Abstract
A series of 20 N-substituted derivatives of 1-arylimidazolidyn-2-ylideneurea and products of their cyclization was designed as compounds having double antinociceptive and serotoninergic activity. Ethyl {[(1-arylimidazolidin-2-ylidene)carbamoyl]amino}acetates were prepared from 1-aryl-4,5-dihydro-1H-imidazol-2-amines and ethyl isocyanatoacetate, and then converted with ammonia solution to 2-{[(1-phenylimidazolidin-2-ylidene)carbamoyl]amino}acetamides. Both series of N-substituted derivatives of 1-arylimidazolidyn-2-ylideneureas were subjected to cyclization to respective imidazo[1,2-a][1,3,5]triazines. Chain and cyclic compounds bearing ester moiety affected spontaneous locomotor activity, body temperature of mice as well as showed antinociceptive and serotoninergic activity. Interestingly, their antinociceptive activity was not reversed by naloxone, thus it is not mediated through the opioid system. Chain and cyclic compounds bearing amide moiety were devoid of central nervous system (CNS) activity which may be attributed to unfavorably low lipophilicity (connected with too high polar surface area and too small molecular volume) and poor blood–brain barrier permeation properties.
Introduction
Pain can significantly affect patients’ health and can have serious medical negative consequences, including poor nutrition, decreased appetite, abnormal sleep patterns, fatigue, and impairment of daily living activitiesCitation1. Furthermore, pain can cause psychological impairment and decrease healing and recovery from injuries and illnessCitation1. Pain is also connected with social and economic consequences due to the influence on the functioning of the individual in the family and society as well as due to high costs of pain treatment. Efficient treatment of pain requires more effective medications devoid of side effects of current drugs. For morphine and related antinociceptive compounds the most severe side effects are euphoria/dysphoria, sedation, depression of respiratory center, depression of vasomotor center with hypotension, nausea, convulsions, tolerance and serious dependenceCitation2. There are two main approaches which are exploited in searching for new antinociceptive drugs: elaboration of more selective and safer substances acting through the well-established molecular targets and identification of new molecular targets thanks to progress in proteomics and bioinformatics.
Opioid receptors are among the most important molecular targets for antinociceptive medications. Many morphine-like opioid analgesics have in their structure similar features, i.e. the phenyl ring, tertiary nitrogen atom and the two carbon fragment (e.g. as a part of the piperidine ring), which are required by the receptor cavityCitation2–4. These shared structural features can be found in bezitramide, fentanyl and petidine, and their analogues, Citation2. This “pharmacophore” model was proposed by Beckett (with its subsequent modificationsCitation5–7) and was one of the first models used to explain the antinociceptive activity of morphine derivativesCitation2. Discovery of pharmacological properties of salvinorin A wich is κ opioid receptor agonist lacking a protonable nitrogen atom capable to interact with the conserved Asp(3.32) led to revisiting of the classical opioid receptor pharmacophore model. Consequently, non-classical pharmacophore models explaining opioid receptor activity were proposed as presented in . These models involve a base (B), a hydrophobic (H) and aromatic moiety (Ar) or hydrogen bond acceptor (HA), hydrophobic (H), and aromatic groups (Ar)Citation5,Citation8–11.
Figure 1. Structural formulas of bezitramide, fentanyl and petidine. Pharmacophoric features according to the Beckett’s model are shown in boldCitation2,Citation12.
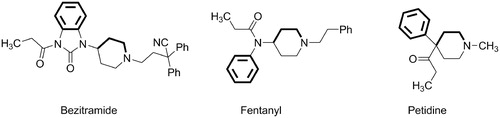
Figure 2. Non-classical opioid receptor pharmacophore models. B, base; Ar, aromatic; H, hydrophobic region; HA, hydrogen bond acceptorCitation2,Citation12.
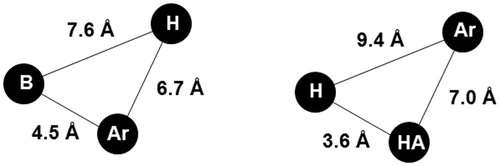
Following the non-classical pharmacophore models for opioid receptor activity, we have previously reported several series of compounds with antinociceptive activity mediated through the opioid system (series A–ECitation8–11), partially mediated through opioid system (series FCitation2) or with a different mechanism of antinociceptive activity (series GCitation12), . Some of these compounds also exerted serotoninergic activity according to the pharmacophore model presented in .
Figure 3. Previously reported antinociceptive and serotoninergic compounds. Series A–E and partially F exert their antinociceptive activity through the opioid system. Series G and some of compounds from series F have antinociceptive activity of unknown mechanismCitation2,Citation8–12.
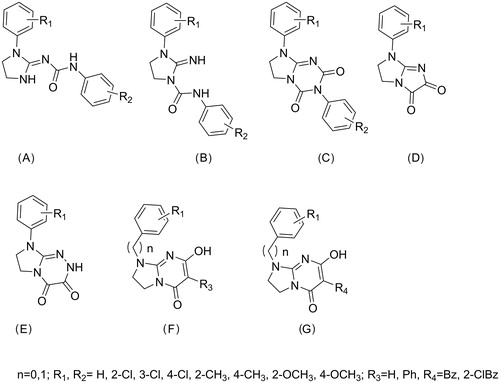
Figure 4. Pharmacophore model for the 5HT2 receptorCitation2,Citation10,Citation12.
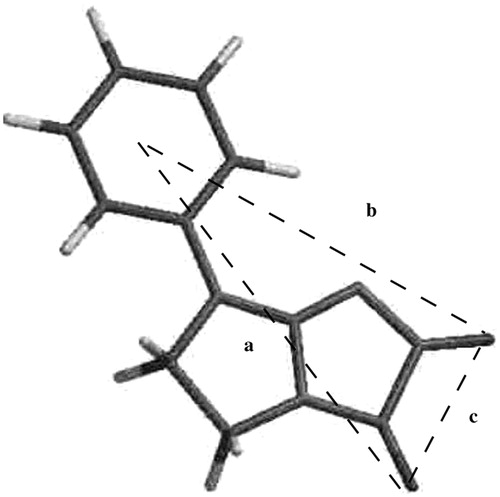
Interestingly, previous investigations have implicated that there is an interaction between opiatergic and serotoninergic pathways, and for example the involvement of opioid system in the modulation of serotoninergic function may contribute to the pharmacological profile of tramadolCitation13,Citation14. Reversely – nociceptive transmission terminated in the supraspinal structures is modulated with not only opioid but also noradrenaline and serotonin systems exerting some modulatory influencesCitation15. It has been also proposed that additional δ opioid receptor activity is favorable for compounds with anti-depressive properties associated mainly with the modulation of the serotoninergic systemCitation16.
In our continuous effort towards novel antinociceptive compounds with additional serotoninergic activity, we have designed and synthesized a series of 20 N-substituted derivatives of 1-arylimidazolidyn-2-ylideneurea (3a–3e, 4a–4e) and products of their cyclization (5a–5e, 6a–6e)Citation17–20 (Scheme 1). The rationale of this work can be summarized as follows: (1) the designed compounds follow the non-classical pharmacophore model for opioid receptor activity as well as the pharmacophore model for the serotoninergic activity; (2) some of the previously obtained series were characterized with high lipohilicity values, thus we decided to introduce ester and amide moieties to lower lipohilicity values; (3) the set of substituents in the aryl ring was selected on the basis of our earlier experience with the substituent effect on the activity; (4) the imidazo[1,2-a][1,3,5]triazine system is present in many pharmalogically active compounds, including compounds with antioxidant, anti-cancer and cytotoxicCitation21,Citation22 and anti-microbial activityCitation23.
Here we present synthesis, drug-likeness evaluation, ADMET (absorption, distribution, metabolism, excretion, toxicity) prescreening, pharmacological studies for central nervous system (CNS) activity and structure–activity relationship analysis for 20 N-substituted derivatives of 1-arylimidazolidyn-2-ylideneurea and products of their cyclization. It was found that compounds (4a–4e) and (6a–6e) bearing amide moiety are devoid of central nervous activity, most probably due to unfavorable ADMET profile (too low lipophilicity values connected with too high polar surface area and too small molecular volume and poor blood–brain barrier permeation properties). Compounds (3a–3e) and (5a–5e) bearing ester moiety displayed expected antinociceptive and serotoninergic activity, but – surprisingly – their antinociceptive activity was not reversed by naloxone, thus it is not mediated through the opioid system.
Methods
Chemistry
Reactions were routinely monitored by thin-layer chromatography (TLC) in silica gel (60 F254 Merck plates), and the products were visualized with ultraviolet light of 254-nm wavelength. All NMR spectra were acquired on Bruker Fourier 300 MHz spectrometer. Spectra were recorded at 25 °C using DMSO as a solvent with a non-spinning sample in 5 mm NMR-tubes. MS spectra were recorded on Bruker microTOF-Q II and processed using Compass Data Analysis software. The elementary analysis was performed with the application of Perkin–Elmer analyzer. Melting points were determined with Boetius apparatus.
General procedure to obtain compounds (3a–3e)
Ethyl isocyanatoacetate 2.58 g (0.02 mol) was dissolved in 25 ml of dichloromethane under the atmosphere of dry nitrogen and added to the solution of free base (0.02 mol) of 1-aryl-4,5-dihydro-1H-imidazol-2-amines (1a–1e) dissolved in 100 ml of dichloromethane. The mixture was shaken for 24 h at room temperature. Solvent was removed by distillation and the rubber-like residue was treated with warm propan-2-ol. The solid product ethyl {[(1-arylimidazolidin-2-ylidene)carbamoyl]amino}acetate (3a–3e) was filtrated off and recrystallized from propan-2-ol.
Ethyl {[(1-phenylimidazolidin-2-ylidene)carbamoyl]amino}acetate (3a)
Ethyl isocyanatoacetate 2.58 g (0.02 mol) was dissolved in 25 ml of dichloromethane under the atmosphere of dry nitrogen and added to the solution of free base 3.22 g (0.02 mol) of 1-phenyl-4,5-dihydro-1H-imidazol-2-amine (1a) dissolved in 100 ml of dichloromethane. The mixture was shaken for 24 h at room temperature. Solvent was removed by distillation and the rubber-like residue was treated with warm propan-2-ol. 2.32 g of (3a) was obtained (40% yield), white crystalline solid, m.p. 104–106 °C; 1H NMR (DMSO-d6, 300 MHz): δ = 8.16 (s, 1H, NH); 7.09–7.80 (m, 5H, H-Ar); 7.31 (s, 1H, NH); 4.05 (dd, 2H, CH2, J = 9.0, J′ = 7.6 Hz); 4.19 (dd, 2H, CH2, J = 9.0, J′ = 7.6 Hz); 3.98–4.02 (m, 2H, CH2); 3.71–3.85 (s, 2H, CH2), 1.26–1.31 (t, 3H, CH3, J = 7.1 Hz); 13C NMR (DMSO-d6, 75 MHz): δ = 14.6 (CH3), 41.2 (CH2), 42.7 (CH2), 42.3 (CH2), 45.8 (CH2), 120.1, 120.5, 121.7, 122.9, 123.2, 125.6 (C-Ar), 164.6 (C-2), 170.7 (C = O), 171.5 (C = O); EIMS m/z 291.1 [M + H]+. HREIMS (m/z): 290.1330 [M+] (Calcd for C14H18N4O3 290.3300); Anal Calcd for: C14H18N4O3 C, 57.92; H, 6.2; N, 19.30. Found C, 57.99; H, 6.36; N, 19.39.
Ethyl {[1-(3-chloropheny)limidazolidin-2-ylidene)carbamoyl]amino}acetate (3b)
Ethyl isocyanatoacetate 2.58 g (0.02 mol) was dissolved in 25 ml of dichloromethane under the atmosphere of dry nitrogen and added to the solution of free base 3.90 g (0.02 mol) of 1-(3-chlorphenyl)-4,5-dihydro-1H-imidazol-2-amine (1b) dissolved in 100 ml of dichloromethane. The mixture was shaken for 24 h at room temperature. Solvent was removed by distillation and the rubber-like residue was treated with warm propan-2-ol. 4.35 g of (3b) was obtained (67% yield), white crystalline solid, m.p. 94–95 °C; 1H NMR (DMSO-d6, 300 MHz): δ = 8.56 (s, 1H, NH); 8.05 (s, 1H, NH); 7.05–7.80 (m, 4H, H-Ar); 4.00 (dd, 2H, CH2, J = 8.9, J′ = 7.5 Hz); 4.10 (dd, 2H, CH2, J = 8.9, J′ = 7.5 Hz); 3.60 (m, 2H, CH2); 3.20 (s, 2H, CH2), 1.19–1.23 (t, 3H, CH3, J = 7.0 Hz); 13C NMR (DMSO-d6, 75 MHz): δ = 14.5 (CH3), 40.1 (CH2), 42.3 (CH2), 43.9 (CH2), 45.6 (CH2), 117.9, 118.2, 119.6, 119.7, 122.4, 122.4 (C-Ar), 164.8 (C-2), 171.6 (C = O), 171.1 (C = O); EIMS m/z 325.2 [M + H]+. HREIMS (m/z): 351.0986 [M+] (Calcd for C14H17ClN4O3 324.7790); Anal Calcd for C14H17ClN4O3 C, 51.77; H, 5.28; Cl, 10.92; N, 17.25. Found C, 51.71; H, 5.26; Cl, 10.81, N, 17.15.
Ethyl {[1-(4-chloropheny)limidazolidin-2-ylidene)carbamoyl]amino}acetate (3c)
Ethyl isocyanatoacetate 2.58 g (0.02 mol) was dissolved in 25 ml of dichloromethane under the atmosphere of dry nitrogen and added to the solution of free base 3.90 g (0.02 mol) of 1-(4-chlorphenyl)-4,5-dihydro-1H-imidazol-2-amine (1c) dissolved in 100 ml of dichloromethane. The mixture was shaken for 24 h at room temperature. Solvent was removed by distillation and the rubber-like residue was treated with warm propan-2-ol. 3.64 g of (3c) was obtained (56% yield), white crystalline solid, m.p. 125–127 °C; 1H NMR (DMSO-d6, 300 MHz): δ = 8.64 (s, 1H, NH); 7.35–7.86 (m, 4H, H-Ar); 7.10 (s, 1H, NH); 4.02 (dd, 2H CH2, J = 8.9, J′ = 7.4 Hz); −4.15 (dd, 2H, CH2, J = 8.9, J′ = 7.4 Hz); 4.20–4.22 (m 2H, CH2); 3.55–3.74 (2H, CH2), 1.12–1.34 (t, 3H, CH3, J = 7.1 Hz); 13C NMR (DMSO-d6, 75 MHz): δ = 15.1 (CH3), 40.2 (CH2), 41.0 (CH2), 42.3 (CH2), 44.1 (CH2), 121.1, 121.7, 124.9, 126.6, 128.4, 128.5 (C-Ar), 164.7 (C-2), 170.7 (C = O), 171.4 (C = O); EIMS m/z 325.3 [M + H]+. HREIMS (m/z): 324.0995 [M+] (Calcd for C14H17ClN4O3 324.7790); Anal Calcd for C14H17ClN4O3 C, 51.77; H, 5.28; Cl, 10.92, N, 17.25. Found C, 51.85; H, 5.25; Cl, 10.98, N, 17.20.
Ethyl {[1-(2,6-dichloropheny)limidazolidin-2-ylidene)carbamoyl]amino}acetate (3d)
Ethyl isocyanatoacetate 2.58 g (0.02 mol) was dissolved in 25 ml of dichloromethane under the atmosphere of dry nitrogen and added to the solution of free base 4.58 g (0.02 mol) of 1-(2,6-dichlorophenyl)-4,5-dihydro-1H-imidazol-2-amine (1d) dissolved in 100 ml of dichloromethane. The mixture was shaken for 24 h at room temperature. Solvent was removed by distillation and the rubber-like residue was treated with warm propan-2-ol. 3.73 g of (3d) was obtained (52% yield), white crystalline solid, m.p. 146–148 °C; 1H NMR (DMSO-d6, 300 MHz): δ = 8.94 (s, 1H, NH); 6.89–7.81 (m, 5H, H-Ar); 7.03 (s, 1H, NH); 4.15 (dd, 2H, CH2 J = 8.9, J′ = 7.5 Hz); 4.29 (dd, 2H, CH2, J = 8.9, J′ = 7.5 Hz); 4.20–4.26 (m 2H, CH2); 3.78-3.81 (2H, CH2), 1.20–1.31 (t, 3H, CH3, J = 7.0 Hz); 13C NMR (DMSO-d6, 75 MHz): δ = 15.4 (CH3), 40.5 (CH2), 41.6 (CH2), 42.3 (CH2), 45.8 (CH2), 120.1, 120.5, 121.2, 122.9, 123.2, 125.6 (C-Ar), 164.4 (C-2), 170.7 (C = O), 171.5 (C = O); EIMS m/z 360.2 [M + H]+. HREIMS (m/z): 359.0796 [M+] (Calcd for C14H16Cl2N4O3 359.2280); Anal Calcd for: C14H16Cl2N4O3 C, 46.81; H, 4.49; Cl, 19.74; N, 15.60. Found C, 46.85; H, 4.42; Cl, 19.81; N, 15.71.
Ethyl {[1-(4-methoxypheny)limidazolidin-2-ylidene)carbamoyl]amino}acetate (3e)
Ethyl isocyanatoacetate 2.58 g (0.02 mol) was dissolved in 25 ml of dichloromethane under the atmosphere of dry nitrogen and added to the solution of free base 3.82 g (0.02 mol) of 1-(4-methoxyphenyl)-4,5-dihydro-1H-imidazol-2-amine (1 e) dissolved in 100 ml of dichloromethane. The mixture was shaken for 24 h at room temperature. Solvent was removed by distillation and the rubber-like residue was treated with warm propan-2-ol. 3.97 g of (3e) was obtained (62% yield), white crystalline solid, m.p. 123–125 °C; 1H NMR (DMSO-d6, 300 MHz): δ = 8.16 (s, 1H, NH); 7.09–7.80 (m, 4H, H-Ar); 7.03 (s, 1H, NH); 4.00 (dd, 2H, CH2, J = 9.0, J′ = 7.5 Hz); 4.22 (dd, 2H, CH2, J = 9.0, J′ = 7.5 Hz); 4.20–4.26 (m 2H, CH2); 3.65–3.89 (m, 2H, CH2), 3.40–3.45 (s, 3H, OCH3); 1.25–1.34 (t, 3H, CH3, J = 7.0 Hz); 13C NMR (DMSO-d6, 75 MHz): δ = 14.9 (CH3), 22.4 (OCH3), 40.7 (CH2), 41.2 (CH2), 42.3 (CH2), 45.8 (CH2), 119.1, 119.5, 120.2, 121.9, 123.2, 123.6 (C-Ar), 164.9 (C-2), 169.7 (C = O), 173.1 (C = O); EIMS m/z 321.1 [M + H]+. HREIMS (m/z): 320.1579 [M+] (Calcd for C15H20N4O4 320.3570); Anal Calcd for: C15H20N4O4 C, 56.24; H, 6.29; N, 17.49. Found C, 56.27; H, 6.21; N, 17.43.
General procedure to obtain compounds (4 a–4e)
Ethyl {[(1-arylimidazolidin-2-ylidene)carbamoyl]amino}acetates (3a–3e) (0.01 mol) were dissolved in 50 ml of methanol and added to 20% aqueous solution of ammonia. The mixture was shaken for 6 h at room temperature. The solid product was filtrated off and recrystallized from propan-2-ol.
2- {[(1-Phenylimidazolidin-2-ylidene)carbamoyl]amino}acetamide (4a)
Ethyl {[(1-phenylimidazolidin-2-ylidene)carbamoyl]amino}acetate (3a) (0.01 mol) was dissolved in 50 ml of methanol and added to 20% aqueous solution of ammonia. The mixture was shaken for 6 h at room temperature. The solid product was filtrated off and recrystallized from propan-2-ol. 1.7 g of (4a) was obtained (65% yield), white crystalline solid, m.p. 161–163 °C; 1H NMR (DMSO-d6, 300 MHz): δ = 9.62 (s, 1H, NH); 8.46 (s, 1H, NH); 8.11–8.15 (d, 2H, NH2); 7.40–7.68 (m, 5H, H-Ar); 4.02 (dd, 2H, CH2, J = 8.9, J′ = 7.4 Hz); 4.18 (dd, 2H, CH2, J = 8.9, J′ = 7.4 Hz); 3.66–3.80 (m 2H, CH2); 13C NMR (75 MHz, DMSO-d6): δ = 40.4 (CH2), 43.8 (CH2), 46.2 (CH2), 119.04, 120.1, 121.2, 122.1, 122.8, 123.4 (C-Ar), 164.1 (C-2), 171.7 (C = O), 172.7 (C = O); EIMS m/z 262.3 [M + H]+. HREIMS (m/z): 261.0844 [M+] (Calcd for C12H15N5O2 261.2920); Anal Calcd for: C12H15N5O2 C, 55.16; H, 5.79; N, 26.81. Found C, 55.26; H, 5.67; N, 26.95.
2-{[(1-(3-Chlorophenyl)imidazolidin-2-ylidene)carbamoyl]amino}acetamide (4b)
Ethyl {[1-(3-chlorophenylimidazolidin-2-ylidene)carbamoyl]amino}acetate (3b) (0.01 mol) was dissolved in 50 ml of methanol and added to 20% aqueous solution of ammonia. The mixture was shaken for 6 h at room temperature. The solid product was filtrated off and recrystallized from propan-2-ol. 1.21 g of (4b) was obtained (41% yield), white crystalline solid, m.p. 183–184 °C; 1H NMR (DMSO-d6, 300 MHz): δ = 10.01 (s, 1H, NH); 8.71 (s, 1H, NH); 7.89–7.95 (d, 2H, NH2); 7.30–7.68 (m, 4H, H-Ar); 4.07 (dd, 2H, CH2, J = 8.9, J′ = 7.6 Hz); 4.21 (dd, 2H, CH2, J = 8.9, J′ = 7.6 Hz); 3.81–3.85 (m 2H, CH2); 13C NMR (DMSO-d6, 75 MHz): δ = 40.61 (CH2), 43.5 (CH2), 46.1 (CH2), 118.0, 119.1, 120.22, 121.0, 122.8, 123.5 (C-Ar), 164.8 (C-2), 171.5 (C = O), 172.1 (C = O); EIMS m/z 296.2 [M + H]+. HREIMS (m/z): 295.7410 [M+] (Calcd for C12H14ClN5O2 295.7410); Anal Calcd for: C12H14ClN5O2 C, 48.78; H, 4.77; Cl, 11.99; N, 23.68. Found C, 48.85; H, 4.87; Cl, 11.95; N, 23.76.
2- {[(1-(4-Chlorophenyl)imidazolidin-2-ylidene)carbamoyl]amino}acetamide (4c)
Ethyl {[1-(chlorophenylimidazolidin-2-ylidene)carbamoyl]amino}acetate (3c) (0.01 mol) was dissolved in 50 ml of methanol and added to 20% aqueous solution of ammonia. The mixture was shaken for 6 h at room temperature. The solid product was filtrated off and recrystallized from propan-2-ol. 1.24 g of (4c) was obtained (42% yield), white crystalline solid, m.p. 219–221 °C; 1H NMR (DMSO-d6, 300 MHz): δ = 9.97–9.98 (s, 1H, NH); 8.59–8.61 (s, 1H, NH); 7.88–7.97 (d, 2H, NH2); 7.25–7.65 (m, 4H, H-Ar); 4.11 (dd, 2H, CH2, J = 8.9, J′ = 7.5 Hz); 4.19 (dd, 2H, CH2, J = 8.9, J′ = 7.5 Hz); 3.66–3.80 (m 2H, CH2); 13C NMR (DMSO-d6, 75 MHz): δ = 41.2 (CH2), 43.4 (CH2), 46.9 (CH2), 165.9 (C-2), 120.0, 120.5, 120.9, 121.1, 122.8, 123.1 (C-Ar), 172.3 (C = O), 173.1 (C = O); EIMS m/z 296.1 [M + H]+. HREIMS (m/z): 295.7320 [M+] (Calcd for C12H14ClN5O2 295.7410); Anal Calcd for: C12H14ClN5O2 C, 48.74; H, 4.77; Cl, 11.99; N, 23.68. Found C, 48.68; H, 4.67; Cl, 11.88; N, 23.81.
2-{[(1-(2,6-Dichlorophenyl)imidazolidin-2-ylidene)carbamoyl]amino}acetamide (4d)
Ethyl {[1-(2,6-dichlorophenylimidazolidin-2-ylidene)carbamoyl]amino}acetate (3d) (0.01 mol) was dissolved in 50 ml of methanol and added to 20% aqueous solution of ammonia. The mixture was shaken for 6 h at room temperature. The solid product was filtrated off and recrystallized from propan-2-ol. 1.22 g of (4d) was obtained (37% yield), white crystalline solid, m.p. 113–115 °C; 1H NMR (DMSO-d6, 300 MHz): δ = 9.76–9.78 (s, 1H, NH); 8.89–8.91 (s, 1H, NH); 7.80–7.85 (d, 2H, NH2); 7.16–7.54 (m, 3H, H-Ar); 4.25 (dd, 2H, CH2, J = 9.0, J′ = 7.5 Hz); 4.39 (dd, 2H, CH2, J = 9.0, J′ = 7.5 Hz); 3.81–3.91 (m 2H, CH2); 13C NMR (DMSO-d6, 75 MHz): δ = 40.1 CH2, 43.9 (CH2), 46.0 (CH2), 119.54, 120.6, 121.8, 122.1, 122.8, 124.4 (C-Ar), 165.2 (C-2), 171.2 (C = O), 172.1 (C = O); EIMS m/z 331.4 [M + H]+. HREIMS (m/z): 330.2640[M+] (Calcd for C12H13Cl2N5O2 330.1900); Anal Calcd for: C12H13Cl2N5O2 C, 43.65; H, 3.97; Cl, 21.48; N, 21.21. Found C, 43.82; H, 4.05; Cl, 21.46, N, 21.32.
2-{[(1-(4-Methoxyphenyl)imidazolidin-2-ylidene)carbamoyl]amino}acetamide (4e)
Ethyl {[(1-phenylimidazolidin-2-ylidene)carbamoyl]amino}acetate (3e) (0.01 mol) was dissolved in 50 ml of methanol and added to 20% aqueous solution of ammonia. The mixture was shaken for 6 h at room temperature. The solid product was filtrated off and recrystallized from propan-2-ol. 1.63 g of (4e) was obtained (56% yield), white crystalline solid, m.p. 192–194 °C; 1H NMR (DMSO-d6, 300 MHz): δ = 9.84–9.86 (s, 1H, NH); 8.56–8.62 (s, 1H, NH); 8.08–8.15 (d, 2H, NH2); 7.56–7.78 (m, 4H, H-Ar); 4.01 (dd, 2H, CH2, J = 8.9, J′ = 7.5 Hz); 4.19 (dd, 2H, CH2, J = 8.9, J′ = 7.5 Hz); 3.66–3.80 (m 2H, CH2); 3.53–3.56 (s, 3H, OCH3); 13C NMR (DMSO-d6, 75 MHz): δ = 22.5 (OCH3), 40.4 (CH2), 43.6 (CH2), 46.5 (CH2), 119.0, 120.1, 121.2, 122.0, 122.8, 123.4 (C-Ar), 164.9 (C-2), 171.7 (C = O), 172.7 (C = O); EIMS m/z 292.2 [M + H]+. HREIMS (m/z): 291.0920 [M+] MS (Calcd for C13H17N5O3 291.3190); Anal Calcd for: C13H17N5O3 C, 53.60; H, 5.88; N, 24.04. Found C, 53.63; H, 5.92; N, 24.11.
General procedure to obtain compounds (5a–5e)
A mixture containing appropriate 0.01 mol of ethyl {[(1-phenylimidazolidin-2-ylidene]carbamoyl]amino}acetates (3a–3e) and 0.01 mol (1.6 g) of 1,1′-carbonyldiimidazole (CDI) was dissolved in 50 ml of DMF and heated under reflux for 6 h. The solvent was removed by the low-pressure evaporation and the resulting solid was crystallized from a mixture of methanol and propan-2-ol (1:1).
Ethyl (2,4-dioxo-8-phenyl-2,6,7,8-tetrahydroimidazo[1,2-a][1,3,5]triazin-3(4H)-yl)acetate (5a)
A mixture containing 2.90 g (0.01 mol) of ethyl {[(1-phenylimidazolidin-2-ylidene)carbamoyl]amino}acetate (3a) and 1.6 g (0.01 mol) of 1.1′carbonyldiimidazole (CDI) was dissolved in 50 ml of DMF and heated under reflux for 6 h. The solvent was removed by the low-pressure evaporation and the resulting solid was crystallized from a mixture of methanol and propan-2-ol (1:1). 1.77 g of (5a) was obtained (56% yield), white crystalline solid, m.p. 192–195 °C; 1H NMR (DMSO-d6, 300 MHz): δ = 7.12–7.85 (m, 5H, H-Ar); 4.11 (dd, 2H, CH2, J = 8.9, J′ = 7.4 Hz); 4.48 (dd, 2H, CH2, J = 8.9, J′ = 7.4 Hz); 4.20–4.26 (m, 2H, CH2); 3.81–3.97 (m, 2H, CH2), 1.02–1.22 (t, 3H, CH3, J = 7.2 Hz); 13C NMR (75 MHz, DMSO-d6, 75 MHz): δ = 15.1 (CH3), 16.6 (CH2), 40.5 (CH2), 43.1 (C-6), 45.4 (C-7), 118.5, 118.6, 119.9, 120.2, 129.7, 129.8 (C-Ar), 156.6 (C-8a), 166.4 (C = O), 166.9 (C = O), 170.6 (C = O); EIMS m/z 317.1 [M + H]+. HREIMS (m/z) 316.1726 [M+] (Calcd for C15H16N4O4 316.3250); Anal Calcd for: C15H16N4O4 C, 56.95; H, 5.10; N, 17.71. Found C, 56.83; H, 4.99; N, 17.79.
Ethyl {[2,4-dioxo-8-(3-chlorophenyl)-2,6,7,8-tetrahydroimidazo[1,2-a][1,3,5]triazin-3(4H)-yl}acetate (5b)
A mixture containing 3.24 g (0.01 mol) of ethyl {[1-(3-chlorophenylimidazolidin-2-ylidene)carbamoyl]amino}acetate (3b) and 1.6 g (0.01 mol) of 1,1′-carbonyldiimidazole (CDI) was dissolved in 50 ml of DMF and heated under reflux for 6 h. The solvent was removed by the low-pressure evaporation and the resulting solid was crystallized from a mixture of methanol and propan-2-ol (1:1). 1.61 g of (5b) was obtained (46% yield), white crystalline solid, m.p. 288–290 °C; 1H NMR (DMSO-d6, 300 MHz): δ = 7.50–7.78 (m, 4H, H-Ar); 4.00 (dd, 2H, CH2, J = 8.9, J′ = 7.5 Hz); 4.19 (dd, 2H, CH2, J = 8.9, J′ = 7.5 Hz); 3.81–3.97 (m 2H, CH2); 3.42–3.78 (m, 2H, CH2), 1.03–1.15 (t, 3H, CH3, J = 7.0 Hz); 13C NMR (DMSO-d6, 75 MHz): δ = 14.8 (CH3), 39.5 (CH2), 40.2 (CH2), 44.6 (C-6), 45.2 (C-7), 119.3, 119.3, 121.0, 121.3, 121.4, 122.1 (C-Ar), 157.5 (C-8a), 165.9 (C = O), 166.8 (C = O), 166.8 (C = O); EIMS m/z 351.1 [M + H]+. HREIMS (m/z) 350.1284 [M+] (Calcd for C15H15ClN4O4 350.7740); Anal Calcd for: C15H15ClN4O4 C, 51.36; H, 4.31; N, 15.97; Cl, 10.11. Found C, 51.40; H, 4.41; N, 15.95; Cl, 10.14.
Ethyl {[2,4-dioxo-8-(4-chlorophenyl)-2,6,7,8-tetrahydroimidazo[1,2-a][1,3,5]triazin-3(4H)-yl}acetate, (5c)
A mixture containing 3.24 g (0.01 mol) of ethyl {[1-(4-chlorophenylimidazolidin-2-ylidene)carbamoyl]amino}acetate (3c) and 1.6 g (0.01 mol) of 1,1′-carbonyldiimidazole (CDI) were dissolved in 50 ml of DMF and heated under reflux for 6 h. The solvent was removed by the low-pressure evaporation and the resulting solid was crystallized from a mixture of methanol and propan-2-ol (1:1). 2.14 g of (5c) was obtained (61% yield), white crystalline solid, m.p. 258–260 °C; 1H NMR (DMSO-d6, 300 MHz): δ = 7.4 9–7.87 (m, 4H, H-Ar); 3.93 (dd, 2H, CH2, J = 9.0, J′ = 7.6 Hz); 4.12 (dd, 2H, CH2, J = 9.0, J′ = 7.6 Hz); 3.41–3.4 6 (m 2H, CH2); 3.16–3.21 (s, 2H, CH2), 1.04–1.11 (t, 3H, CH3, J = 7.1 Hz); 13C NMR (DMSO-d6, 75 MHz): δ = 14.5 (CH3), 40.5 (CH2), 41.7 (CH2), 42.3 (C-6), 45.4 (C-7), 119.9, 120.2, 121.4, 121.7, 122.6, 123.8 (C-Ar), 157.6 (C-8a), 171.1 (C = O), 171.4 (C = O), 172.4 (C = O); EIMS m/z 351.3 [M + H]+. HREIMS (m/z): 350.1235 [M+] (Calcd for C15H15ClN4O4 350.7740); Anal Calcd for: C15H15ClN4O4 C, 51.36; H, 4.31; N, 15.97; Cl, 10.11. Found C, 51.29; H, 4.28; N, 15.86; Cl, 10.19.
Ethyl {[2,4-dioxo-8-(2,5-dichlorophenyl)-2,6,7,8-tetrahydroimidazo[1,2-a][1,3,5]triazin-3(4H)-yl}acetate (5d)
A mixture containing 3.59 g (0.01 mol) of ethyl {[(1-(2,5-dichlorophenylimidazolidin-2-ylidene)carbamoyl]amino}acetate (3d) and 1.6 g (0.01 mol) of 1,1′-carbonyldiimidazole (CDI) was dissolved in 50 ml of DMF and heated under reflux for 6 h. The solvent was removed by the low-pressure evaporation and the resulting solid was crystallized from a mixture of methanol and propan-2-ol (1:1). 2.54 g of (5d) (66% yield) was obtained, white crystalline solid, m.p. 221–223 °C; 1H NMR (DMSO-d6, 300 MHz): δ = 7.18–7.64 (m, 3H, H-Ar); 4.02 (dd, 2H, CH2, J = 8.9, J′ = 7.5 Hz); 4.23 (dd, 2H, CH2, J = 8.9, J′ = 7.5 Hz); 3.92–3.98 (m 2H, CH2); 3.43–3.61 (s, 2H, CH2), 1.20–1.31 (t, 3H, CH3, J = 7.0 Hz); 13C NMR (DMSO-d6, 75 MHz): δ = 13.8 (CH3), 40.2 (CH2), 41.9 (CH2), 42.3 (C-6), 45.8 (C-7), 119.9, 120.2, 121.6, 122.6, 123.3, 124.6 (C-Ar), 169.9 (C-8a), 169.9 (C = O), 170.7 (C = O), 171.1 (C = O); EIMS m/z 386.1 [M + H]+. HREIMS (m/z): 385.3328 [M+] (Calcd for C15H14Cl2N4O4 385.2230); Anal Calcd for: C15H14Cl2N4O4 C, 46.77; H, 3.66; Cl, 18.41; N, 14.54. Found C, 46.82; H, 3.59; Cl, 18.39, N, 14.61.
Ethyl {[2,4-dioxo-8-(4-methoxyphenyl)-2,6,7,8-tetrahydroimidazo[1,2-a][1,3,5]triazin-3(4H)-yl}acetate (5e)
A mixture containing 3.20 g (0.01 mol) of ethyl {[(1-(4-methoxyphenylimidazolidin-2-ylidene)]carbamoyl]amino}acetate (3e) and 1.6 g (0.01 mol) of 1,1′-carbonyldiimidazole (CDI) was dissolved in 50 ml of DMF and heated under reflux for 6 h. The solvent was removed by the low-pressure evaporation and the resulting solid was crystallized from a mixture of methanol and propan-2-ol (1:1). 2.11 g of (5e) was obtained (60% yield), white crystalline solid, m.p. 194–194 °C; 1H NMR (DMSO-d6, 300 MHz): δ = 6.91–7.68 (m, 4H, H-Ar); 4.04 (2dd, 2H, CH2, J = 8.9, J′ = 7.6 Hz); 4.20 (2dd, 2H, CH2, J = 8.9, J′ = 7.6 Hz); 3.65–3.86 (m 2H, CH2); 3.48–3.51 (s, 2H, CH2), 3.45 (s, 3H, OCH3); 1.26–1.31 (t, 3H, CH3, J = 7.1 Hz); 13C NMR (DMSO-d6, 75 MHz): δ = 14.9 (CH3), 22.4 (OCH3), 40.2 (CH2), 41.9 (CH2), 42.3 (C-6), 45.8 (C-7), 120.1, 120.5, 121.2, 122.9, 123.2, 125.6 (C-Ar), 157.6 (C-8a), 169.5 (C = O), 170.7 (C = O), 171.5 (C = O); EIMS m/z 347.4 [M + H]+. HREIMS (m/z): 346.1288 [M+] (Calcd for C16H18N4O5 346.3520); Anal Calcd for: C16H18N4O5 C, 55.48; H, 5.24; N, 20.22. Found C, 55.44; H, 4.31; N, 20.30.
General procedure to obtain compounds 6a–6e
A mixture containing appropriate 2-{[(1-phenylimidazolidin-2-ylidene)carbamo-yl]amino}acetamide (4a–4e) (0.01 mol) and 1.6 g (0.01 mol) of 1,1′-carbonyldiimidazole (CDI) was dissolved in 50 ml of DMF and heated under reflux for 8 h. The solvent was removed by the low-pressure evaporation and the resulting solid was crystallized from a mixture of methanol and propan-2-ol (1:3).
2-{(2,4-Dioxo-8-phenyl-2,6,7,8-tetrahydroimidazo[1,2-a][1,3,5]triazin-3(4H)-yl)}acetamide (6a)
A mixture containing 2.61 g (0.01 mol) of 2-{[(1-phenylimidazolidin-2-ylidene)carbamoyl]amino}acetamide (4a) and 1.6 g (0.01 mol) of 1,1′-carbonyldiimidazole (CDI) was dissolved in 50 ml of DMF and heated under reflux for 8 h. The solvent was removed by the low-pressure evaporation and the resulting solid was crystallized from a mixture of methanol and propan-2-ol (1:3). 1.41 g of 6a was obtained (49% yield), white crystalline solid, m.p. 246–248 °C; 1H NMR (DMSO-d6, 300 MHz): δ = 8.89–9.11 (d, 2H, NH2); 7.44–7.98 (m, 5H, H-Ar); 4.01 (dd, 2H, CH2, J = 8.9, J′ = 7.4 Hz); −4.12 (dd, 2H, CH2, J = 8.9, J′ = 7.4 Hz); 3.76–3.87 (m 2H, CH2); 13C NMR (DMSO-d6, 75 MHz): δ = 40.4 (CH2), 43.5 (C-6), 46.0 (C-7), 119.9, 120.2, 122.09, 123.4, 123.7, 124.0 (C-Ar), 157.6 (C-8a), 169.2 (C = O), 170.0 (C = O), 170.7 (C = O); EIMS m/z 288.2 [M + H]+. HREIMS (m/z): 287.1069 [M+] (Calcd for C13H13N5O3 287.2870); Anal Calcd for: C13H13N5O3 C, 54.35; H, 4.46; N, 24.38. Found C, 54.63; H, 4.82; N, 24.55.
2-{[2,4-Dioxo-8-(3-chlorophenyl)-2,6,7,8-tetrahydroimidazo[1,2-a][1,3,5]triazin-3(4H)-yl]}acetamide, (6b)
A mixture containing 2.95 g (0.01 mol) of 2-{[(1-(3-chlorophenyl)imidazolidin-2-ylidene)carbamoyl]amino}acetamide (4b) and 1.6 g (0.01 mol) of 1,1′-carbonyldiimidazole (CDI) was dissolved in 50 ml of DMF and heated under reflux for 8 h. The solvent was removed by the low-pressure evaporation and the resulting solid was crystallized from a mixture of methanol and propan-2-ol (1:3). 1.54 g of (6b) was obtained (48% yield), white crystalline solid, m.p. 285–287 °C; 1H NMR (DMSO-d6, 300 MHz): δ = 8.68–8.77 (d, 2H, NH2); 6.91–7.68 (m, 4H, H-Ar); 4.14 (dd, 2H, CH2, J = 8.9, J′ = 7.5 Hz); 4.22 (dd, 2H, CH2, J = 8.9, J′ = 7.5 Hz); 3.07–3.96 (m 2H, CH2); 13C NMR (75 MHz, DMSO-d6): δ = 40.1 (CH2), 43.5 (C-6), 46.2 (C-7), 119.1, 120.2, 121,3, 121.9, 122.0, 123.1 (C-Ar), 157.6 (C-8a), 168.7 (C = O), 171.2 (C = O), 170.4 (C = O); EIMS m/z 322.1 [M + H]+. HREIMS (m/z): 321.0583 [M+] (Calcd for C13H12ClN5O3 321.7360); Anal Calcd for: C13H12ClN5O3 C, 48.53; H, 4.07; Cl, 11.02, N, 21.77. Found C, 48.44; H, 4.11; Cl, 11.32; N, 21.73.
2-{[2,4-Dioxo-8-(4-chlorophenyl)-2,6,7,8-tetrahydroimidazo[1,2-a][1,3,5]triazin-3(4H)-yl]}acetamide, (6c)
A mixture containing 2.95 g (0.01 mol) of 2-{[(1-(4-chlorophenyl)imidazolidin-2-ylide-ne)carbamoyl]amino}acetamide (4c) and 1.6 g (0.01 mol) of 1,1′-carbonyldiimidazole (CDI) was dissolved in 50 ml of DMF and heated under reflux for 8 h. The solvent was removed by the low-pressure evaporation and the resulting solid was crystallized from a mixture of methanol and propan-2-ol (1:3). 1.61 g of (6c) was obtained (50% yield), white crystalline solid, m.p. 310–312 °C; 1H NMR (DMSO-d6, 300 MHz): δ = 9.22–9.30 (d, 2H, NH2); 7.11–7.69 (m, 4H, H-Ar); 4.00 (dd, 2H, CH2, J = 8.9, J′ = 7.6 Hz); 4.16 (dd, 2H, CH2, J = 8.9, J′ = 7.6 Hz); 3.66–3.80 (m 2H, CH2); 13C NMR (75 MHz, DMSO-d6): δ = 40.6 (CH2), 43.6 (C-6), 45.8 (C-7), 120.6, 120.8, 123.7, 124.3, 124.8, 125.0 (C-Ar), 157.1 (C-8a), 169.2 (C = O), 171.7 (C = O), 172.1 (C = O); EIMS m/z 322.3 [M + H]+. HREIMS (m/z): 321.1579 [M+] (Calcd for C13H12ClN5O3 321.7360); Anal Calcd for: C13H12ClN5O3 C, 48.53; H, 4.07; Cl, 11.02; N, 21.77. Found C, 48.40; H, 4.41; Cl, 11.14; N, 21.95.
2{-[2,4-Dioxo-8-(2,6-dichlorophenyl)-2,6,7,8-tetrahydroimidazo[1,2-a][1,3,5]triazin-3(4H)-yl]}acetamide (6d)
A mixture containing 3.30 g (0.01 mol) of 2-{[(1-(2,6-dichlorophenyl)imidazolidin-2-ylidene)carbamoyl]amino}acetamide (4d) and 1.6 g (0.01 mol) of 1,1′-carbonyldiimidazole (CDI) were dissolved in 50 ml of DMF and heated under reflux for 8 h. The solvent was removed by the low-pressure evaporation and the resulting solid was crystallized from a mixture of methanol and propan-2-ol (1:3). 2.24 g of (6d) was obtained (63% yield), white crystalline solid, m.p. 274–276 °C; 1H NMR (DMSO-d6, 300 MHz): δ = 8.08–8.15 (d, 2H, NH2); 6.98–7.42 (m, 4H, H-Ar); 4.04 (dd, 2H, CH2, J = 9.0, J′ = 7.5 Hz); 4.19 (dd, 2H, CH2, J = 9.0, J′ = 7.5 Hz); 3.40–3.51 (m 2H, CH2); 13C NMR (DMSO-d6, 75 MHz): δ = 39.4 (CH2), 43.7 (C-6), 46.6 (C-7), 120.7, 121.2, 123.9, 124.4, 125.0 125.3 (C-Ar), 157.4 (C-8a), 168.9 (C = O), 171.9 (C = O), 172.2 (C = O); EIMS m/z 357.1 [M + H]+. HREIMS (m/z): 356.2640 [M+] (Calcd for C13H11Cl2N5O3 356.1850); Anal Calcd for: C13H11Cl2N5O3 C, 43.84; H, 3.11; Cl, 19.91, N, 19.66. Found C, 43.29; H, 3.28; Cl, 20.09; N, 19.86.
2-{[2,4-Dioxo-8-(4-methoxyphenyl)-2,6,7,8-tetrahydroimidazo[1,2-a][1,3,5]triazin-3(4H)-yl]}acetamide (6e)
A mixture containing 2.91 g (0.01 mol) of 2-{[(1-(4-methoxyphenyl)imidazolidin-2-ylidene)carbamoyl]amino}acetamide (4e) and 1.6 g (0.01 mol) of 1,1′-carbonyldiimidazole (CDI) was dissolved in 50 ml of DMF and heated under reflux for 8 h. The solvent was removed by the low-pressure evaporation and the resulting solid was crystallized from a mixture of methanol and propan-2-ol (1:3). 1.33 g of (6e) was obtained (42% yield), white crystalline solid, m.p. 263–265 °C; 1H NMR (DMSO-d6, 300 MHz): δ = 8.08–8.15 (d, 2H, NH2); 7.05–7.68 (m, 4H, H-Ar); 4.05 (dd, 2H, CH2, J = 8.9, J′ = 7.5 Hz); 4.20 (dd, 2H, CH2, J = 8.9, J′ = 7.5 Hz); 3.36–3.42 (m 2H, CH2), 3.28 (s, 3H, OCH3); 13C NMR (DMSO-d6, 75 MHz): δ = 22.5 (OCH3), 40.6 (CH2), 43.5 (C-6), 46.1 (C-7), 119.3, 121.0, 121.2, 121.7, 122.0, 123.1 (C-Ar), 157.9 (C-8a), 169.1 (C = O), 170.8 (C = O), 172.6 (C = O); EIMS m/z 318.2 [M + H]+. HREIMS (m/z): 317.0584 [M+] (Calcd for C14H15N5O4 317.3140); Anal Calcd for: C14H15N5O4 C, 52.99; H, 4.77; N, 22.07. Found C, 53.02; H, 4.61; N, 22.19.
Molecular modeling
The investigated compounds were modeled using the LigPrep protocol from the Schrödinger SuiteCitation24. In order to sample different protonation states of ligands in physiological pH, Epik module was usedCitation25. Parameters to evaluate drug-likeness were calculated using VegaZZ v. 3.0.1Citation26 (number of atoms), Discovery Studio v. 3.1.Citation27 (molar mass, number of rings, lipophilicity, number of rotatable bonds), ACDLabs (molar refractivity, number of hydrogen bond donors and acceptors), and MOE Molecular EnvironmentCitation28 (a number of rigid bonds) as described previouslyCitation2,Citation12. Drug-likeness was also evaluated with Osiris Property ExplorerCitation29. This approach is based on a list of about 5300 distinct substructure fragments with associated drug-likeness scores. The drug-likeness is calculated summing up score values of those fragments that are present in the molecule under investigation. ADMET parameters were calculated with Discovery Studio 3.1 (solubility, blood–brain permeation) or Osiris Property ExplorerCitation29 (toxicity risks). The prediction of toxicity by this tool relies on a precomputed set of structural fragment that give rise to toxicity alerts in case they are encountered in the investigated structure. For structure–activity relationship studies, HOMO and LUMO energies and polar surface area were calculated with Discovery Studio 3.1 as reported earlierCitation2,Citation12. Molar volume and polarizability were calculated with ACDLabs softwareCitation2,Citation12. The maps of the electrostatic potential (ESP) onto a surface of the electron density were visualized with ArgusLabCitation30.
Pharmacology
Animals
The experiments were performed on male Albino Swiss mice (18–30 g). The animals were kept 8–10 to a cage, at room temperature of 20 ± 1 °C, on a 12:12 h dark–light cycle. Standard food (LSM) and water were available ad libitum. The experiments were performed between 9:00 a.m. and 4:00 p.m., in accordance with the opinion of Local Ethics Committee for Animal Experimentation. After 1 week of adaptation and handling, the animals were divided into groups and prepared for the tests. Each group consisted of 8–10 mice.
Drugs and treatment
Pentylenetrazole (PTZ) was bought from Polfa, whereas naloxone, D-amphetamine sulphate, and 5-hydroxytryptophan (L-5-HTP) were purchased from Sigma, St. Louis, MO.
In all tests the investigated compounds (3b, 3c, 3e, 4c, 5a, 5e, 6c, 6d, 6e) were administered systemically – intraperitoneally (i.p.), or subcutaneously (s.c.) in volume of 10 ml/kg as a suspensions in aqueous solution of 0.5% methylcellulose (tylose) in the doses equivalent to 0.1, 0.05 and 0.025 ED50 and were injected 60 min before the tests. The control animals received an equivalent volume of the solvent at the respective time before the test. All tests performed, as suggested by Vogel and VogelCitation31 are generally accepted as basic in investigation of the central activity by behavioral methods.
The toxicity of the compounds was assessed in mice according to Litchfield and Wilcoxon methodCitation32, as the ED50 calculated on the loss of the righting reflex within 48 h.
Behavioral experiments
Nociceptive reactions were studied in the acetic acid-induced writhing testCitation33. This test is a chemical method, used to study pain, induced by i.p. injection of irritant acetic acid (0.6%), as a nociceptive stimulus, inducing characteristic writhing episodes. It is possible to detect even very weak antinociceptive agents using this method. It is also considered as an experimental model closest to the nature of clinical pain. Furthermore, it enables evaluation of analgesic action of both central and peripheral origin. However, this method has some limitations: in this test, it is difficult to determine the length of antinociceptive activity, and the test is not specific, so it can show an analgesic effect for many substancesCitation31,Citation34. In this test all the investigated compounds were administered s.c. 50 min before injection of acetic acid i.p., and 5 min after acetic acid administration, the number of writhing episodes was recorded for a period of 10 min. In this test, the influence of naloxone on the antinociceptive effect of the compounds was also assessed. For this purpose, naloxone (5 mg/kg, s.c.) was applied 20 min before acetic acid solution injection.
Spontaneous activity and amphetamine-induced hyperactivity in mice were measured in round actometer cages (32 cm in diameter, Multiserv, Lublin, Poland), which were kept in a sound-attenuated experimental room. The cages were equipped with one row of infrared light-sensitive photocells located 1 cm above the floor. The locomotor activity was measured for a single mouse, 60 min after compound injection, for a total period of 30 min as spontaneous activity and amphetamine-induced hyperactivity (for the latter one mice received s.c. 5 mg/kg of amphetamine 30 min before the test).
The motor coordination of mice was assessed in the rota-rod test (Ataner Zakład Usługowy Elektroniki Inż K. Fic, Lublin, Poland) and in the chimney test. Rota-rod testCitation35 assesses the ability of animals to keep balance on a rotating rod (20 mm diameter) during 60 s. The rod revolves with constant speed of 18 rpm. The time period that the mice were spent on the rotating rod, was measured and recorded. Chimney testCitation36 evaluates the ability of mice to go backwards, vertically from the tube. Accordingly, the time period that the mice were spent in a tube (maximum 60 s) was measured and recorded. The tube was made of Plexiglas with rough surface (30 mm diameter and 25 cm length). Both tests were performed 60 min after i.p. injection of the examined drugs, on the same mice, i.e. mice were removed from the rod and immediately fed to the chimney. Before these tests, the animals had been trained: each mouse was placed on the rod and in the chimney for 3 min. The number of trials for each mouse was unlimited. Then, consequently, only those animals were approved that were able to stay on the rotating rod for 60 s or were able to leave the chimney without a problem (up to 15 s).
Body temperature in normothermic mice was measured in the rectum of animals with a thermistor thermometer during the total period 240 min (60 min before and 180 min after drug injection). The average of the first two measurements (60 and 30 min before drug administration) was determined as an initial temperature (Δi). After the drug administration the final temperature (Δf) was measured six times every 30 min (up to 180 min) in each mice. The changes in body temperature (Δ) were calculated according to the formula: Δ = Δf−Δi. The changes of the body temperature were studied after administration of the compounds at the doses of 0.1 and 0.05 ED50 i.p.
In order to study pentylenetetrazole-induced (PTZ) seizures, a single dose of PTZ (110 mg/kg, i.p.) was administered to induce convulsions in mice. Animals were observed for a period of 30 min, 60 min post-PTZ administration in the glass chambers. Behavioral parameters such as clonic convulsions, tonic extensor, and mortality were observed and recorded.
Head twitch responses (HTR) after 5-hydroxy-l-tryptophan (L-5-HTP), were estimated according to Corne et al.Citation37. Mice were treated with L-5-HTP (230 mg/kg, i.p.) and immediately placed individually in clear glass chambers, and the number of HTR exhibited by each mouse during the next 1 h was recorded in six 2-min intervals (between 4 and 6, 14 and 16, 24 and 26, 34 and 36, 44 and 46 and 54 and 56 min). The HTR was characterized by abrupt lateral movements, which may or may not be accompanied by body twitches and hind limb retraction.
Statistical analysis
The obtained data were calculated by Fisher exact test (PTZ-induced seizures), two-way analysis of variance (ANOVA) and followed by a post-hoc confirmation with the Bonferroni test (body temperature), and one-way ANOVA followed by Dunnett’s post-hoc test (other tests). All results are presented as means ± SEM. p < 0.05 was considered as statistically significant.
Results and discussion
Chemistry
We have previously reported a few series of 1-aryl-4,5-dihydro-1H-imidazol-2-amines derivatives which do not possess a protonable nitrogen atom but exhibit serotoninergic and antinociceptive activity mediatedCitation2,Citation8–11 or notCitation12 through the opioid system. Encouraged by these results, we designed, synthesized and studied further four series of compounds (3a–3e, 4a–4e, 5a–5e, 6a–6e) (Scheme 1)Citation17–20. Compounds (3a–3e) were prepared in the reaction between respective 1-aryl-4,5-dihydro-1H-imidazol-2-amines (1a–1e) and ethyl isocyanatoacetate (2) in dichloromethane. Derivatives (4a–4e) resulted as the products of conversion of (3a–3e) into respective amides due to action of aqueous solution of ammonia. Compounds (3a–3e) and (4a–4e) were subjected to cyclization by heating in DMF which yielded imidazo[1,2-a][1,3,5]triazin derivatives (5a–5e) and (6a–6e), respectively.
Estimation of drug-likeness
The descriptors used for estimation of drug-likeness are collected in . Drug-likness was assessed using Lipinski’s rule as well as the placement of the investigated compounds in the chemical space determined by the databases of the pharmacologically active compounds (CMC, Comprehensive Medicinal Chemistry Database, containing about 7000 compounds and MDDR, MACCS-II Drug Data Report, containing about 100 000 compounds) according to the methodology of PREADMETCitation38 service as described previouslyCitation12. Concerning Lipinski’s rule, all the compounds possess the molar mass below 500, the number of hydrogen bond donors below 5, the number of hydrogen bond acceptors below 10, and the lipophilicity below 5. Optimal value of lipophilicity for drugs acting on CNS is from 2 to 4Citation39. Moreover, many other drug parameters are influenced by lipophilicity. High lipophilicity may cause high rapid metabolic turnover and low solubility and poor absorptionCitation39. As lipophilicity increases, there is an increased probability of binding to hydrophobic protein targets other than the desired one, and therefore, there is more potential for toxicity (cytochrome P450 inhibition and human ether-ago-go-related gene (hERG) K-channel blockade)Citation39. Concerning lipophilicity values compounds (3a–3e) and (5a–5e) are better candidates for central nervous drugs than (4a–4e) and (6a–6e).
Table 1. Parameters for drug-likeness estimation.
Regarding subsequent criteria of drug-likeness, most compounds collected in the CMC database has lipophilicity from −0.4 to 5.6, molar refractivity in the range of 40–130, molar mass from 160 to 480, and the number of atoms from 20 to 70Citation12. All the investigated compounds fulfill this criterion. Concerning the compounds in MDDR database, the drug-like substances have the number of rings equal or greater than 3, the number of rigid bonds equal or greater than 18, and the number of rotatable bonds equal or greater than 6Citation12. Thus, compounds (3a–3e) and (4a–4e) possess too few rings and too few rotatable bonds. Compounds (5a–5e) and (6a–6e) fulfill the first two conditions, but it may turn out favorable to increase the number of rotatable bonds which we will consider in the design of next series of compounds. Finally, molecule drug-likeness score (fragment-based score) was calculated using Osiris Property ExplorerCitation29. According to this score, compounds (4a–4b) and (6a–6b) are more drug-like than (3a–3e) and (5a–5e) which is opposite to their unfavorable lipophilicity values. In summary, the investigated compounds may be termed drug-like, and it is justified to test them in the in vivo experiments. Evaluation of drug-likeness has not allowed to exclude any compound series from in vivo experiments.
Prediction of ADMET properties
In order to facilitate the selection of compounds for animal studies, some ADMET parameters were calculated (). The plot presented in confirms that most of the tested compounds possess favorable ADMET properties, although some of them have borderline values. Comparing the plot in with lipophilicity values from and polar surface areas from , it can be concluded that compounds from series 4 and 6 (in particular (6e)) have less favorable blood–brain permeation properties. All compounds are well absorbed (), however compounds (5b), (5c), (5d) and (6d) are not enough soluble in water as they have values of logS below −4Citation29. Moreover, compounds from series 5 have low overall drug score which combines druglikeness, cLogP, logS, molecular weight and toxicity risks in one handy value than may be used to judge the compound's overall potential to qualify for a drugCitation29. Importantly, compounds from series 3 and 4 are non-toxic (all scores equal to 1.00 in ) whereas compounds from series 5 and 6 have irritant properties (score 0.6) but they are not mutagenic, tumorigenic and do not have reproductive effects. On the basis of calculation of ADMET parameters and our earlier experience on the effect of substituents on the activity, we decided to test nine compounds (3b, 3c, 3e, 4c, 5a, 5e, 6c, 6d, 6e). We decided to test only one compound from series 4 due to low lipophilicity values and poor blood–brain permeation for compounds in this series. In particular compound (6e) was incuded in the set as a validation of our in silico predictions.
Table 2. ADMET parameters of the studied compounds.
Table 3. The effect of the new compounds on the PTZ-induced clonic seizures, tonic convulsions and death of mice.
Pharmacology
Nine compounds (3b, 3c, 3e, 4c, 5a, 5e, 6c, 6d, 6e) were selected for pharmacological studies. The selection was made on the basis of the results for previous series of compounds and on the basis on in silco pre-screening as described above. The influence of these new urea derivatives on the CNS of laboratory animals has not been tested yet. The variety of behavioral tests were planned, as previously described in the Methods section.
The effects of the compounds on the spontaneous locomotor activity and amphetamine-evoked hyperactivity were estimated. Analgesic activity, without or in the presence of naloxone, was quantified in the writhing test. The tendency to diminish the HTR, evoked by L-5-HTP and the involvement in alteration in body temperature in mice was studied. Additionally, to check possible occurrence of drug-induced changes in the muscle relaxant activity of mice, which may have contributed to their behavior in other tests, the rota-rod and chimney tests were performed. These allowed preliminary determination of the impact of the new substances on the CNS of experimental animals.
It was found out that the tested compounds are characterized by either very low, lower than 2000 mg/kg i.p. (series 3, 5 and 6 (except for (6c) compound)) or low toxicity (1620 for (4c) compound and 774 mg/kg i.p. for 6c compound calculated on the loss of the righting reflex within 48 h. These values of ED50 were adopted for further study.
All four series of compounds investigated exhibit different activity spectra, which is most probably an effect of their structural diversity. The most significant influence on the CNS of laboratory animals was observed after administration of derivatives of series 3 (3b, 3c, 3e) and 5 (5a, 5e).
The effect of the compounds from series 3 and 5 on spontaneous activity and amphetamine-induced hyperactivity
The one-way ANOVA showed significant changes in the locomotor activity of studied mice [F(11,87) = 4.657; p < 0.0001]. The post-hoc Dunnett test confirmed a significant reduction in motility of mice after the administration of series 3 compounds at the dose of 0.1 ED50: (3b) (p < 0.01), and (3c, 3e) ED50 (p < 0.05) and series 5 compounds: (5a) at the dose of 0.1 ED50 (p < 0.001) and (5e) at the doses of 0.1 and 0.05 ED50 (p < 0.01) versus the vehicle treated group (). Locomotor activity of mice was decreased by all compounds from series 3 and 5, which seem to suggest their depressive effects on the CNSCitation31, while none of them changed the hyperactivity caused by administration of amphetamine (data not presented).
Figure 6. The influence of compounds from series 3 and 5 compounds on the spontaneous motor activity of mice. One-way ANOVA showed significant changes in locomotor activity of mice [F(11,87) = 4.657; p < 0.0001]. The post-hoc Dunnett’s test confirmed a significant reduction in motility of mice after the administration of series 3 compounds at the dose of 0.1 ED50: (3b) (p < 0.01), and (3c, 3e) ED50 (p < 0.05) and series 5 compounds: (5a) at the dose of 0.1 ED50 (p < 0.001) and (5e) at the doses of 0.1 and 0.05 ED50 (p < 0.01).
![Figure 6. The influence of compounds from series 3 and 5 compounds on the spontaneous motor activity of mice. One-way ANOVA showed significant changes in locomotor activity of mice [F(11,87) = 4.657; p < 0.0001]. The post-hoc Dunnett’s test confirmed a significant reduction in motility of mice after the administration of series 3 compounds at the dose of 0.1 ED50: (3b) (p < 0.01), and (3c, 3e) ED50 (p < 0.05) and series 5 compounds: (5a) at the dose of 0.1 ED50 (p < 0.001) and (5e) at the doses of 0.1 and 0.05 ED50 (p < 0.01).](/cms/asset/5daeb542-e722-47d2-98b1-a05d9329a92e/ienz_a_965699_f0006_b.jpg)
The effect of the compounds from series 3 and 5 in the writhing test
The one-way analysis revealed significant changes in the number of writhing episodes of mice, F(5,42) = 2.766, p < 0.05. Post-hoc Dunnett’s test confirmed a significant antinociceptive effect (reduction in the writhing episodes of mice) after the administration of compound 3b at the dose of 0.1 ED50 (p < 0.05) and compounds: 5a at the doses of 0.1 and 0.05 ED50 (p < 0.05) and 5e at the doses of 0.1 and 0.05 ED50 (p < 0.05 and p < 0.01, respectively) ().
Figure 7. The influence of compounds from series 3 and 5 on the nociceptive reactions studied in the acetic acid (0.6%)-induced writhing test. One-way ANOVA showed significant changes in the number of writhing episodes of mice (F(5,42) = 2.766, p < 0.05). Post-hoc Dunnett’s test confirmed a significant reduction in the writhing episodes of mice after the administration of compound 3b at the dose of 0.1 ED50 (p < 0.05) and compounds: 5a at the doses of 0.1 and 0.05 ED50 (p < 0.05) and 5e at the doses of 0.1 and 0.05 ED50 (p < 0.0 and p < 0.01, respectively).
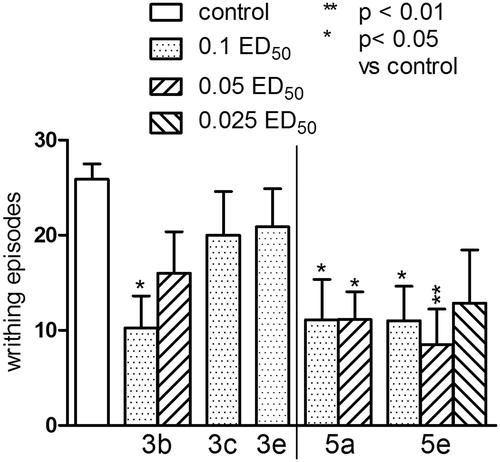
The effect of naloxone on antinociceptive activity of the compounds from series 5 in the writhing test
One-way ANOVA showed significant changes in the number of writhing episodes of mice after the administration of compounds (5a) and (5e) and co-administration of these compounds with naloxone, F(4,31) = 4.073, p < 0.01. Post-hoc Dunnett’s test showed a significant reduction in the writhing episodes of mice after the administration of the compound (5a) and (5e) at the dose of 0.05 ED50 (p < 0.05 and p < 0.001, respectively). Pretreatment with naloxone did not affect the numer of writhing episodes as compared to respective compounds tested (). The antinociceptive activity of the tested compounds does not appear to be associated with endogenous opioid system, because naloxone (5 mg/kg) – non-selective opioid receptor antagonistCitation40 did not alter the observed effects.
Figure 8. The influence of naloxone, 5 mg/kg, s.c. on antinociceptive activity of series 5 (compounds 5a and 5e), evaluated in the acetic acid (0.6%)-induced writhing test. One-way ANOVA showed significant changes in the number of writhing episodes of mice after the administration of compounds 5a and 5e and coadministration of these compounds with naloxone (F(4,31) = 4.073, p < 0.01). Post-hoc Dunnett’s test showed a significant reduction in the writhing episodes of mice after the administration of the compounds 5a and 5e at the dose of 0.1 ED50 (p < 0.05 and p < 0.001, respectively). Pretreatment with naloxone did not affect the numer of writhing episodes as compared to respective compounds tested.
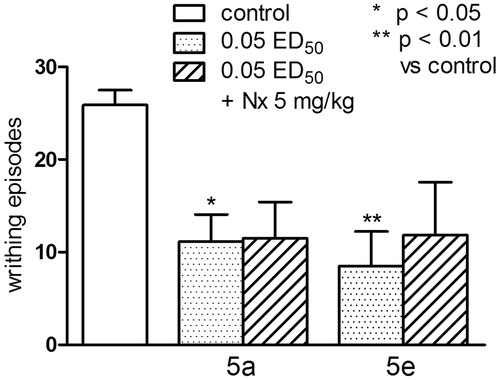
The effect of the compounds from series 3 and 5 on the head twitch responses (HTR) after L-5-HTP
Furthermore, tests were also carried out to evaluate the effect of the new derivatives on the HTR in mice caused by administration of a serotonin precursor, L-5-HTP (230 mg/kg), which may indicate the involvement of the serotonergic system in the observed effects. One-way ANOVA showed significant changes in the number of HTR [F(5,42) = 2.460; p < 0.05]. Post-hoc Dunnett’s test confirmed a significant reduction in HTR of mice after the administration of compound (3e) at the dose of 0.1 ED50 (p < 0.05). The HTR were also decreased, markedly, but not significantly by (5a) and (5e), while the compounds (3b) and (3c) did not produce any significant effects on HRT ().
Figure 9. The influence of series 3 and 5 compounds on the head twitch responses (HTR) evoked by 5-hydroxy-L-tryptophan (L-5-HTP, 230 mg/kg). One-way ANOVA showed significant changes in the number of HTR [F(5,42) = 2.460; p < 0.05]. Post-hoc Dunnett’s test confirmed a significant reduction in HTR of mice after the administration of compound (3e) at the dose of 0.1 ED50 (p < 0.05).
![Figure 9. The influence of series 3 and 5 compounds on the head twitch responses (HTR) evoked by 5-hydroxy-L-tryptophan (L-5-HTP, 230 mg/kg). One-way ANOVA showed significant changes in the number of HTR [F(5,42) = 2.460; p < 0.05]. Post-hoc Dunnett’s test confirmed a significant reduction in HTR of mice after the administration of compound (3e) at the dose of 0.1 ED50 (p < 0.05).](/cms/asset/0607908e-be94-461e-b8a1-85bc8ed59e10/ienz_a_965699_f0009_b.jpg)
The effect of the compounds from series 3 and 5 on the changes in body temperature
In addition, compounds from series 3 (3c) and 5 (5a, 5e) substantially decreased the body temperature of normothermic mice, which also may confirm the involvement of serotonergic system. Serotonin has been reported to play an important role in central regulation of body temperatureCitation41. Hypothermia in rodents has been reported for MAO (monoamine oxidase) type A enzyme inhibitors such as clorgylineCitation42 and harman (1-methyl-β-carboline)Citation43. As a result of MAO-inhibition, 5-HT levels in the body are increased and may precipitate a serotonin syndrome. Two-way ANOVA revealed significant effects for compounds from series 3 (3c) and 5 (5a, 5e). Post-hoc Bonferroni test confirmed that the substance (5e) given at the dose corresponding to 0.1 ED50 caused a very clear, statistically significant decrease in body temperature in mice (p < 0.001 from 30 to 60 min and p < 0.01 in 90 min). Also, administration of a lower dose of this substance (0.05 ED50) resulted in statistically significant reduction of the observed parameter, and this effect was also prolonged (p < 0.001 in 30 min and p < 0.05 from 60 to 180 min). Compound 5a at the dose of 0.1 ED50 caused a significant decrease in the body temperature of mice from 30 to 120 min (p < 0.001, p < 0.01, p < 0.01, p < 0.05 and compound 3c at the dose of 0.1 ED50 from 60 to 90 min (p < 0.05) of observation. Administration of other compounds practically did not affect the body temperature of animals ()
Figure 10. The influence of compounds 3c, 5a and 5e used at the doses of 0.1 and 0.05 ED50 on the body temperature of mice. Post-hoc Bonferroni test confirmed a significant decrease in the body temperature of mice after the administration of compound 5a at the dose of 0.1 ED50 from 30 to 120 min (p < 0.001, p < 0.01, p < 0.01, p < 0.05), (5e) at the dose of 0.1 ED50 from 30 to 90 min (p < 0.001, p < 0.001, p < 0.01) and at the dose of 0.05 ED50 from 30 to 90 min (p < 0.001 in 30 min, and p < 0.05 from 60 to 180 min), and compound 3c at the dose of 0.1 ED50 from 60 to 90 min (p < 0.05) of observation.
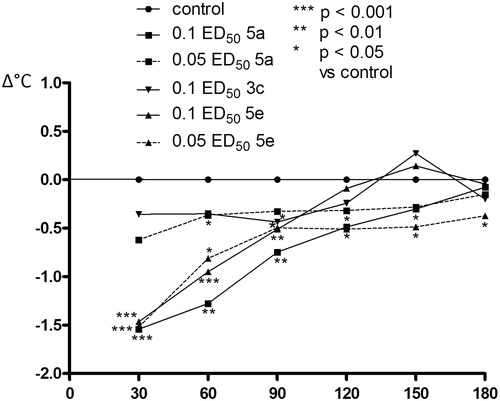
Series 4 and 6 (modification of series 3 and 5) did not show significant influence on the CNS. Only activity that showed the substance 6d from series 6, was weak antinociceptive activity in the writhing test (not associated with the opioid system, data not presented). From series 4, compound 4c affected only body temperature of mice. Two-way ANOVA revealed significant effects for both doses [F(2,146) = 45.70; p < 0.0001] and time [(F(5,146) = 3.18; p < 0.01], as well as a statically significant dose × time [F(10,146) = 3.02; p < 0.01]. Post-hoc Bonferroni test confirmed a significant decrease in the body temperature of mice after the administration of compound 4c at the dose of 0.1 ED50 from 120 to 150 min (p < 0.01). This compound used at twice less dose – had no effect ().
Figure 11. The influence of compound 4c (used at the doses of 0.1 and 0.05 ED50) on the body temperature of mice. Two-way ANOVA revealed significant effects for both doses [F(2,146) = 45.70; p < 0.0001] and time [(F(5,146) = 3.18; p < 0.01], as well as a statically significant dose x time [F(10,146) = 3.02; p < 0.01]. Post-hoc Bonferroni test confirmed a significant decrease in the body temperature of mice after the administration of compound 4c at the dose of 0.1 ED50 from 120 to 150 min (p < 0.01).
![Figure 11. The influence of compound 4c (used at the doses of 0.1 and 0.05 ED50) on the body temperature of mice. Two-way ANOVA revealed significant effects for both doses [F(2,146) = 45.70; p < 0.0001] and time [(F(5,146) = 3.18; p < 0.01], as well as a statically significant dose x time [F(10,146) = 3.02; p < 0.01]. Post-hoc Bonferroni test confirmed a significant decrease in the body temperature of mice after the administration of compound 4c at the dose of 0.1 ED50 from 120 to 150 min (p < 0.01).](/cms/asset/d83d6eda-7b38-42bf-a291-bf4566dbed95/ienz_a_965699_f0011_c.jpg)
The effect of the compounds from series 3 and 5 in other tests (rota-rod test, chimney test, PTZ-induced seizures)
All the investigated series of compounds caused no coordination impairments as measured in the rota-rod and chimney test. These results show the lack of neurotoxicity, and muscle relaxant potency of the substances, what is important because it can change the results of other tests (e.g. motility test) and affecting reliability of the tests resultsCitation31. Moreover, the tested substances failed to protect against clonic seizures, tonic convulsions and death in PTZ-induced model of seizures ().
In conclusion, pharmacological results obtained in this work, suggest a possible involvement of serotonin system in the effects of compounds series 3 and 5. The precise mechanism, however, remains elusive and future studies are needed.
Structure–activity relationship
Molecular structures of selected compounds from each series are presented in . It can be noticed that the imidazo[1,2-a][1,3,5]triazin system is planar as expected.
The lack of activity of compounds from series 4 and 6 may be primarily attributed to their unfavourable ADMET properties, i.e. low lipophilicity (related to hihgher polar surface area and smaller volume than for series 3 and 6, ) and poor blood–brain permeation. It is also illustrated in , showing ESP distribution for selected compounds. The HOMO and LUMO energies and polarizability do not seem to be directly related to activity ().
Figure 13. The map of the electrostatic potential (ESP) onto a surface of the electron density for 3b – A, 4c – B, 5e – C and 6d – D.
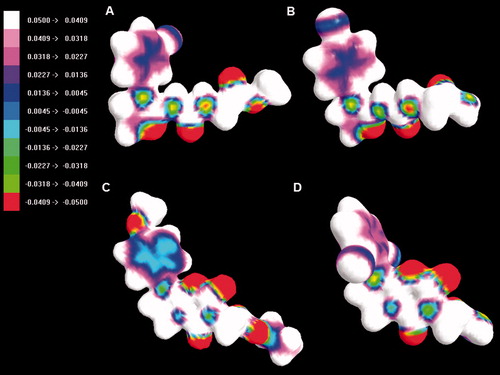
Table 4. Parameters for structure–activity relationship studies.
Conclusions
In conclusion, we have designed and studied 4 series of compounds and two of them exhibited antinociceptive properties of unknown mechanism accompanied by serotoninergic properties. Further studies are necessary to investigate the molecular target(s) for these compounds which will enable to apply structure-based design methods to obtain more favorable modifications of these compounds.
Acknowledgements
The article was developed using the equipment purchased within the project “The equipment of innovative laboratories doing research on new medicines used in the therapy of civilization and neoplastic diseases” within the Operational Program Development of Eastern Poland 2007–2013, Priority Axis I modern Economy, operations I.3 Innovation promotion.
Declaration of interest
The research was partially performed during the post-doctoral fellowship of Agnieszka A. Kaczor at University of Eastern Finland, Kuopio, Finland under Marie Curie fellowship. Part of the calculations was performed under a computational grant by Interdisciplinary Center for Mathematical and Computational Modelling (ICM), Warsaw, Poland, grant number G30-18 and under resources and licenses by CSC, Finland. The authors report no declarations of interest.
References
- Manjiani D, Paul DB, Kunnumpurath S, et al. Availability and utilization of opioids for pain management: global issues. Ochsner J 2014;14:208–15
- Rzadkowska M, Szacon E, Kaczor AA, et al. Synthesis, pharmacological activity and molecular modeling of 1-aryl-7-hydroxy-2,3-dihydroimidazo[1,2-a]pyrimidine-5(1H)-ones and their 6-substituted derivatives. Med Chem 2014;10:460–75
- Kaczor A, Matosiuk D. Non-peptide opioid receptor ligands – recent advances. Part I – agonists. Curr Med Chem 2002;9:1567–89
- Kaczor A, Matosiuk D. Non-peptide opioid receptor ligands – recent advances. Part II – antagonists. Curr Med Chem 2002;9:1591–603
- Huang P, Kim S, Loew G. Development of a common 3D pharmacophore for delta-opioid recognition from peptides and non-peptides using a novel computer program. J Comput Aided Mol Des 1997;11:21–8
- Mosberg HI, Omnaas JR, Lomize A, et al. Development of a model for the delta opioid receptor pharmacophore. 2. Conformationally restricted Phe3 replacements in the cyclic delta receptor selective tetrapeptide Tyr-c[D-Cys-Phe-D-Pen]OH (JOM-13). J Med Chem 1994;37:4384–91
- Korlipara VL, Takemori AE, Portoghese PS. Electrophilic N-benzylnaltrindoles as delta opioid receptor-selective antagonists. J Med Chem 1995;38:1337–43
- Matosiuk D, Fidecka S, Antkiewicz-Michaluk L, et al. Synthesis and pharmacological activity of new carbonyl derivatives of 1-aryl-2-iminoimidazolidine. Part 1. Synthesis and pharmacological activity of chain derivatives of 1-aryl-2-iminoimidazolidine containing urea moiety. Eur J Med Chem 2001;36:783–97
- Matosiuk D, Fidecka S, Antkiewicz-Michaluk L, et al. Synthesis and pharmacological activity of new carbonyl derivatives of 1-aryl-2-iminoimidazolidine. Part 3. Synthesis and pharmacological activity of 1-aryl-5,6(1H)dioxo-2,3-dihydroimidazo[1,2-a]imidazoles. Eur J Med Chem 2002;37:845–53
- Matosiuk D, Fidecka S, Antkiewicz-Michaluk L, et al. Synthesis and pharmacological activity of new carbonyl derivatives of 1-aryl-2-iminoimidazolidine: part 2. Synthesis and pharmacological activity of 1,6-diaryl-5,7(1H)dioxo-2,3-dihydroimidazo[1,2-a][1,3,5]triazines. Eur J Med Chem 2002;37:761–72
- Sztanke K, Fidecka S, Kedzierska E, et al. Antinociceptive activity of new imidazolidine carbonyl derivatives. Part 4. Synthesis and pharmacological activity of 8-aryl-3,4-dioxo-2H,8H-6,7-dihydroimidazo[2,1-c] [1,2,4]triazines. Eur J Med Chem 2005;40:127–34
- Rządkowska M, Szacoń E, Kaczor AA, et al. Synthesis, central nervous system activity, and structure–activity relationship of 1-aryl-6-benzyl-7-hydroxy-2,3-dihydroimidazo[1,2-a]pyrimidine-5(1H)-ones. Med Chem Res 2014;10:460–75
- Peckys D, Landwehrmeyer GB. Expression of mu, kappa, and delta opioid receptor messenger RNA in the human CNS: a 33P in situ hybridization study. Neuroscience 1999;88:1093–135
- Sun H-L, Zheng J-W, Wang K, et al. Tramadol reduces the 5-HTP-induced head-twitch response in mice via the activation of mu and kappa opioid receptors. Life Sci 2003;72:1221–30
- Fields HL, Basbaum AI. Central nervous system mechanisms of pain modulation. In: Wall PD, Melzack R, eds. Textbook of pain. Edinburgh, London: Churchill Livingstone; 1994:243–57
- Pradhan AA, Smith ML, Zyuzin J, Charles A. δ-Opioid receptor agonists inhibit migraine-related hyperalgesia, aversive state and cortical spreading depression in mice. Br J Pharmacol 2014;171:2375–84
- Szacon E, Rzadkowska M, Matosiuk D, et al. Polish Patent PL 208539/2011
- Rzadkowska M, Szacon E, Matosiuk D, et al. Polish patent PL 211768/2012
- Rzadkowska M, Szacon E, Matosiuk D, et al. Polish patent PL 211769/2012
- Szacoń E, Rzadkowska M, Matosiuk D, et al. Polish patent PL 2138806/2013
- Bekircan O, Küxük M, Kahveci B, Kolayli S. Convenient synthesis of fused heterocyclic 1,3,5-triazines from some N-acyl imidates and heterocyclic amines as anticancer and antioxidant agents. Arch Pharm (Weinheim) 2005;338:365–72
- Saczewski F, Maruszak M, Bednarski PJ. Synthesis and cytotoxic activity of imidazo[1,2-a]-1,3,5-triazine analogues of 6-mercaptopurine. Arch Pharm (Weinheim) 2008;341:121–5
- Soliman AM, Mohamed SK, El Remaily MAA, Abdel-Ghany H. Synthesis and biological activity of dihydroimidazole and 3,4-dihydrobenzo[4,5]imidazo[1,2-a][1,3,5]triazins. Eur J Med Chem 2012;47:138–42
- LigPrep version 2.4. New York: Schrödinger, LLC; 2010
- Epik version 2.1. New York: Schrödinger, LLC; 2010
- Pedretti A, Villa L, Vistoli G. VEGA - an open platform to develop chemo-bio-informatic applications, using plug-in architecture and script” programming. J Comput Aided Mol Des 2004;18:167–73
- Discovery Studio 3.1, Accelrys. Available from: http://accelrys.com [last accessed 15 Jun 2014]
- MOE Molecular Operating Environment (2009/2010), Chemical Computing Group. Available from: http://www.chemcomp.com/software.htm [last accessed 15 Jun 2014]
- Osiris Property Explorer. Available from: http://www.organic-chemistry.org/prog/peo/logS.html [last accessed 15 Jun 2014]
- Argus Lab, Available from: http://www.arguslab.com/arguslab.com/ArgusLab.html [last accessed 15 Jun 2014]
- Vogel GH, Vogel W. Drug discovery and evaluation. Pharmacological Assays. Berlin: Springer-Verlag, 1997
- Litchfield JT Jr, Wilcoxon F. A simplified method of evaluating dose-effect experiments. J Pharmacol Exp Ther 1949;96:99–113
- Koster R, Anderson M, DeBeer EJ. Acetic acid for analgesic screening. Fed Proc 1959;18:412–16
- Le Bars D, Gozariu M, Cadden SW. Animal models of nociception. Pharmacol Rev 2001;53:597–652
- Gross F, Tripod J, Meir R. Zur Pharmakologischen charakterisierung des Schlafmittels. Doriden Schweiz Med Wochschr 1955;85:305–9
- Boissier JR, Tardy J, Diverres JC. Une nouvelle méthode simple pour explorer l’action tranquilisante: le test de la cheminée. Med Exp (Basel) 1960;3:81–4
- Corne SJ, Pickering RW, Warner BT. A method for assessing the effects of drugs on the central actions of 5-hydroxytryptamine. Br J Pharmacol Chemother 1963;20:106–20
- Lee SK, Chang GS, Lee IH, et al. The PreADME: pc-based program for batchprediction of ADMEproperties, In: EuroQSAR 2004, September, 5–10, Istanbul, Turkey
- Pajouhesh H, Lenz GR. Medicinal chemical properties of successful central nervous system drugs. NeuroRx 2005;2:541–53
- Gutstein HB, Akil H. Opioid analgesics. In: Bruntn L, ed. Goodman and Gilman's. The pharmacological basis of therapeutics, 11th ed. New York: MacGrow Hill; 2006:547–90
- Cox B, Lomax P. Pharmacological studies on the central regulation of body temperature. Annu Rev Pharmacol Toxicol 1997;17:341–53
- Gao B, Jr Ducan WC, Wehr TA. Clorgyline-induced reduction in body temperature and its relationship to vigilance states in Syrian hamsters. Neuropsychopharmacology 1991;4:187–97
- Adell A, Bigg TA, Myers RD. Action of harman (1-methyl-β-carboline) on the brain: body temperature and in vivo efflux of 5-HT from hippocampus of the rat. Neuropharmacology 1996;35:1101–7