussion and conclusions
CO2 dissolves rapidly in water producing a hydrated, unstable form (carbonic acid) that can react, at pH higher than 10, according to the following equations:
(Equation n. 1): CO2 + H2O = H2CO3;
(Equation n. 2): H2CO3 = HCO3-+ H+;
(Equation n. 3): HCO3-= CO32-+ H+
Among these reactions, the hydration of CO2 (Equation 1) is the slowest step. When the pH is higher than 10, equation 3 dominates over the bicarbonate formation (Equation n. 2); when the pH is less than 8, equation 3 is negligible. At a moderate pH, we can fix a large amount of CO2 as carbonate only speeding up reaction 1. This has been demonstrated by using carbonic anhydrases of human and bovine originCitation29–31. There is however a search for CAs, which are more stable and more active in the operative conditions and, in addition, enzymes that could be produced in large amounts at low purification costs. With the technologies available on the market it is possible to produce large amounts of enzymes at moderate costs as happens, for example, for the enzymes utilized in detergents plantsCitation32–34. Generally, CAs isolated from mammals or prokaryotes are active at physiological temperatures and are, as many enzymes, quite unstable under or extreme conditions, such as high temperature, high concentrations of salts, etc. Taking into account the biodiversity, studies of enzymes from thermophiles, i.e., microorganisms living at temperatures ranging from 70 °C to 110 °C, have demonstrated that these enzymes are thermostable, thermoactive and generally stable to the common enzyme denaturantsCitation35,Citation36. In fact, the temperature of combustion gasses or liquids into which the CO2 are dissolved may, however, easily exceed the optimal temperature for the carbonic anhydrase used to capture the CO2. One of the drawbacks of using enzymes based approaches is that an extensive cooling it is necessary before adding the enzyme to gas or liquid containing CO2, and cooling is an energy consuming process. These findings strongly support the utilization of “thermostable-α-CAs” like SspCA fin the CO2 capture process.
We investigated an α-CA from the thermophilic bacterium Sulfurihydrogenibium yellowstonense YO3AOP1 which is catalytically very active for the physiological reaction of CO2 hydration to bicarbonate and protons, and was shown earlier to be highly thermostableCitation20. Here we report the immobilization of this enzyme, SspCA, with polyurethane (HYPOL2060). The SspCA covalently bound to polyurethane showed the following characteristics: a) SspCA preserves its activity; b) SspCA enhances its stability when incubated at room temperature for long time (1 month); c) SspCA shows a good CO2 capture performance when the PU-SspCA shredded foam is used in the bioreactor reported in the present paper. Moreover, in a previously work we have demonstrated that SspCA was not substantially inhibited by bicarbonate and carbonate, and hydrogen sulfiteCitation21. The lack of strong affinity for bicarbonate, carbonate and also hydrogen sulfide, make this enzyme an interesting candidate for biotechnological applications of enzymatic CO2 fixation.
Declaration of interest
This research was financed by a grant of the “Accordo di Programma CNR-MSE”. The authors declare no conflict of interest.
References
- Huesemann MH, Hausmann TS, Bartha R, Aksoy M, Weissman JC, Benemann JR. Biomass productivities in wild type and pigment mutant of Cyclotella sp. (Diatom). Appl Biochem Biotechnol 2009;157:507–526
- Gentzis T. Subsurface sequestration of carbon dioxide; an overview from an Alberta (Canada) perspective. International Journal of Coal Geology. 2000;43:287–305
- Greer T, Bedelbayev A, Igreja JM, Gomes JF, Lie B. A simulation study on the abatement of CO2 emissions by de-absorption with monoethanolamine. Environ Technol 2010;31:107–115
- Mignardi S, De Vito C, Ferrini V, Martin RF. The efficiency of CO2 sequestration via carbonate mineralization with simulated wastewaters of high salinity. J Hazard Mater 2011;191:49–55
- Bond GM, Stringer J, Brandvold DK, Simsek FA, Medina MG, Egeland G. Development of integrated system for biomimetic CO2 sequestration using the enzyme carbonic anhydrase. Energy Fuels. 2001;15:309–16
- Supuran CT. Structure-based drug discovery of carbonic anhydrase inhibitors. J Enzyme Inhib Med Chem. 2012
- Supuran CT. Carbonic anhydrase inhibitors as emerging drugs for the treatment of obesity. Expert Opin Emerg Drugs 2012;17:11–15
- Supuran CT. Inhibition of bacterial carbonic anhydrases and zinc proteases: from orphan targets to innovative new antibiotic drugs. Curr Med Chem 2012;19:831–844
- Neri D, Supuran CT. Interfering with pH regulation in tumours as a therapeutic strategy. Nat Rev Drug Discov 2011;10:767–777
- Supuran CT. Carbonic anhydrases: novel therapeutic applications for inhibitors and activators. Nat Rev Drug Discov 2008;7:168–181
- Supuran CT, Scozzafava A, Casini A. Carbonic anhydrase inhibitors. Med Res Rev. 2003;23:146–89
- Supuran CT, Scozzafava A, Clare BW. Bacterial protease inhibitors. Med Res Rev 2002;22:329–372
- Winum JY, Poulsen SA, Supuran CT. Therapeutic applications of glycosidic carbonic anhydrase inhibitors. Med Res Rev 2009;29:419–435
- Winum JY, Rami M, Scozzafava A, Montero JL, Supuran C. Carbonic anhydrase IX: a new druggable target for the design of antitumor agents. Med Res Rev 2008;28:445–463
- Winum JY, Scozzafava A, Montero JL, Supuran CT. Sulfamates and their therapeutic potential. Med Res Rev 2005;25:186–228
- Winum JY, Scozzafava A, Montero JL, Supuran CT. Therapeutic potential of sulfamides as enzyme inhibitors. Med Res Rev 2006;26:767–792
- Supuran CT, Di Fiore A, Alterio V, Monti SM, De Simone G. Recent advances in structural studies of the carbonic anhydrase family: the crystal structure of human CA IX and CA XIII. Curr Pharm Des. 2010;16:3246–54
- Supuran CT. Carbonic anhydrase inhibition with natural products: novel chemotypes and inhibition mechanisms. Mol Divers 2011;15:305–316
- Supuran CT. Carbonic anhydrase inhibitors and activators for novel therapeutic applications. Future Med Chem 2011;3:1165–1180
- Capasso C, De Luca V, Carginale V, Cannio R, Rossi M. Biochemical properties of a novel and highly thermostable bacterial a-carbonic anhydrase from Sulfurihydrogenibium yellowstonense YO3AOP1. J Enzyme Inhib Med Chem 2012;27:892–897
- De Luca V, Vullo D, Scozzafava A, Carginale V, Rossi M, Supuran CT et al Anion inhibition studies of an a-carbonic anhydrase from the thermophilic bacterium Sulfurihydrogenibium yellowstonense YO3AOP1. Bioorg Med Chem Lett 2012;22:5630–5634
- De Luca V, Vullo D, Scozzafava A, Carginale V, Rossi M, Supuran CT et al An a-carbonic anhydrase from the thermophilic bacterium Sulphurihydrogenibium azorense is the fastest enzyme known for the CO2hydration reaction. Bioorg Med Chem 2012; doi: 10.1016/j.bmc.2012.09.047 (e-pub ahead of print)
- Vullo D, De Luca V, Scozzafava A, Carginale V, Rossi M, Supuran CT et al Anion inhibition studies of the fastest carbonic anhydrase (CA) known, the extremo-CA from the bacterium Sulfurihydrogenibium azorense. Bioorg Med Chem Lett 2012;22:7142–7145
- Vullo D, De Luca V, Scozzafava A, Carginale V, Rossi M, Supuran CT, et al The first activation study of a bacterial carbonic anhydrase (CA). The thermostable alpha-CA from Sulfurihydrogenibium yellowstonense YO3AOP1 is highly activated by amino acids and amines. Bioorg Med Chem Lett. 2012;22:6324–6327
- Vullo D, De Luca V, Scozzafava A, Carginale V, Rossi M, Supuran CT et al The alpha-carbonic anhydrase from the thermophilic bacterium Sulfurihydrogenibium yellowstonense YO3AOP1 is highly susceptible to inhibition by sulfonamides. Bioorg Med Chem 2012; doi: 10.1016/j.bmc.2012.07.024 (e-pub ahead of print)
- Kanbar B, Ozdemir E. Thermal stability of carbonic anhydrase immobilized within polyurethane foam. Biotechnol Prog 2010;26:1474–1480
- Dudukovic MP, Larachi F, Mills PL. Multiphase reactors-revisited. Chem Eng Sci. 1999;54:1975–95
- Dilmore R, Griffith C, Liu Z, Soong Y, Hedges SW, Koepsel R, et al Carbonic anhydrase-facilitated CO2 absorption with polyacrylamide buffering bead capture. Int J Greenh Gas Con. 2009;3:401–410
- Vinoba M, Lim KS, Lee SH, Jeong SK, Alagar M. Immobilization of human carbonic anhydrase on gold nanoparticles assembled onto amine/thiol-functionalized mesoporous SBA-15 for biomimetic sequestration of CO2. Langmuir 2011;27:6227–6234
- Vinoba M, Bhagiyalakshmi M, Jeong SK, Nam SC, Yoon Y. Carbonic anhydrase immobilized on encapsulated magnetic nanoparticles for CO2 sequestration. Chemistry 2012;18:12028–12034
- Zhang S, Zhang Z, Lu Y, Rostam-Abadi M, Jones A. Activity and stability of immobilized carbonic anhydrase for promoting CO2 absorption into a carbonate solution for post-combustion CO2 capture. Bioresour Technol 2011;102:10194–10201
- Quyen TD, Vu CH, Le GT. Enhancing functional production of a chaperone-dependent lipase in Escherichia coli using the dual expression cassette plasmid. Microb Cell Fact 2012;11:29
- Yang H, Liu L, Li J, Du G, Chen J. Heterologous expression, biochemical characterization, and overproduction of alkaline a-amylase from Bacillus alcalophilus in Bacillus subtilis. Microb Cell Fact 2011;10:77
- Joseph B, Ramteke PW, Thomas G. Cold active microbial lipases: some hot issues and recent developments. Biotechnol Adv 2008;26:457–470
- Myers JK, Pace CN, Scholtz JM. Denaturant m values and heat capacity changes: relation to changes in accessible surface areas of protein unfolding. Protein Sci 1995;4:2138–2148
- Kumar S, Nussinov R. How do thermophilic proteins deal with heat? Cell Mol Life Sci 2001;58:1216–1233