Abstract
A novel proton transfer compound (HMeOABT) + (HDPC)− (1) and its Fe(III), Co(II), Ni(II) and Cu(II) complexes (2–5) have been prepared and characterized by spectroscopic techniques. Complex 4 has distorted octahedral conformation revealed by single crystal X-ray diffraction method. Structures of the other complexes might be proposed as octahedral according to experimental data. All compounds were also evaluated for their in vitro inhibition effects on hCA I and II for their hydratase and esterase activities. Although there is no inhibition for hydratase activities, all compounds have inhibited the esterase activities of hCA I and II. Data have been analyzed by using a one-way analysis of variance. The comparison of the inhibition studies of 1–5 to parent compounds indicates that 1–5 have superior inhibitory effects. The inhibition effects of 2–5 are also compared to inhibitory properties of the metal complexes of MeOABT and H2DPC, revealing an improved transfection profile.
Introduction
2,6-Pyridinedicarboxylic acid (or dipicolinic acid, H2DPC) is commonly used as an acid in proton transfer systemsCitation1. Continuing the path to synthesize water soluble proton transfer compounds, our group has focused on forming ion pairs between H2DPC and various organic bases, such as 2-aminobenzothiazoleCitation2, 2-amino-6-methylbenzothiazoleCitation3, 2-(piperazin-1-yl)ethanolCitation4, 2-amino-4-methylpyridineCitation5. The interest of metal complexes for H2DPC and its deprotonated forms, HDPC− and DPC2−, stems from their interesting structural features with various coordination modesCitation1, stabilization of unusual oxidation statesCitation6 and insulin-mimetic effectsCitation7,Citation8.
Benzothiazoles and their simple metal complexes are well known for their biological activitiesCitation2,Citation3,Citation9,Citation10. The simple transition metal complexes of 2-amino-6-methoxybenzothiazole (MeOABT) have been synthesizedCitation11, nevertheless there are few reports on the synthesis and structure characterization of mixed ligand metal complexes of MeOABT with other ligands, such as stearic acidCitation12,Citation13 and cyanonitrosylCitation14,Citation15. The mixed ligand metal complexes of these compounds have shown better biological activities than the simple onesCitation2–4. In order to prepare the mixed ligand complexes, two types of ligands, generally acids and bases, are brought together before coordination to the metal ionCitation1–5,Citation16,Citation17.
In this study, a novel proton transfer compound (HMeOABT)+(HDPC)− (1) between MeOABT and H2DPC, and its complexes, (HMeOABT)[Fe(DPC)2]·2H2O (2), (HMeOABT)2[Co(DPC)2]·4H2O (3), (HMeOABT)2[Ni(DPC)2]·H2O (4) and (HMeOABT)2[Cu(DPC)2]·H2O (5), have been prepared and characterized by elemental, spectral (1H-NMR, IR and UV-Vis), thermal analyses, magnetic measurements and molar conductivity. Single crystal X-ray analysis of the complex 4 was also reported.
Furthermore, we have investigated the potential use of these compounds as new inhibitors of human carbonic anhydrase (hCA I and hCA II) isozymes in the treatment of glaucoma, which is a group of diseases characterized by the gradual loss of visual field due to an elevation in intraocular pressure (IOP)Citation18,Citation19. Carbonic anhydrases (CAs, EC 4.2.1.1) are ubiquitous metalloenzymes to catalyze interconversion of carbon dioxide and water to bicarbonate and proton and are encoded by five unrelated gene families: α-, β-, γ-, δ- and ζ-CAs. There are sixteen α-CA isoforms or CA-related proteins identified in mammalsCitation20. Many of CA subtypes are targets for the design of pharmacological agents for the treatment of some disorders, such as glaucoma, obesity, cancer and osteoporosisCitation21. For glaucoma therapy, some clinical sulfonamide derivatives of CA inhibitors exist nowadays, but they have some side effects, such as fatigue, weight loss, paresthesias, leucopenia, kidney stones, and metabolic acidosisCitation22,Citation23. Therefore, the syntheses of novel non-sulfonamide and water soluble CA inhibitors are required to minimize the side effectsCitation2,Citation3,Citation16.
Experimental section
General methods and materials
All chemicals used were analytical reagents and were commercially purchased from Sigma-Aldrich (Munich, Germany). FeSO4·7H2O, Co(CH3COO)2·4H2O, Ni(CH3COO)2·4H2O, Cu(CH3COO)2·H2O, 2-amino-6-methoxybenzothiazole and pyridine-2,6-dicarboxylic acid were used as received. Elemental analyses for C, H, N and S were performed on Elementar Vario III EL (Hanau, Germany) and Fe, Co, Ni and Cu were detected with Perkin Elmer Optima 4300 DV ICP-OES (Perkin Elmer Inc., Wellesley, MA). 1H-NMR spectra were recorded using a Bruker DPX FT NMR (500 MHz) spectrometer (Karlsruhe, Germany) (SiMe4 as internal standard and 85% H3PO4 as an external standard). FT-IR spectra were recorded in the 4000–400 cm−1 region with Bruker Optics, Vertex 70 FT-IR spectrometer using ATR techniques (Ettlingen, Germany). Thermal analyses were performed on a SII Exstar 6000 TG/DTA 6300 model (Shimadzu Co, Kyoto, Japan) using platinum crucible with 10 mg sample. Measurements were taken in the static air, within 30 °C–900 °C temperature range. The UV-Vis spectra were obtained for aqueous solutions of the compounds (10−3 M) with a SHIMADZU UV-2550 spectrometer (Shimadzu Co, Kyoto, Japan) in the range of 200–900 nm. Magnetic susceptibility measurements at room temperature were performed using a Sherwood Scientific Magway MSB MK1 (Sherwood Scientific Ltd, Cambridge, UK) model magnetic balance by the Gouy method using Hg[Co(SCN)4] as calibrant. Molar conductances of the compound were determined in DMSO (10−3 M) at room temperature using a WTW Cond 315i/SET Model conductivity meter (Weilheim, Germany).
Synthesis of (HMeOABT)+(HDPC)− (1) and metal complexes (2–5)
A solution of MeOABT (0.900 g, 5 mmol) in 25 mL ethanol was added to the solution of H2DPC (0.836 g, 5 mmol) in 25 mL ethanol. The mixture was refluxed for three hours and then was cooled to room temperature. The reaction mixture was kept at room temperature for three hours to give white solid of 1 (1.649 g, 95% yield).
A solution of 1 mmol metal(II) salt [0.278 g FeSO4·7H2O or 0.249 g Co(CH3COO)2·4H2O or 0.248 g Ni(CH3COO)2·4H2O or 0.199 g Cu(CH3COO)2·H2O] in water (10 mL) was added dropwise to the solution of 1 (0.347 g, 1 mmol) in water/ethanol (1:1) (20 mL) with stirring at room temperature for two hours. The reaction mixture was kept at room temperature for two weeks to give green crystalline solid for 4 (0.538 g, 70% yield). Other amorphous solid metal complexes were obtained as orange for 2 (0.422 g, 70% yield), as brown for 3 (0.535 g, 65% yield) and as turquoise for 5 (0.503 g, 75% yield) (). The prismatic single crystals of 4 suitable for X-ray diffraction were separated from the batch and washed with EtOH/water (1:1). The elemental analysis of the synthesized compounds (1–5) can be seen in supplemental data (Table S1).
In addition, simple metal complexes of MeOABT (FeMeOABT, CoMeOABT, NiMeOABT and CuMeOABT) and of H2DPC (FeDPC, CoDPC, NiDPC and CuDPC) were synthesized according to the literature in order to compare the inhibition studies with the complex compounds of proton transfer saltCitation11,Citation24.
X-ray data collection and structure refinement
The crystal and instrumental parameters used in the unit-cell determination and data collection are summarized in for the compound 4. Crystallographic data of 4 was recorded on a Bruker Kappa APEX II CCD area-dedector X-ray diffractometer using graphite monochromatized with MoKα radiation (λ =0.71073 Å), using ω-2θ scan mode. The empirical absorption corrections were applied by multi-scan via Bruker, SADABS softwareCitation25. The structures were solved by the direct methods and subsequently completed by difference Fourier recycling. All non-hydrogen atoms were refined anisotropically using the full-matrix least-squares techniques on F2. The SHELXS-97 and SHELXL-97 programsCitation26 were used for all the calculations. The H atoms were placed in idealized positions and constrained to ride on their parent atoms with distances in the range N–H = 0.86 Å, C–H = 0.93–0.96 Å and with Uiso(H) =1.2Ueq(C,N). Hydrogen atoms of water molecules were located from difference Fourier maps and refined with isotropic thermal parameters, with distance of O–H = 0.78(4)–0.85(4) Å and with Uiso(H) = 1.2Ueq(O). The disorder of atoms C28a and C28b was refined, with occupancies of 0.485(6) and 0.515(6), respectively. The drawings of molecules were accomplished with the help of ORTEP-3 for WindowsCitation27.
Table 1. Crystal data and structure refinement details for compound 4.
Hydratase and esterase activity assay
The details of the purification of isozymes hCA-I and II from human erythrocytes have been explanied in the Supplementary dataCitation28–31.
On hydrationCitation32 of CO2, CO2-hydratase activity as an enzyme unit (EU) was calculated by using the equation ((t0−tc)/tc), where t0 and tc are the times for pH change of the nonenzymatic and the enzymatic reactions, respectively.
Carbonic anhydrase esterase activity was assayed by following the change in the absorbance at 348 nm of 4-nitrophenylacetate to 4-nitrophenylate ion over a period of 3 min at 25 °C using a spectrophotometer (SHIMADZU UV 1700 PharmaSpec) according to the method described in the literatureCitation33,Citation34. The enzymatic reaction, in a total volume of 3.0 mL, contained 1.4 mL of 0.05 M tris–SO4 buffer (pH 7.4), 1 mL of 3 mM 4-nitrophenylacetate, 0.5 mL H2O and 0.1 mL enzyme solution. A reference measurement was obtained by preparing the same cuvette without enzyme solution.
IC50 values (the concentration of inhibitor producing a 50% inhibition of CA activity) have been obtained as in vitro for free ligands (MeOABT and H2DPC) and their simple metal complexes, and the synthesized compounds 1–5, and acetazolamide (AAZ) as the control compound for their hydratase and esterase activities.
Statistical analysis
All the presented data were confirmed with three independent experiments and were expressed as the mean ± standard deviation (SD). Data were analyzed by using a one-way analysis of variance for multiple comparisons (SPSS 13.0). p value < 0.0001 was considered to be statistically significant.
Results and discussion
Crystal structure of 4 (HMeOABT)2[Ni(DPC)2]·H2O
The molecular structure of 4, with the atom labeling of symmetric unit is displayed as ORTEP representation in . The details of the crystal structure solution are summarized in and the selected bond lengths and angles are collected in .
Figure 2. An ORTEP drawing of asymmetric unit of 4 with the atom-numbering scheme. Displacement ellipsoids are drawn at the 40% probability level.
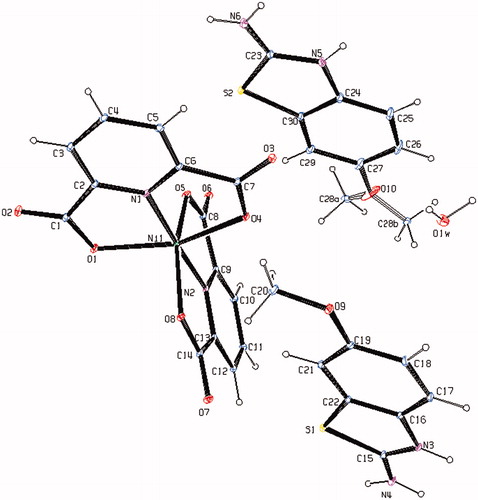
Table 2. Selected bond distances [Å] and angles [°] of compound 4.
The complex 4, (HMeOABT)2[Ni(DPC)2]·H2O, crystallizes in the triclinic P space group. The structure of 4 consists of two (HMeOABT)+ cations, one [Ni(DPC)2]2− anion and one uncoordinated water molecule. In complex 4, the Ni(II) ion coordinates to four oxygen atoms (O1, O4, and O5, O8) and two nitrogen atoms (N1 and N2) of two pyridine-2,6-dicarboxylate molecules which resulted with a distorted octahedral conformation. Both carboxylate oxygen atoms from DPC2− occupy the trans-apical positions of the Ni(II) ion, and define low trans-angle value around Ni(II) ion as 155.25(5)° for O1--Ni1--O4, which reveals a rather rigid structure of such tri-dentate ligands (). In the structure of 4, the N1--Ni1--N2 trans-angle is much closer to 180° [177.01(6)°] and the dihedral angle defined by the mean planes of two dipic ligands is 86.97(14)° showing that they fall almost perpendicular. The Ni--N (1.9671(17) and 1.9763(17) Å) and Ni–O (2.1210(17)--2.1698(17) Å) bond distances lie within expected range (). The methoxy group of one of the (HMeOABT)+ cations (C28) was disordered such that the methoxy group had occupancy of 0.485(6) at the site C28a and occupancy of 0.515(6) at the site C28b. In all essential details, the geometry of the complex regarding bond lengths and angles of the compound is in good agreement with the values observed in similar Ni(II) complexesCitation3,Citation35,Citation36.
1H NMR studies of (HMeOABT)+(HDPC)− (1)
The 1H-NMR spectrum of the compound 1 was obtained in d6-DMSO at room temperature using TMS as internal Standard (Figure S1). The 1H signals were assigned on the basis of chemical shifts, multiplicities, intensities of the signals and coupling constants. lists complete 1H-NMR assignments for the compounds 1.
Table 3. 1H-NMR chemical shifts (ppm) with coupling constants and assignments for compound 1.
H3 and H6 protons of the (HMeOABT)+ ring are doublet with 1H intensity and they are observed at 7.23 ppm (H3, 3JH3-H4 = 9.00 Hz) and 7.29 ppm (H6, 4JH6-H4 = 2.50 Hz). H4 is doublet-doublet with 1H intensity observed at 6.81 ppm (H4, 3JH4-H3 = 8.50, 4JH4-H6 = 2.50 Hz). In addition, H5 proton of the (HMeOABT)+ ring is singlet with 3H intensity and it is observed at 3.72 ppm. H8 proton of the (HDPC)− ring is triplet with 1H intensity and observed at 8.18 ppm (3JH8‐H7,9 = 7.00 Hz). H7 and H9 protons are symmetric and they are observed at 8.26 ppm as doublet with 2H intensity (3JH7,9-H8 = 7.50 Hz). The hydrogen atoms, on –NH2 (H1), ( =N+–H) (H2) and (=N+–H) (H10) in compound 1 were not observed in the 1H NMR spectrum.
The room temperature 1H-NMR spectrum for compound 1 indicates clearly the formation of the proton transfer compound with 1:1 ratio of MeOABT and H2DPC (Figure S1).
FT–IR measurements
The infrared spectral data of the starting compounds (MeOABT and H2DPC) and compounds 1–5 are given in Table S2. In the high frequency region, weak bands 3025–2836 cm−1 and 3094–3055 cm−1 are attributed to the stretching vibrations of methyl group and aromatic C–H, respectively. There is a broad absorption band at 2900 cm−1 attributed to the ν(OH) vibrations of carboxylate group of H2DPC. This band is not observed in compound 1 due to proton transfer. There are also broad absorption bands at 3463–3451 cm−1 attributed to the ν(OH) vibrations of uncoordinated water molecules in the complex compounds 2–5. The relatively weak and broad bands at 2777–2500 cm−1 are attributed to the ν(N+–H) vibration for 1–5. The absorption bands at 3383 and 3293 cm−1 of NH2 group of MeOABT are slightly shifted from those found for 1 (3392 and 3225 cm−1), for 2 (3290 and 3119 cm−1), for 3 (3363 and 3237 cm−1), for 4 (3360 and 3234 cm−1) and for 5 (3345 and 3272 cm−1) due to the weak intermolecular interactions. The carboxylate groups exhibit strong carbonyl bands in the region 1701–1456 cm−1. These bands are reflected by IR spectrum of the asymmetric (νas) and symmetric (νs) stretching vibrations at 1701 and 1456 cm−1 for H2DPC, 1649 and 1488 cm−1 for 1, 1664 and 1488 cm−1 for 2, 1658 and 1481 cm−1 for 3, 1636 and 1486 cm−1 for 4 and 1659 and 1481 cm−1 for 5. The differences (Δυ) between the asymmetric and symmetric stretches of the carboxylate groups of 2–5 are 176, 177, 150 and 178, respectively, which suggest a monodentate binding of the carboxylate group to the metal ion for all complexes. The C--O vibrations data for all compounds are between 1370 and 1048 cm−1 as expected. The strong absorption bands at the region of 1640–1415 cm−1 are attributed to the ν(C=N) and ν(C=C) vibrations for all compounds. The ring wagging vibrations of the pyridine groups are also observed at 799–673 cm−1 region for compounds H2DPC and 1–5. The weak bands at 458–425 cm−1 and 597–579 cm−1 are from the M–N and M–O vibrations of compound 2–5.
Thermal analyses of 1–5.
Figures S2–S6 show the TG-DTG and DTA curves of compounds 1–5, respectively, and thermal analyses results are given in Table S3.
For the compound 1, three stages are observed and the first endothermic stage corresponds to the loss of one mole HMeOABT. The second exothermic stage is consistent to the loss of two moles COO. The third exothermic one is the decomposition of the C5H4N which is the residue of HDPC.
For the compounds 2, 4 and 5, three stages are observed, and the first endothermic stage corresponds to the loss of the two, one and one moles of water, respectively. The second endothermic stage is consistent to the loss of one, two and two moles HMeOABT, respectively. Two moles of DPC are decomposed exothermically in the third stages for 2, 4 and 5. The final decomposition products are FeO, NiO and CuO, respectively, and they are identified by IR spectroscopy.
For the compound 3, three stages are observed, and the first endothermic peak corresponds to the loss of one mole of water. The second endothermic stage agrees to the loss of the C12H12N4S2 unit and the C18H12N2O10 unit is decomposed exothermically in the following stage. The final decomposition product is CoO identified by IR spectroscopy.
UV/Vis Spectrum, magnetic susceptibility and molar conductivity
The electronic spectra of compounds 1–5, and the free ligands MeOABT and H2DPC were recorded in water/ethanol (1:1) and in DMSO solution with 1 × 10−3 M concentrations at room temperature (Table S4, Figures S7, S8). Characteristic π–π* transitions are observed in the range of 267–298 nm (20 800–38 180 Lmol−1 cm−1) in water/ethanol and 255–297 nm (18 600–45 160 Lmol−1 cm−1) in DMSO for 1–5. The same π–π* transition profiles are also detected for the free ligands MeOABT (294 nm (34 020 Lmol−1 cm−1) in water/ethanol and 289 nm (34 550 Lmol−1 cm−1) in DMSO) and H2DPC (299 nm (10 000 Lmol−1 cm−1), nm in water/ethanol and 303 nm (4340 Lmol−1 cm−1), in DMSO). π–π* transitions of the free ligands do not show any marked differences from those of either proton transfer compound or metal complexes in both solutions. The intensities of the absorption bands for all compounds in DMSO are, in general, higher than in water/ethanol. The bands for the d-d transitions in water/ethanol and in DMSO are observed at 763 nm (240 Lmol−1 cm−1) and 784 nm (400 Lmol−1 cm−1) for 3, 782 nm (250 Lmol−1 cm−1) and 772 nm (700 Lmol−1 cm−1) for 4 and 781 nm (300 Lmol−1 cm−1) and 785 nm (610 Lmol−1 cm−1) for 5, respectively, which fit for octahedral structure for the complexesCitation2,Citation3 3, 4 and 5. The d-d transition for complex 2 containing Fe(III) ion with d5 has not been observed.
The room temperature magnetic moment of the metal complexes are 5.94 BM for 2, 3.86 BM for 3, 2.81 BM for 4 and 1.62 BM for 5 (per metal ion), indicating the presence of five (d5), three (d7), two (d8) and one (d9) unpaired electrons, respectively.
The molar conductivity data in water/ethanol and in DMSO are the 80.0 and 66.5 Ω−1 cm2 mol−1 for 2, 87.8 and 61.2 Ω−1 cm2 mol−1 for 3, 87.1 and 62.3 Ω−1 cm2 mol−1 for 4 and 93.1 and 58.4 Ω−1 cm2 mol−1 for 5 respectively, indicating that the complexes 3, 4 and 5 are ionic with a 2:1 ratio and complex 2 is ionic with 1:1 ratio.
The structure of the complexes 2, 3 and 5 were proposed by means of the ratio of MeOABT:DPC:M ion:water molecules (M = Fe(III), Co(II) and Cu(II)) obtained from the elemental and thermal analyses, and of the spectral studies of UV and FT-IR as well as the studies of magnetic susceptibility and molar conductivity (). All experimental results are also in good agreement with the X-ray diffraction studies for complex 4 ().
In vitro inhibition studies
In this study, human carbonic anhydrase isozymes (hCA I and hCA II) were purified from erythrocyte cells using Sepharose 4B-L-Tyrosine-p-aminobenzenesulfonamide affinity chromatography method. Then the inhibition potentials of the synthesized compounds (1–5), MeOABT, H2DPC and metal complexes of MeOABT and H2DPC, as well as standard, clinically used CAI (AAZ) have been tested for two cytosolic isoforms hCA I and hCA II. The inhibition assays were performed by hydratase and esterase activity method under in vitro conditions. The following structure and activity relationships can be observed in the .
Table 4. IC50 values of newly synthesized compounds on the esterase activities of hCA I and hCA II isozymes.
According to in vitro studies, all of the synthesized compounds have not inhibited the hydratase activity of hCA I and hCA II isoforms. Also, no inhibition effect of H2DPC and its metal complexes have been observed on esterase activities of hCA I and II. Other studied compounds act as moderate inhibitors (compared to AAZ) against to esterase activities of hCA I and hCA II isozymes, with IC50 values in the range of 0.174 ± 0.004–0.800 ± 0.003 mM. All compounds, except H2DPC and its metal complexes have more powerful inhibition effect for hCA II than hCA I, but the differences of inhibition potentials are little for these isozymes. MeOABT has weaker inhibition potential than all other compounds. Overall differences between the lowest and the highest inhibition potentials are nearly 5-fold (i.e. IC50 value of simple ligant, MeOABT, for hCA I = 0.800 ± 0.003 mM, IC50 value of 2, for hCA II = 0.174 ± 0.004 mM). Among the simple metal complexes of MeOABT, NiMeOABT is the most effective inhibitor on hCA I and hCA II, with IC50 values 0.305 ± 0.001 and 0.238 ± 0.009 mM, respectively.
While H2DPC does not show any inhibition effects on hCA I and hCA II, the proton transfer salt (1) has inhibition effect. In addition, the salt has more effective inhibition effect than MeOABT (IC50 value of 1 for hCA I = 0.512 ± 0.004 mM, for hCA II = 0.493 ± 0.002 mM, IC50 value of MeOABT for hCA I = 0.800 ± 0.003 mM, for hCA II = 0.754 ± 0.003 mM). Similarly, the metal complexes of 1 (0.193 ± 0.002 – 0.242 ±0.006 mM for hCA I and 0.174 ± 0.004–0.214 ± 0.002 mM for hCA II) have more potent effects than 1 (0.512 ± 0.004 mM for hCA I and 0.493 ± 0.002 mM for hCA II) and than all simple metal complexes of MeOABT (0.305 ± 0.001–0.356 ± 0.008 mM for hCA I and 0.238 ± 0.009–0.330 ± 0.007 mM for hCA II). These results reveal that the structural changes increase the inhibition effects. Hence, we conclude that metal ions facilitate the enzyme-inhibitor interactions.
All inhibition values found in this study are in good agreement with those found in our similar previous studiesCitation2,Citation3.
Conclusions
In the present work, Fe(III), Co(II), Ni(II) and Cu(II) ionic complexes with (HMeOABT)+(HDPC)− (1) were prepared for the first time. In complex 4, Ni(II) ion coordinates to four oxygen atoms and two nitrogen atoms of two pyridine-2,6-dicarboxylate molecules forming a distorted octahedral conformation. Elemental analyses and all measurements show good agreement with the structures. The structures of 2, 3 and 5 might be proposed as octahedral according to results of elemental, spectral, magnetic measurement, molar conductivity and thermal analyses.
The novel compounds (1–5) possess significant inhibition effect on hCA I and on hCA II for esterase activity. These results suggest that further inhibition studies are worthwhile in order to obtain correlation in such compounds and the derivatives of such compounds should be subject to further inhibition in vivo tests. The order of the inhibition effects increasing through starting compounds to proton transfer salt and to the complexes of these compounds might be due to the structural changes leading to an impressive difference of activity.
Declaration of interest
The authors acknowledge the support provided by Dumlupınar University Research Fund (grant No. 2012/16). The authors report no conflicts of interest. The authors alone are responsible for the content and writing of this article.
Supplementary material available online Supplementary Tables S1–S4 Supplementary Figures S1–S8.
Supplementary Material
Download PDF (418.6 KB)Acknowledgements
In addition, the authors would like to thank the Medicinal Plants and Medicine Research Center of Anadolu University, for allowing us to use the X-ray facility.
References
- Aghabozorg H, Manteghi F, Sheshmani S. A brief review on structural concepts of novel supramolecular proton transfer compounds and their metal complexes. J Iran Chem Soc 2008;5:184–227
- İlkimen H, Yenikaya C, Sarı M, et al. Synthesis and characterization of a proton transfer salt between 2,6-pyridinedicarboxylic acid and 2-aminobenzothiazole, and its complexes and their inhibition studies on carbonic anhydrase isoenzymes. J Inhib Enzyme Med Chem 2013. [Epub ahead of print]. doi: 10.3109/14756366.2013.782299
- İlkimen H, Yenikaya C, Sarı M, et al. Synthesis and characterization of a proton transfer salt between dipicolinic acid and 2-Amino-6-methylbenzothiazole and its complexes, and their inhibition studies on carbonic anhydrase isoenzymes. Polyhedron 2013;61:56–64
- Büyükkıdan N, Yenikaya C, İlkimen H, et al. Synthesis, characterization and antimicrobial activity of a novel proton salt and its Cu(II) complex. Russian J Coord Chem 2013;39:24–31
- Büyükkidan N, Yenikaya C, Sari M, et al. Synthesis, characterization, and biological evaluation of Cu(II) complexes with the proton transfer salt of 2,6-pyridinedicarboxylic acid and 2-amino-4-methylpyridine. J Coord Chem 2011;64:3353–65
- Hoof DL, Tisley DG, Walton RA. Studies on metal carboxylates. Part III. Pyridine-2,6-dicarboxylates of the lanthanides. Synthesis and spectral studies and the X-ray photoelectron spectra of several pyridine carboxylate complexes. J Chem Soc Dalton Trans 1973;2:200–4
- Yang L, Crans DC, Miller SM, et al. Cobalt(II) and cobalt(III) dipicolinate complexes: solid state, solution, and in vivo ınsulin-like properties. Inorg Chem 2002;41:4859–71
- Buglyó P, Crans DC, Nagy EM, et al. Aqueous chemistry of the VanadiumIII (VIII) and the VIII−dipicolinate systems and a comparison of the effect of three oxidation states of vanadium compounds on diabetic hyperglycemia in rats. Inorg Chem. 2005;44:5416–27
- Prakash A, Adhikari D. Application of Schiff bases and their metal complexes -- a review. Int J ChemTech Res 2011;3:1891–6
- Genin MJ, Biles C, Keiser BJ, et al. Novel 1,5-diphenylpyrazole nonnucleoside HIV-1 reverse transcriptase ınhibitors with enhanced activity versus the delavirdine-resistant P236L mutant: lead identification and SAR of 3- and 4-substituted derivatives. J Med Chem 2000;43:1034–40
- Sinha AIP, Agarwal P, Tewari SP. Metal complexes of 6-methoxy-2-benzothiazolamine. Curr Sci 1989;58:899–903
- Mathur N, Heda LC, Mathur VK, Saxena P. Study of CLSI-M44-A disk diffusion method for determining the susceptibility of candida species against novel complexes derived from copper stearate with 2-amino benzothiazoles. Tenside Surfactants Detergents 2011;48:23–7
- Mathur N, Ojha KG, Imran A, Pooja S. Viscometric behaviour and micellization of complexes of copper (II) stearate with N-donor heterocyclic dyes. Tenside Surfactants Detergents 2009;46:24–30
- Maurya RC, Mishra DD, Khan IB, Awasthi S. Magneto, spectral and thermal studies of some mixed-ligand cyanonitrosyl chromium (CrNO)5 complexes involving benzothiazole derivatives. J Inst Chem (India) 1992;64:7–8
- Maurya RC, Mishra DD, Awasthi S, Pandey M. Studies on some chromium(I) cyanonitrosyl heterocomplexes. Nat Acad Sci Lett (India) 1991;13:91–3
- Yenikaya C, Sarı M, İlkimen H, et al. Synthesis, structural and antiglaucoma activity studies of a novel amino salicylato salt and its Cu(II) complex. Polyhedron 2011;30:535–41
- Yenikaya C, Sarı M, Bülbül M, et al. Synthesis, characterization and antiglaucoma activity of a novel proton transfer compound and a mixed-ligand Zn(II) complex. Bioorganic Med Chem 2010;18:930–8
- Schuman JS. Antiglaucoma medications: a review of safety and tolerability issues related to their use. Clin Ther 2000;22:167–208
- Scozzafava A, Banciu MD, Popescu A, Supuran CT. Carbonic anhydrase inhibitors: inhibition of isozymes I, II and IV by sulfamide and sulfamic acid derivatives. J Enzyme Inhib Med Chem 2000;15:443–553
- Supuran CT. Carbonic anhydrases: novel therapeutic applications for inhibitors and activators. Nat Rev Drug Discov 2008;7:168–81
- Tuccinardi T, Bertini S, Granchi C, et al. Salicylaldoxime derivatives as new leads for the development of carbonic anhydrase inhibitors. Bioorg Med Chem 2013;21:1511–15
- Steele RM, Benedini F, Biondi FS, et al. Nitric oxide-donating carbonic anhydrase inhibitors for the treatment of open-angle glaucoma. Bioorg Med Chem Lett 2009;19:6565–70
- Vernier W, Chong W, Rewolinski D, et al. Thioether benzenesulfonamide inhibitors of carbonic anhydrases II and IV: structure-based drug design, synthesis, and biological evaluation. Bioorg Med Chem 2010;18:3307–19
- Anderegg G, Bottari E. Pyridine derivatives as ligands. VII. Complexing tendency of substituted dipicolinate ions. Helv Chim Acta 1965;48:887–92
- Bruker. SADABS. Madison: Bruker AXS Inc.; 2005
- Sheldrick GM. SHELXS97 AND SHEXL97. Program for crystal structure solution and refinement. Germany: University of Göttingen; 1997
- Farrugia LJ. ORTEP-3 for Windows – a version of ORTEP-III with a graphical user interface (GUI). J Appl Cryst 1997;30:565
- Arslan O, Nalbantoğlu B, Demir N, et al. A new method for the purification of carbonic anhydrase isozymes by affinity chromatography. Trop J Med Sci 1996;26:163–6
- Rickli EE, Ghazanfar SA, Gibbson BH, Edsall JT. Carbonic anhydrases from human erythrocytes: preparation and properties of two enzymes. J Biol Chem 1964;239:1065–8
- Bradford MM. A rapid and sensitive method for the quantitation of microgram quantities of protein utilizing the principle of protein-dye binding. Anal Biochem 1976;72:248–54
- Laemmli UK. Cleavage of structural proteins during the assembly of the head of bacteriophage T4. Nature 1970;227:680–5
- Wilbur KM, Anderson NG. Electrometric and colorimetric determination of carbonic anhydrase. J Biol Chem 1948;176:147–54
- Verpoorte JA, Mehta S, Edsall JT. Esterase activities of human carbonic anhydrases B and C. J Biol Chem 1967;242:4221–9
- Innocenti A, Scozzafava A, Parkkila S, et al. Investigations of the esterase, phosphatase and sulfatase activities of the cytosolic mammalian carbonic anhydrase isoforms I, II and XIII with 4-nitrophenyl esters as substrates. Bioorg Med Chem Lett 2008;18:2267–71
- Eshtiagh-Hosseini H, Aghabozorg H, Mirzaei M, et al. Diversity in coordination behavior of dipicolinic acid with lead(II), calcium(II), and nickel(II) in the presence of pyrazine and 2-amino-4-methylpyridine spacers in construction of three supramolecular architectures. J Mol Struct 2010;973:180–9
- Borah MJ, Singh RKB, Sinha UB, et al. Synthesis and crystal structure determination of dimeric Co(II) and Ni(II) with pyridine-2,6-dicarboxylic acid. J Chem Cryst 2012;42:67–75