Abstract
In this article, we describe that mononuclear complexes composed of (5-chloro-2-hydroxybenzylidene)aminobenzenesulfonamides (L1–3) of general formula (L2(M)2H2O, where M is Co, Cu, Zn, Ni or Mn) reduced epimastigote proliferation and were found cidal for trypomastigotes of Trypanosoma cruzi Y strain. Complexes C5 and C11 have IC50 of 2.7 ± 0.27 and 4.8 ± 0.47 µM, respectively, for trypomastigotes, when the positive control Nifurtimox, which is also an approved drug for Chagas disease, showed IC50 of 2.7 ± 0.25 µM. We tested whether these complexes inhibit the enzyme T. cruzi trypanothione reductase or acting as DNA binders. While none of these complexes inhibited trypanothione reductase, we observed some degree of DNA binding, albeit less pronounced than observed for cisplatin in this assay. Unfortunately, most of these complexes were also toxic for mouse splenocytes. Along with the present studies, we discuss a number of interesting structure–activity relationships and chemical features for these metal complexes, including computational calculations.
Introduction
Trypanosoma cruzi, the parasitic protozoan agent of Chagas disease or American trypanosomiasis, affects about 17 million people, and around 100 million are at risk of infection across AmericaCitation1. T. cruzi infection initially leads to a generally mild acute phase, followed by a long but asymptomatic phase, while during the time it is estimated around 30% infected patients develop the symptomatic chronic phase of Chagas disease. It is characterized by the presence of myocarditis (often called chagasic cardiomyopathy) and in some cases, pathological disorders of the peripheral nervous and gastrointestinal systemsCitation2,Citation3. Benznidazole is the only chemotherapeutic agent used for the treatment, but it has issues relating to toxicity and limited efficacy during the chronic phase of the diseaseCitation4. As a consequence, it is essential to discover and identify novel therapies that could fight more aggressively as anti-T. cruzi agents.
A number of studies have proved the usefulness of metal complexes as an important chemical class of anti-T. cruzi agentsCitation5. The DNA structure was the first drug target recognized for anti-trypanosomal metal complexesCitation6, but other drug targets of T. cruzi were recently identified, such as the cysteine protease cruzainCitation7, trypanothione reductaseCitation8, NADH-fumarate reductaseCitation9 and also the biochemical path of nitric oxideCitation10. In the search for new therapeutic agents, the incorporation of a metallic atom into anti-T. cruzi compounds can lead to metal complexes with a strong anti-trypanosomal effect on cell culturesCitation11,Citation12. From the structure–activity studies of these metal complexes, it appeared that increase in the hydrophobicity of the resulting complexes is the cause for the potency enhancement of the anti-trypanosomal effectsCitation13–16.
In this paper, we report the evaluation of metal complexes bearing a sulfonamide moiety. We used the sulfonamides, denoted L1–3 as the ligand to form metal complexation. We decided to use a sulfonamide group on L1–3 because of the plenty of works highlighting their pharmacological potential as therapeutic agentsCitation17,Citation18. For instance, sulfonamides are important structural component of the carbonic anhydrase inhibitors, which are important drug targets in widespread pathological conditionsCitation19,Citation20. Besides, they undergo chemical transformations in physiological medium and therefore behaving as prodrugsCitation21.
We report here that while the metal-free ligands L1–3 did not have potent trypanosomicidal activity, we noted the striking ability of the metal complexes in inhibiting epimastigote proliferation and showing toxicity for trypomastigotes of Y strain T. cruzi. A detailed computational study and attempt to elucidate the action mechanism were performed for these metal complexes. Emphasizing these studies could potentially offer further mechanism of drug designing in this significant area of research for the next generation of anti-T. cruzi metal complexes. For the accomplishment of this task, the sulfonamides and their metal-based (cobalt, copper, nickel or zinc) compounds were synthesized as described in and 22,Citation23, by generating the respective mononuclear chelated complexes of metal:ligand ratio of 1:2, and having the general formula [M(L1–3)2(H2O)2].
All metallo-sulfonamides were evaluated against Y strain T. cruzi. We first measured cell viability of mouse splenocytes treated with metal-free ligand L1–3 and their complexes, with determination of highest non-toxic concentration. Once the toxicity for mouse splenocytes was measured, we tested these compounds against epimastigotes (axenic culture) and for bloodstream trypomastigotes of Y strain T. cruzi, using Nifurtimox as a control drug. The ability of representative of these metallic compounds to inhibit the enzymatic activity of cruzain or trypanothione reductase of T. cruzi was also performed in parallel to the parasite cell assays. summarizes the enzymatic, trypanosomicidal activity as well as the toxicity to splenocytes.
Table 1. Antiprotozoal activity, toxicity and enzyme inhibition for the sulfonamides and their metal complexes.
Nifurtimox (Nfx) displayed an IC50 value of 2.75 and 1.8 µM for trypomastigotes and epimastigotes, respectively. These IC50 values we found for Nifurtimox were reliable, since in our previous works, we have generally observed IC50 values below 5.0 µMCitation24. The metal-free ligands L1–3 were far less potent than Nifurtimox, at least fivefold.
Having ascertained the IC50 values for L1–3 and Nifurtimox, we moved forward and tested the metal complexes. We found very promising trypanosomicidal activities for the metal complexes prepared from the sulfonamide compounds L1–3. The complexes C1, C5, C7 and C11 showed pronounced antiproliferative activity for the replicative form of epimastigote as well as toxicity for the infective form trypomastigote of Y strain T. cruzi. For example, complexes C5 and C11 have IC50 of 2.7 and 4.8 µM for trypomastigotes Y strain, when our positive control Nifurtimox showed IC50 of 2.7 µM. Although these complexes were less toxic for mouse splenocytes than gentian violet (positive control), significant toxicity for mouse splenocytes was observed for metal complexes when we compared to that observed for Nifurtimox ().
With the intention of broadening our understanding on the functional activity of metal complexes on T. cruzi, we selected and tested some of these compounds against the recombinant cysteine protease of T. cruzi cruzain as well as against T. cruzi trypanothione reductase. The complexes were used at concentrations of 50 and 100 µM in the enzymatic assays with trypanothione reductase and cruzain, respectively (data not shown). We did not observe any substantial inhibition of catalytic activity for these metal complexes neither for the metal-free ligand L1 against trypanothione reductase and cruzain. We were surprised with these results, because these enzymes have been largely studied as important T. cruzi molecular targetsCitation24,Citation25 and their well-known metallic compounds are reported as inhibitors of these enzymes. Because of the findings in the literature, a possibility is that these compounds may inhibit T. cruzi-carbonic anhydrase.
Finally, we attempted to see whether these complexes bind to DNA. Using an UV-Vis-based titration assayCitation26, we observed that complexes C3 and C11 strongly interact with calf-thymus DNA in a time- and dose–response way (data not shown). But we cannot state that there is a clear correlation between DNA binding and trypanosomicidal activity, so we might suggest that the DNA pathway can be a way for further investigation of these trypanosomicidal complexes.
With the intent of broadening our understanding of anti-T. cruzi activity of metal complexes, we decided to perform an extensive work of structural characterization using theoretical calculation.To our knowledge, structural data of complexes 1–12 are absent in the literature. From our DFT calculations, complexes 1–12 were found to have approximate C2 symmetry in which the metal centre (Co, Ni, Cu and Zn) is coordinated in a pseudo-octahedral fashion. In particular, the metal centre is located at a centre of inversion and coordinated by two chelating Ln (n = 1, 2 or 3) ligands in the equatorial plane and by two water molecules (hereafter W1 and W2) in the axial positions. The ligands coordinated to metal in their nonprotonated form leads to a six-membered chelation ring. In the equatorial plane, O atoms (N atoms) are trans to each other. A molecular representation of compounds 1–12 and the corresponding atom-labeling scheme are given in and . Some relevant bond distances around the metal centre are presented in . NBO and APT charges as well as spin densities on the metal centre are also given in .
Figure 3. Top: Calculated molecular structure of complexes C1--4. Central “M” spheres represent metal (Co, Ni, Cu or Zn). W1 and W2 describe oxygen atoms belonging to water molecules. Bottom: Calculated molecular structure of complexes C5--8.
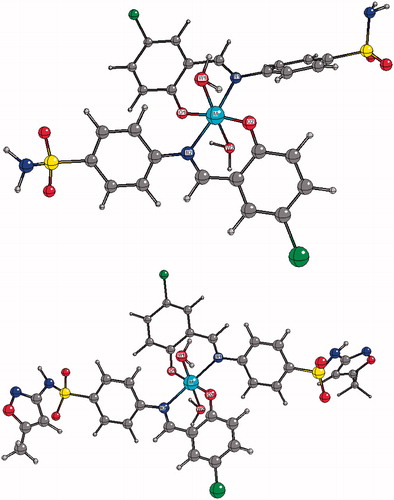
Table 2. Calculated geometric and electronic parameters for complexes C1–12.
Due to an approximate C2 symmetry, the M–O1 and M–O2 bond distances are very similar to each other in all complexes. They differ by no more than 0.02 Å. A similar finding is observed for M–N1 and M–N2 bond distances. Nevertheless, differences of up to 0.1 Å are found when comparing M–W1 and M–W2 bond distances for a given complex. The M–O and M–N bond distances in complexes 1–12 are depend on the nature of the ligands (L1, L2 or L3). For all complexes, M–O bond distances are found to be slightly shorter than the corresponding M–N bond. We also observed that, for all ligands, the M–O bond length increases in the following order: Cu–O < Co–O < Ni–O < Zn–O. The corresponding sequence for the M–N bond distances is Cu–O < Ni–O < Co–O < Zn–O. It can be observed that for a given metal, on passing from L1 to L2, the M–X (X = O or N) bond length is virtually unchanged. The only exception is observed for the Co–N bond, in which there is a decrease of 0.03 Å. In any case, in going from L2 to L3 the M–O bonds increase slightly while an opposite effect is observed for the M–N ones.
Main bond angles around the metal centre are listed in . We also inferred that in passing from L1 to L2, O1–M–N1 and N2–M–O2 bond angles do not change significantly. The largest increasing of about 1.7° is predicted for O2–Co–N2 bond angle. However, all chelation bond angles increased with the replacement of L2 by L3. The largest changing was predicted for O2–Zn–N2 angle (3.8°). Our calculations also reveal significant intramolecular hydrogen bonding between the hydrogen atom from the water molecule W1 and the oxygen atom O2 (W1H–O2) and other hydrogen bonding between the hydrogen atom from W2 and the oxygen atom O1 (W2H–O1).
Table 3. Calculated bond angles (°) and intramolecular hydrogen bonds (Å) for complexes C1–12.
We also calculated NPA and APT charges (). For a given ligand, APT charges on metal are found to increase monotonically in the order Cu < Ni < Co < Zn. NBO scheme predicts the same trend, but they gave a similar charge distribution for both Ni and Co centres.
Experimental section
Synthesis: Ligands L1–3 and their metal complexes were previously prepared and fully characterized by spectral analysisCitation22,Citation23.
Pharmacological evaluation
Parasites: Epimastigotes of T. cruzi (Y strain) were maintained at 26 °C in LIT medium (Liver Infusion Tryptose) supplemented with 10% fetal calf serum (FCS; Cultilab, Campinas, SP, Brazil), 1% hemin (Sigma Chemical Co., St. Louis, MO), 1% R9 medium, and 50 µg/mL of gentamycin (Novafarma, Anápolis, GO, Brazil). Bloodstream trypomastigotes forms of T. cruzi were obtained from supernatants of LLC-MK2 cells previously infected and maintained in the RPMI-1640 medium (Sigma) supplemented with 10% FBS, 1% hemin, 1% R9 medium and 50 mg/mL gentamycin at 37 °C and 5% CO2.
Cytotoxicity to mice splenocytes: BALB/c mice splenocytes were placed into 96-well plates at a cell density of 5 × 106 cells/well in the RPMI-1640 medium supplemented with 10% of FCS and 50 µg/mL of gentamycin. Each test inhibitor was used in five concentrations (1.23, 3.70, 11.11, 33.33 and 100 μg/mL) in triplicate. To each well, an aliquot of test inhibitor suspended in DMSO was added, in addition to wells only containing either solvent (untreated cells) or gencian violet (drug control). Then, the plate was incubated for 24 h at 37 °C and 5% CO2. After incubation, 1.0 µCi of 3H-thymidine (Perkin Elmer, Waltham, MA) was added to each well, and the plate was returned to the incubator. The plate was then transferred to a beta-radiation counter (Multilabel Reader, Finland), and the percent of 3H-thymidine was determined. Cell viability was measured as the percent of 3H-thymidine incorporation for treated-cells in comparison to untreated cells.
Antiproliferative activity for epimastigotes: Epimastigotes were counted in a hemocytometric and then dispensed into 96-well plates at a cell density of 106 cells/well. Test inhibitors, dissolved in DMSO, were diluted into five different concentrations (1.23, 3.70, 11.11, 33.33 and 100 μg/mL) and added to the respective wells in triplicate. The plate was incubated for 11 days at 26 °C, and aliquots of each well were collected and the number of viable parasites were counted in a Neubauer chamber, and compared to untreated parasite culture. IC50 calculation was carried out using a nonlinear regression on GraphPad Prism version 5.00 for Windows, GraphPad Software (San Diego, CA). This experiment was done in duplicate, and Nifurtimox (Nfx) was used as the reference inhibitor.
Toxicity for trypomastigotes: Trypomastigotes collected from the supernatants of LLC-MK2 cells were dispensed into 96-well plates at a cell density of 4 × 105 cells/well. Test inhibitors, dissolved in DMSO, were diluted into five different concentrations and added into their respective wells, and the plate was incubated for 24 h at 37 °C and 5% of CO2. Aliquots of each well were collected and the number of viable parasites, assessed as parasite motility, was counted in a Neubauer chamber. The percentage of inhibition was calculated in relation to untreated cultures. IC50 calculation was also carried out using a nonlinear regression on Prism 4.0 GraphPad software. This experiment was repeated once, and Nifurtimox (Nfx) was used as the reference inhibitor.
Inhibition of recombinant trypanothione reductase: The enzymatic activity was measured spectrophotometrically at 25 °C in protein assay buffer [40 mM N-(2-hydroxyethyl)piperazine-N′-ethanesulfonic acid (Hepes), 1 mM EDTA, pH 7.5] as previously describedCitation8. Stock solutions of the complexes were dissolved in DMSO. The assay mixtures (1 mL) contained 100 mM NADPH, 100 mM of trypanothione disulfide (TS2) and various concentrations of the inhibitor. NADPH, enzyme and inhibitor were mixed. The reaction was started by adding TS2 and the absorption decrease at 340 nm due to NADPH consumption was followed. Control assays contained the respective amount of DMSO instead of inhibitor.
Computational calculations: Electronic structure calculations were performed using the Gaussian 03 quantum chemistry softwareCitation27. DFT calculations were carried out using de Becke’s three-parameters functional and the correlation function of Lee, Yang and Parr (B3LYP)Citation28,Citation29. A pseudo-potential LANL2DZ basis setCitation30 was used to describe the metallic centres, whereas all electron 6-31G(d,p) was used for main group elements. The corresponding valence basis sets for Co, Ni, Cu and Zn were augmented by an f-type polarization function with the coefficients ξ = 2.780, 3.130, 3.525 and 3.031, respectivelyCitation31,Citation32. All geometry optimizations were performed without any symmetry constraints. Stationary points were characterized by the calculation of vibrational frequencies. We calculated net atomic charges via two approaches: natural population analysisCitation33 and from the atomic polar tensorCitation34. A MOLDRAW33 program was used to draw the molecular structuresCitation35.
Conclusion
Overall, we have demonstrated that the complexation of bioactive sulfonamides with transition metals leads to a new set of anti-T. cruzi agents with an attractive range of efficacy against the aforementioned parasite. Structure–activity relationships, chemical reactivity as well predicted geometry we determined here in details for complexes C1–12 might be used for further structural design of complexes as well to explain the difference of pharmacological property between different used metals.
Declaration of interest
This work received funding by CNPq and FACEPE.
Acknowledgements
We are in debt with Prof. R. Luise Krauth-Siegel for performing the enzymatic assays.
References
- Coura JR. Chagas disease: what is known and what is needed – a background article. Mem Inst Oswaldo Cruz 2007;102:113–22
- Soares MBP, Pontes-De-Carvalho L, Ribeiro-dos-Santos R. The pathogenesis of Chagas’ disease: when autoimmune and parasite-specific immune responses meet. Acad Bras Cienc 2001;73:547–59
- Antonio Marin-Neto SJ, Cunha-Neto E, Maciel BC, Simões MV. Pathogenesis of chronic Chagas heart disease. Circulation 2007;115:1109–23
- Coura JR, Castro SL. A critical review on Chagas disease chemotherapy. Mem Inst Oswaldo Cruz 2000;97:3–21
- Fricker SP. Metal based drugs: from serendipity to design. Dalton Trans 2007;43:4903–17
- Sanchez-Delgado RA, Anzellotti A. Metal complexes as chemotherapeutic agents against tropical diseases: trypanosomiasis, malaria and leishmaniasis. Mini Rev Med Chem 2004;4:23–30
- Caffrey CR, Scory S, Steverding D. Cysteine proteinases of trypanosome parasites: novel targets for chemotherapy. Curr Drug Targets 2000;1:155–62
- Krauth-Siegel RL, Coombs GH. Enzymes of parasite thiol metabolism as drug targets. Parasitol Today 1999;15:404–9
- Vieites M, Smircich P, Parajo-Costa B, et al. Platinum-based complexes of bioactive 3-(5-nitrofuryl)acroleine thiosemicarbazones showing anti-Trypanosoma cruzi activity. J Biol Inorg Chem 2008;13:733–5
- Silva JJN, Osakabe AL, Pavanelli WR, et al. In vitro and in vivo antiproliferative and trypanocidal activities of ruthenium NO donors. Brit J Pharmacol 2007;152:112–21
- Fricker SP, Mosi RM, Cameron B, et al. Metal compounds for the treatment of parasitic diseases. J Inorg Biochem 2008;102:1839–45
- Otero L, Vieites M, Boianí L, et al. Novel antitrypanosomal agents based on palladium nitrofurylthiosemicarbazone complexes: DNA and redox metabolism as potential therapeutic targets. J Med Chem 2006;49:3322–31
- Navarro M, Lehmann T, Cisneros-Fajardo EJ, et al. Toward a novel metal-based chemotherapy against tropical diseases. Part 5. Synthesis and characterization of new Ru(II) and Ru(III) clotrimazole and ketoconazole complexes and evaluation of their activity against Trypanosoma cruzi. Polyhedron 2000;19:2319–25
- Silva JJN, Pavanelli WR, da Silva ABF, et al. Complexation of the anti-Trypanosoma cruzi drug benznidazole improves solubility and efficacy. J Med Chem 2008;51:4104–14
- Donnici CL, Araújo MH, Oliveira HS, et al. Ruthenium complexes endowed with potent anti-Trypanosoma cruzi activity: synthesis, biological characterization and structure–activity relationships. Bioorg Med Chem 2009;15:5038–43
- Bernhardt PV, Sharpe PC, Islam M, et al. Thiosemicarbazones from the old to new: iron chelators that are more than just ribonucleotide reductase inhibitors. J Med Chem 2009;52:407–15
- Scozzafava A, Carta F, Supuran CT. Secondary and tertiary sulfonamides: a patent review (2008–2012). Expert Opin Ther Pat 2013;23:203--13
- Chohan ZH, Supuran CT. Structural elucidation and biological significance of 2-hydroxy-1-naphthaldehyde derived sulfonamides and their first row d-transition metal chelates. J Enzyme Inhib Med Chem 2008;23:240–51
- Supuran CT. Structure-based drug discovery of carbonic anhydrase inhibitors. J Enzyme Inhib Med Chem 2012;26:759–72
- Supuran CT. Carbonic anhydrases: novel therapeutic applications for inhibitors and activators. Nat Rev Drug Discov 2008;7:168–81
- De Simone G, Vitale RM, Di Fiore A, et al. Carbonic anhydrase inhibitors: hypoxia-activatable sulfonamides incorporating disulfide bonds that target the tumor-associated isoform IX. J Med Chem. 2006;49:5544–51
- Chohan ZH, Shad HA. Metal-based new sulfonamides: design, synthesis, antibacterial, antifungal, and cytotoxic properties. J Enzyme Inhib Med Chem 2012;27:403–12
- Chohan ZH, Shad HA, Supuran CT. Synthesis, characterization and biological studies of sulfonamide Schiff’s bases and some of their metal derivatives. J Enzyme Inhib Med Chem 2012;27:58–68
- Leite ACL, Moreira DRM, Cardoso MVO, et al. Synthesis, Cruzain docking, and in vitro studies of aryl-4-oxothiazolylhydrazones against Trypanosoma cruzi. ChemMedChem, 2007;2:1339–45
- Moreira DRM, Leite ACL, dos Santos RR, Soares MBP. Approaches for the development of new anti-Trypanosoma cruzi agents. Curr Drug Targets 2009;10:212–31
- Mahnken RE, Billadeau MA, Nikonowicz EP, Morrison H. Development of photo cis-platinum reagents. Reaction of cis-dichlorobis(1,10-phenanthroline) rhodium(III) with calf thymus DNA, nucleotides and nucleosides. J Am Chem Soc 1992;114:9253–65
- Frisch MJ, Trucks GW, Schlegel HB, et al. Gaussian 03, Revision B.04 ed. Wallingford, CT: Gaussian, Inc.; 2004
- Beck AD. Density-functional thermochemistry. 3. The role of exact exchanger. J Chem Phys 1983;98:5648–5652
- Lee CT, Yang WT, Parr RG. Development of the Colle-Salvetti correlation-energy formula into a functional of the electron density. Phys Rev B 1988;37:785–9
- Wadt WR, Hay PJ. Ab initio effective core potentials for molecular calculations. Potentials for the transition metal atoms Sc to Hg. J Chem Phys 1985;82:284–98
- Ehlers AW, Bohme M, Dapprich S, et al. A set of f-polarization functions for pseudo-potential basis sets of the transition metals ScCu, Yg and LaAu. Chem Phys Lett 1993;208:111–14
- Hollwarth A, Bohme M, Dapprich S, et al. A set of d-polarization functions for pseudo-potential basis sets of the main group elements AlBi and f-type polarization functions for Zn, Cd, Hg. Chem Phys Lett 1993;208:237–40
- Reed AE, Curtiss LA, Weinhold F. Intermolecular interactions from a natural bond orbital, donor-acceptor viewpoint. Chem Rev 1988;88:899–926
- Cioslowski J. A new population analysis based on atomic polar tensors. J Am Chem Soc 1989;111:8333–6
- Ugliengo P, Viterbo D, Chiari G. MOLDRAW: molecular graphics on a personal computer. Z Kristallogr 1993;207:9–23