Abstract
Campylotropis hirtella is used as a food supplement in the subtropical region of China. In an intensive hunt for human neutrophil elastase inhibitors, we isolated eight flavonoids from C. hirtella three of which (1–3) emerged to be elastase inhibitors. Geranylated flavonoids (1–3) displayed significant inhibitory activity with IC50s between 8.5 and 30.8 μM. The most striking example was geranylated isofavanone 3 that inhibited elastase significantly (IC50 = 30.8 μM) but its parent compound (dalbergioidin) and isoflavone analog (5) were inactive (IC50 > 200 μM). Compounds (1–3) displayed different kinetic mechanisms (noncompetitive, competitive, and mixed type, respectively) that were dependent upon the parent skeleton. The competitive inhibitor, isoflavan-3-ol-4-one 2 manifested an inhibition of isomerization profile for elastase with kinetic parameters K5 = 0.0386 M−1S−1, K6 = 0.0244 μM−1S−1 and Kiapp = 16.3427 μM. The specific identification of metabolites was accomplished by LC-DAD-ESI/MS that was also used to analyze abundance of active components (1–3) within the plant.
Introduction
Elastin is the major component of elastic fibers which surround blood vessels, lung tissues, and ligaments. Human neutrophil elastase (HNE, EC 3.4.21.37) is a serine protease stored in large quantities in neutrophils. Numerous studies have reported that neutrophils are the main inflammatory mediators implicated in acute alveolar injury and are also essential for host defense against invading microorganismsCitation1,Citation2. HNE plays a critical role in the initiation and progression of pulmonary inflammationCitation3. The catalytic site of HNE is composed of the triad His 41-Asp 99-Ser 173, in which the serine oxygen becomes a powerful nucleophile and ultimately attacks the carbonyl of the substrate attached to the scissile bond. HNE may be activated upon exposure to various cytokines and chemoattractants including tumor necrosis factor (TIVF)-a, interleukin (IL)-8, C5a, and LPS. The activity of HNE is controlled by an inhibitor termed α1-antitrypsin, produced in the liver. The imbalance between HNE and its inhibitors is implicated in many inflammation diseases, such as rheumatoid arthritis, respiratory distress syndrome, pulmonary emphysema, etcCitation4,Citation5. Thus, neutrophil elastase inhibitors have been actively sought after by the medicinal chemistry community. Most works have focused on the development of synthetic elastase inhibitors that have high potency (IC50 in the nanomolar range), show selectivity relative to a panel of other proteases and have good metabolic stability. For instance, the BAY 85-8501 is one of best candidate inhibitors for neutrophil elastaseCitation6,Citation7. However, there are still relatively few studies disclosing natural products that target this important enzymeCitation8,Citation9. Moreover studies that have previously investigated HNE inhibition by secondary metabolites do not include detailed kinetic characterization.
Campylotropis hirtella belongs to the Leguminosae family and has been cultivated as a food ingredient in subtropical parts of China such as the Yunna, Sichuan, and Guizhou provinceCitation10. Its roots have been used to treat a variety of maladies including dysmenorrhea, metrorrhagia, metrostaxis, gastric ulcers, as well as benign prostate hyperplasia in ChinaCitation11. C. hirtella is known to contain a variety of secondary metabolites including lignans, sesquilignans, diligana, coumarin, and geranylated flavonoidsCitation12–14. It has previously been shown that some compounds within C. hirtella have inhibitory activity against prostate specific antigen secretion in LIVCaP cellsCitation15. Geranylated flavnonids from the roots of this plant also exhibit immunosuppressive activityCitation16. The aim of the present work is to investigate the HNE inhibitory activities of metabolites within the roots of C. hirtella. We isolated eight polypehnols from the ethyl acetate extract of C. hirtella. All isolated compounds were examined for their HNE inhibitory activities and three of these compounds emerged to inhibit HNE. These three compounds showed different kinetic behaviors dependent upon their molecular structure. We also assessed the relative abundance of these extracts within the native root using an HPLC-ESI/MS chromatogram profile.
Materials and methods
Chemical and instruments
1D and 2D NMR spectra were recorded on a Bruker (a.m. 500 MHz) spectrometer (Billerica, MA), using Acetone-d6, as solvent and tetramethylsilane (TMS) as an internal standard. Electron ionization (EI) and EI high resolution (HR) mass spectra were obtained on a JEOL JMS-700 instrument (JEOL, Tokyo, Japan). Optical rotations were measured on a Perkin-Elmer 343 polarimeter (Perkin-Elmer, Bridgeport Ave, Shelton, CT). Column chromatography was performed using silica gel (230–400 mesh; Merck Co., Darmstadt, Germany), YMC-gel ODS-A (S-75 μm; YMC), and Sephadex LH-20 (GE GEalthcare Bio-Science AB, Uppsala, Sweden). Thin-layer chromatography (TLC) was performed on precoated silica gel 60 F254 (0.25 mm; Merck). Spots were detected by UV light (245 nm) and spaying with 10% H2SO4 followed by heating. Enzymatic assays were carried out on a SpectraMaxM3Multi-Mode Microplate Reader (Molecular Devise, Sunnyvale, CA). Melting points were measured on a Thomas Scientific capillary apparatus (Swedesboro, NJ). UV spectra were measured on a Beckman DU650 spectrophotometer (Indianapolis, IN). Enzymatic assay was carried out on a SpectraMaxM3Multi-Mode Microplate Reader (Molecular Devices). Dalbergioidin and oleanolic acid were purchased from Sigma-Aldrich (St. Louis, MO). All other chemicals were of analytical grade and used as received. C. hertella (approximately 2 kg) was purchase from a medicinal market in China.
Extraction and isolation of C. hertella
The dried and ground root of C. hirtella (1 kg) was extracted three times with MeOH (10 L) at room temperature over a week. The methanol-soluble portion was concentrated to give the crude extract (68 g), which was partitioned between H2O and n-hexane. The aqueous layer was further partitioned with EtOAc to give the EtOAc extract (32 g) that showed potent inhibition against HNE. This fraction (30 g) was subjected to flash column chromatography (15 × 30 cm, silica gel 230–400 mesh) eluting with gradient of n-Hexane-EtOAc (150:1 to 0:1) to afford eight subtractions (CH1–CH9). Fraction CH3 was chromatographed on a silica gel column using a stepwise gradient of n-hexane:acetone mixture as a solvent system (30:1 to 10:1) to yield compound 1 (27 mg) and five subfractions (SH3.1–CH3.5). Subfraction CH3.4 was further purified by reverse-phase chromatography using MeOH:H2O (9:1) as a mobile phase to yield compound 3 (23 mg).
Fraction CH5 was chromatographed on a reversed-phase column using RP-C18 eluting with a stepwise gradient of ACN:H2O mixture (1:10 to 1:0) to afford three subfractions (CH5.1–CH5.3). CH5.1 was chromatographed on silica gel eluting with a gradient of CHCl3:MeOH) (10:1 to 0:1). The eluates were then purified with Sephadex LH-20 column using 95% MeOH as a mobile phase to yield compound 2 (47 mg). The purity of the isolated compounds ranged from 95.0% to 99.5% as assessed by analytical HPLC (Agilent 1100 HPLC system; a Zorbax Bonus-RP-C18 column [4.6 × 150 mm, 5 μm]: H2O/ACN (60:40); UV detection, 254, 280, 310, 380 nm; flow rate, 1.0 ml/min).
Preparation of sample solutions
The roots of C. hertella were pulverized into powder. The sample (1.0 g) was extracted in 20 ml of methanol for 6 h at room temperature with shaking. The supernatant was centrifuged at 3000 g for 5 min and then filtered through a 0.45 mm syringed filter prior to LC-ESI-MS analysis.
Liquid-chromatography electrospray-ionization mass spectrometry (LC-ESI-MS)
HPLC analysis was carried out on a 1100 series liquid chromatography (LC) system, equipped with a G1322A degasser, G1312A pump, G1313A auto sampler, and G1316A oven (Agilent Technologies, Palo Alto, CA). The chromatographic separation was performed on a Zorbax Bonus-RP-C18 column (4.6 × 150 mm, 5 μm, Agilent Technologies, Rising Sun, MD). The mobile phase (A) was acetic acid/water (0.1/100, v/v), and the mobile phase (B) was acetic acid/ACN (0.1/100, v/v). The linear gradient solvent system was as follows: 0–40 min, 15–70% B; 40–60 min, 100% B; 60–75 min, 100% B. The flow rate was 0.5 ml/min, with a column temperature of 27 °C and an injection volume of 10 μl in all experiments. UV spectra were recorded over a range of 200–400 nm and chromatograms were acquired at 280 nm. The LC elution was introduced directly into the ESI interface without splitting.
Mass spectrometry was performed using a 3200 Q TRAP LC/MS/MS system (Applied Biosystems, Foster City, CA) equipped with a Turbo VTM source and a Turbo Ion Spray probe (450 °C). The mass spectrometer was operated in the positive and negative ion mode. Bio-AnalystTM, version 1.4.2, and Analyst software, version 1.4.2, were used for instrument control and data acquisition, respectively. Nitrogen was used as both nebulizing and drying gas. The flow rate in both instances was 60 psi. The capillary voltage was set at 5.5 kV/−4.5 kV and the source temperature at 450 °C. The resolutions of quadrupole were 0.8–1.0 (low resolution), respectively. Mass spectra were recorded over the scan range; m/z 100–1000 by a step size of 0.1 amu.
Measurement of human neutrophil elastase activity
HNE inhibitory activity was evaluated according to a previously described procedureCitation17. The hydrolytic activity of HNE (Sigma-Aldrich) was measured using methoxysuccinyl-Ala-Ala-Pro-Val-p-nitroanilide (Sigma-Aldrich) as substrate in clear 96-well microliter plates. All samples were dissolved in 100% dimethyl sulfoxide (DMSO) at 200 μM stock concentrations and used for the experiment with dilution. The mixture contained 130 μl 0.02 mM Tris-HCl buffer solutions (pH 8.0) and 20 μl enzymes (0.01 Unit/ml) were preincubated for 5 min in the presence of test compounds or vehicle (DMSO). The final concentration of DMSO was 5% throughout. The reaction was initiated by the addition of 40 μl substrate. The formation of p-nitroanilide (pNA) was monitored by detection at 405 nm for 5 min. Using a substrate concentration range from 0.1 mM to 6 mM Km was calculated to be 1.5 ± 0.3 mM and Vmax was 3.9 ± 0.4 mM s−1 for the commercial enzyme preparation. IC50s were calculated using non-linear regression fitting to Equation (1) as followsCitation18:
(1)
Progress curves determination and time-dependent assay
For further investigation of the inhibition mechanism, we measured initial velocities of substrate hydrolysis as a function of preincubation time of HNE with inhibitor, probing the time-dependence of inhibition of the hydrolysis of substrate by these inhibitors. The data were analyzed using the a nonlinear regression program (Sigma Plot (SPCC Inc., Chicago, IL)) to give the individual parameters for each curve; vi (initial velocity), vs (steady-state velocity), kobs (apparent first-order rate constant for the transition from vi to vs), A (absorbance at 410 nm), and (apparent Ki) according to the following equationsCitation19–21:
(2)
(3)
(4)
Statistical analysis
All the measurements were made in triplicated. The results were subject to variance analysis using Sigma Plot (Version 12.0, Systat Software, Inc., San Jose, CA). Differences were considered significant at p < 0.05.
Results and discussion
Characterization of human neutrophil elastase inhibitory compounds
In preliminary experiments, the methanol extract of the roots of C. hirtella exhibited good potential for enzymatic inhibitory activities against HNE. In further activity-guided fractionation, ethyl acetate fraction (100 μg/ml, 80% inhibition) was found to be the most effective fraction for HNE inhibition and it encourage us to identify the compounds responsible for its HNE inhibition. Ethyl acetate fraction mainly consisted of chromophoric polyphenols that have absorbance at 280 nm (. Purification of EtOAc extract over silica gel, octadecyl-functionalized silica gel Sephadex LH-20 gave eight flavonoids (1–8). The compounds were identified as (2R, 3R)-6-methyl-3′-geranyl-2,3-trans-5,7,4′-trihydroxy-flavonol (1), (E)-3-(3-(3,7-dimethylocta-2,6-dienyl)-2,4-dihydroxyphenyl)-3,5,7-trihydroxy-chroman-4-one (2), 3′-geranyl-5,7,2′,4′-tetrahydroxyisoflavanone (3), 3(S)-2′,4′-dihydroxy-5,5′-dimethoxy-(6″,6″-dimethylpyano)-(2″,3″:7,6)-isoflavan (4), 3′-geranyl-5,7,2′,5′-tetrahy-droxyisoflavone (5), myrsininone A (6), isosophoranone (7), and myrsininone B (8) through analysis of spectroscopic data and by comparison with previous studiesCitation22–Citation24. The exact chemical structures were fully determined from spectroscopic data including 2D-NMR ( and Supplementary material). For example, the most effective compound (2) was obtained as a pale yellow solid having the molecular formula C25H28O7 and 12 degrees of unsaturation established by HREIMS (m/z 440.1838 [M]+). Eight C-C double bonds and one carbonyl group were identified, accounting for 9 of 12 degrees of unsaturation. The extra three degrees of unsaturation were ascribed to an isoflavanone function. The HMBC correlation of the 1H NMR peak (H–1″; δH 3.45, J = 5.76 Hz) with C-3′ (δC 116.6) allowed us to assign the location of the geranyl group. The position of C-3-OH was confirmed because C-3 had a characteristically low chemical shift (δC 95) and doublet germinal coupling of H-2 (δH 10.31 and 11.24). Thus, compound 2 was identified as (E)-3-(3-(3,7-dimethylocta-2,6-dienyl)-2,4-dihydroxyphenyl)-3,5,7-trihydroxychroman-4-one (2).
Human neutrophil esterase inhibitory activity
HNE is controlled by its endogenous inhibitors, like α1-antitrysin, but the affinity of these endogenous inhibitors for HNE is strongly decreased by oxidative stress and by proteases released upon inflammation. Extra activity of HNE is considered to cause tissue destruction associated with inflammation and microorganism infection. The aim of this study is to develop lead structures for HNE inhibition from C. hirtella. The enzyme was assayed according to standard literature by following the hydrolysis of MeOSuc-AAPV-pNA, spectrophotometrically. The isolated flavonoids (1–8) and parent compound, dalbergioidin were screened for their in vitro HNE inhibitory activities at different concentrations. Compounds 1–3 exhibited significant and a dose-dependent inhibitory effect against HNE activity with IC50s ranging 8.5–30.8 μM ( and ). These inhibitor potencies are relatively weak compared to known drug candidates, such as BAY 85-8501. However, they are potent among inhibitors isolated from plant extracts. Because most inhibitors from plants inhibited HNE with IC50 values ranging from 20 μM to millimolar level, although one of best inhibitor, quercetin, showed 6.0 μM of IC50Citation9,Citation25,Citation26. The activity was affected by subtle changes in structure as shown . It is apparent that C-2 hydroxyl group in dihydroflavanol is pivotal for inhibition because dihydroflavanol 1 is relatively potent (IC50 = 17.9 μM) whereas flavanone 8, that lacks the hydroxyl function is not (IC50 > 200 μM). This observation is in agreement with other flavonol-derived NE inhibitors in the literature which have a hydroxyl group on C-3 position. The parallel comparison between isoflavanone 3 (IC50 = 30.8 μM) and its isoflavone analog 5 (IC50 > 200 μM) established that the latter is not a good scaffold for HNE inhibition. Most importantly, geranylation was confirmed to be a key requisite for inhibitory activity by comparison of isoflavanone 3 (IC50 = 30.8 μM) and its parent compound, dalbergioidin (IC50 > 200 μM). The most effective inhibitor was found to be isoflavanone 2 (IC50 = 8.5 μM) that is unique in having a C-2 hydroxyl group.
Figure 2. (A) Effect of compounds on the HNE-catalyzed hydrolysis of methoxysuccinyl-Ala-Ala-Pro-Val-p-nitroanilide (compound 1, •; compound 2, ▾; compound 3, ○; dalbergioidin, △, respectively.) (B–D) Lineweaver–Burk plot for inhibition of the HNE-catalyzed hydrolysis of substrate by compounds (1–3).
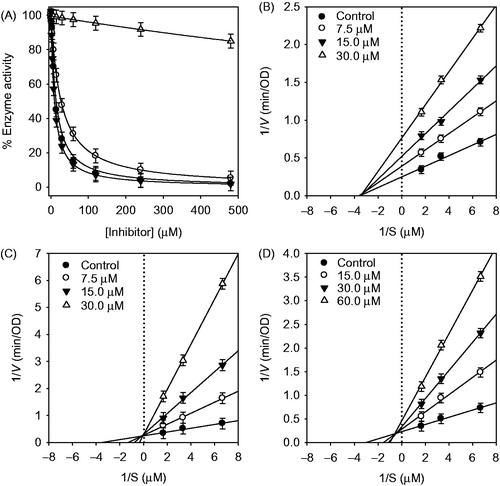
Table 1. Inhibitory effect of isolated compounds 1–8 on human leukocyte elastase.
Table 2. Peak assignments, mass spectral data, and cited references of flavonoids in the root bark of C. hirtella from the positive and negative mode using HPLC-DAD-ESI/MS.
LC-DAD-ESI/MS characterization of C. hirtella
To set the importance of inhibitors in a practical context, we performed a comparative analysis of the levels of individual NE inhibitory flavonoids within the native roots of C. hirtella using HPLC analysis. The nature of each peak in the HPLC trace was doubly verified by comparison with retention time of the pure compound and also HPLC-DAD-ESI/MS (). The mass analysis of peak 1 (tR = 43.69 min) presented the molecular ion [M + H]+ at m/z 439 and a fragment ion at m/z 315, which was formed by the loss of a geranyl group. The mass scan analysis of peak 2 (tR = 40.05 min) showed α [M–H]− ion at m/z 439 and fragment ion at m/z 421 that resulted from loss of H2O, which is unique feature of 2-hydroxy isoflavone. The product ion mass spectrum of peak 3 (tR = 42.59 min) showed [M + H]+ at 425 and the major fragment at 301 from loss of the geranyl group. Moreover, the most active HNE inhibitors (1–3) were present in high concentrations in the plant because they appeared as principal peaks in the chromatogram such as at 43.69 min (1), 40.05 min (2), and 42.59 min (3), respectively. shows UV-Vis absorption maxima, retention times, mass spectral data, including molecular and fragment ions of the isolated flavonoids in comparison with previously published data.
Determination of the inhibition type of flavonoids on human neutrophil elastase
Having established efficacy and abundance of these inhibitors, we next studied the kinetic mechanism of the interaction of three potent inhibitors (1–3) with HNE. The enzymatic activity was measured over a series of substrate concentrations and at various inhibitor concentrations. The inhibition data were globally fit to all of the possible kinetic inhibition models which gave identical results to the Lineweaver–Burk plots described below. Increasing the concentration of the inhibitors (1–3) drastically lowered residual enzyme activity (). We then further characterized the inhibitory mechanism of inhibitor (1–3) using both Lineweaver–Burk and Dixon plots (). Compound 1 displayed noncompetitive inhibition, because increasing substrate concentrations resulted in a family of lines with different slopes, but a common x-axis intercept. This noncompetitive behavior was also confirmed because Km remained constant with increasing concentration of inhibitor 1, whereas Vmax decreased. As shown in , the kinetic plots show that compound 3 is a mixed inhibitor. This is because increasing the concentration of 3 resulted in a family of lines with a common intercept on the left of the vertical axis and above the horizontal axis. The kinetic plot in ) showed that compound 2 is a competitive inhibitor. This is because increasing concentrations of 2 resulted in a family of lines with a common intercept on the 1/v axis but with different gradients. The plots of the remaining enzyme activity versus the concentrations of enzyme at different inhibitor concentrations gave a family of straight lines, which all passed through the origin (). This indicates that compound 2 is a reversible inhibitor.
Figure 3. (A) Typical HPLC-DAD chromatogram of the methanol extracts of C. hirtella detected at 280 nm. (B) Mass fragmentation patterns of identified peaks that accord with compound number. (a) compound 1; (b) compound 2; (c) compound 3; (d) compound 4; (e) compound 5; (f) compound 6; (g) compound 7; (h) compound 8.
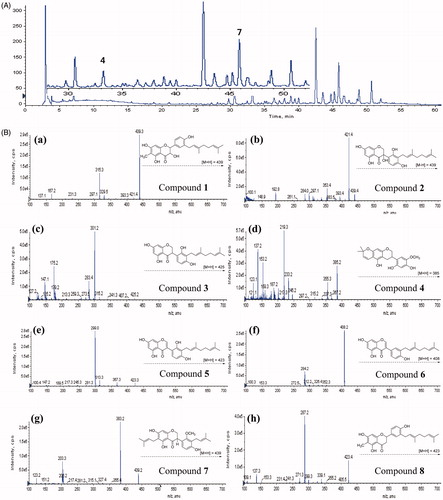
Figure 4. Inhibition of HNE by compound 2. (A) The hydrolytic activity of HNE as function of enzyme concentration at different concentrations of compound 2. (B) Time-dependent inhibition of HNE in the presence of compound 2 with varying pre-incubation times. (C) Pre-incubation time dependence of the fractional velocity of the enzyme-catalyzed reaction in the presence of varying concentration of compound 2. (D) Dependence of kobs on the concentration of compound 2. The kobs values determined in (C) were fitted to Equation (3).
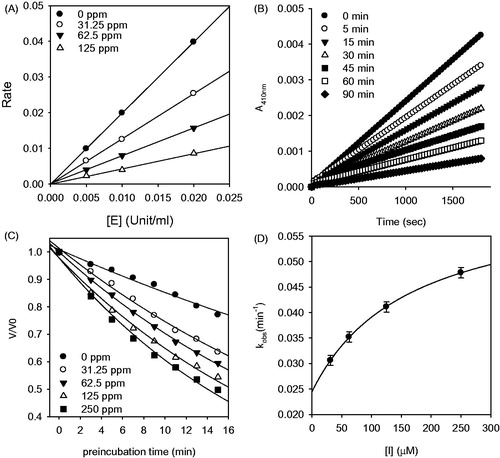
To further investigate the inhibition mechanism, we investigated time dependence of inhibition of HNE by compound 2. Reaction progress curves in the presence of varying concentrations of inhibitor are illustrated (). Compound 2 showed a clear time- and concentration-dependent approach to the steady-state, which is characteristic of slow-binding inhibition kinetics () Slow-binding inhibition mechanisms can be investigated by preincubation of the enzyme with the inhibitor, followed by measurement of the residual velocities for substrate hydrolysis as a function of preincubation time. Increasing the concentration of compound 2 led to a decrease in both the initial velocity (vi) and the steady-state rate (vs). The progress curves obtained using various concentrations of the inhibitors were fit to (EquationEquation 2(2) ) to determine vi, vs, and kobs. The plot represents the relationship between kobs and [I] for all possible inhibition modes (simple reversible slow-binding, enzyme isomerization, and mechanism-based inhibition) to determine which kinetic profile was at play. The kinetic parameter (k3, k4, k5, k6, and Kiapp) were derived from the plots by fitting the data (Supplementary material). The data for inhibitor 2 were fit to the slow-binding (EquationEquation 3
(3) ), illustrating a hyperbolic dependence of kobs on the concentration of HNE. Based on the above kinetic observations, we believe that the reaction undergoes the following kinetic mechanism (). The hyperbolic dependence of kobs versus [I] suggests that compound 2 inhibits HNE by rapid formation of an enzyme complex (E·I), which slowly isomerized to from a modified enzyme complex (E*I) (). This is expected to offer sustained inhibition because the reverse rate from the final inhibited complex is slow (k6 = 0.0244 mM−1s−1).
Figure 5. Scheme for time-dependent enzyme inhibition. The upper part (k1, k2, kcat) denotes turnover of the enzyme in the absence of inhibition and were calculated using traditional methods as delineated in Materials and methods section. The lower part illustrates the mechanism for a slow-binding inhibition. In simple reversible slow-binding inhibition process of the values of k3 and k4 are slow relative to enzyme turnover and k5/k6 does not occur. In enzyme isomerization, an initial binding of the inhibitor to the enzyme leads to formation of the EI complex, which undergoes an isomerization to form the new complex E*I.
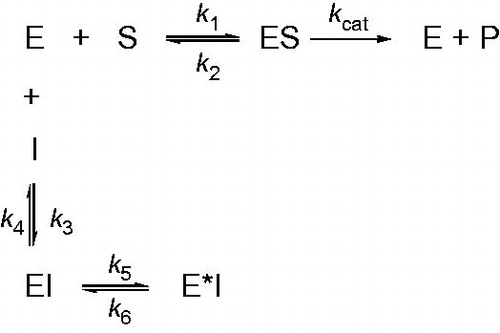
Conclusion
We found that C. hirtella has good potential against HNE. The principal inhibitory compounds in the target plant and their kinetics were fully characterized. The inhibition mechanism was dependent on the compound structure, with each active class dihydroflavanone (1), isoflavan-3-ol-4-one (2), and isoflavanone (3) exhibiting a different inhibition mechanism. The most potent compound 2 displayed low micromolar affinity for HNE, which makes it one of the most potent naturally derived HNE inhibitors. Importantly, this has a relatively long residence time on its target which may improve its efficacy relative to similarly potent, rapidly reversible compounds. We also proved that these bioactive components are abundant within the plant. Thus, C. hirtella can be considered to be an excellent source of natural HNE inhibitors for food and medicinal uses.
Declaration of interest
The authors declared that there are not conflicts of interest. The research was supported by a grant Next-Generation BioGreen 21 program (SSAC, NO. PJ01107001), Rural development Administration, Republic of Korea. All students were supported by a scholarship from the BK21 plus program.
Supplementary material available online
IENZ_1118683_Supplementary_material.pdf
Download PDF (885.9 KB)References
- Pham CTN. Neutrophil serine proteases fine-tune the inflammatory response. Int J Biochem Cell Biol 2008;40:1317–33.
- Griffin KL, Fischer BM, Kummarapurugu AB, et al. 2-O, 3-O-desulfated heparin inhibits neutrophil elastase-induced HMGB-1 secretion and airway inflammation. Am J Respir Cell Mol Biol 2014;50:684–9.
- Henriksen PA. The potential of neutrophil elastase inhibitors as anti-inflammatory therapies. Curr Opin Hematol 2014;21:23–8.
- McPhail LC, Strum SL, Leone PA, Sozzani S. The neutrophil respiratory burst mechanism. Immunol Ser 1992;57:46–76.
- Lee WL, Downey GP. Leukocyte elastase: physiological functions and role in acute lung injury. Am J Respir Crit Care Med 2001;164:896–904.
- von Nussbaum F, Li VM, Allerheiligen S, et al. Freezing the bioactive confromation to boost potency: the identification of BAY 85-8501, a selective and potent inhibitor of human neutrophil elastase for pulmonary disease. ChemMedChem 2015;10:1163–73.
- von Nussbaum F, Li VM. Neutrophil elastase inhibitors for the treatment of (cardio)pulmonary diseases: into clinical testing with pre-adaptive pharmacophores. Bioorg Med Chem Lett 2015;25:4370–81.
- Wittenauer J, Mäckle S, Sußmann D, et al. Inhibitory effects of polyphenols from grape pomace extract on collagenase and elastase activity. Fitoterapia 2015;101:179–87.
- Brás NF, Gonçalves R, Mateus N, et al. Inhibition of pancreatic elastase by polyphenolic compounds. J Agric Food Chem 2010;58:10668–76.
- Wen P, Han H, Wang R, et al. Cglycosylfavones and aromatic glycosides from Camplyotropis hirtella (Franch.) Schindl. Asian J Tradit Med 2007;2:149–53.
- Pharmacopoeia Committee of the People’s Republic of China Edita. Pharmcopoeia of the People’s Republic of China. Beijing: People’s Hygiene Press; 1997:30–1
- Shou QY, Fu RZ, Tan Q, Shen ZW. Geranylated flavonoids from the roots of Campylotropis hirtella and their immunosuppressive activities. J Agric Food Chem 2009;57:6712–19.
- Li XP, Xuan BX, Shou QY, Shen ZW. New flavonoids from Campylotropis hirtella with immunosuppressive activity. Fitoterapia 2014;95:220–8.
- Han HY, Wang XH, Wang NL, et al. Lignans isolated from Campylotropis hirtella (Franch.) Schindl. decreased prostate specific antigen and androgen receptor expression in LNCaP cell. J Agric Food Chem 2008;56:6928–35.
- Han HY, Wen P, Liu HW, et al. Coumarins from Campylotropis hirtella (Franch.) Schindl. and their inhibitory activity on prostate specific antigen secreted from LNCaP cells. Chem Pharm Bull 2008;56:1338–1.
- Shou QY, Tan Q, Shen ZW. Hirtellanines A and B, a pair of isomeric isoflavonoid derivatives from Campylotropis hirtella and their immunosuppressive activities. Bioorg Med Chem Lett 2009;19:3389–91.
- Benedek B, Kopp B, Melzig MF. Achillea millefolium L. s.l. – is the anti-inflammatory activity mediated by protease inhibition? J Ethnopharmacol 2007;113:312–17.
- Copeland RA. Enzyme: a practical introduction to structure, mechanism, and data analysis. New York: Wiley-VCH; 2000.
- Frieden C. Kinetic aspects of regulation of metabolic processes. The hysteretic enzyme concept. J Biol Chem 1970;245:5578–99.
- Morrison JF, Walsh CT. The behavior and significance of slow-binding enzyme inhibitors. Adv Enzymol Relat Areas Mol Biol 1988;61:201–301.
- Sculley MJ, Morrison JF, Cleand WW. Slow-binding inhibition: the general case. Biochim Biophys Acta 1996;1298:78–86.
- Shou QY, Tan Q, Shen Z. Isoflavonoids from the roots of Campylotropis hirtella. Planta Med 2010;76:803–8.
- Kang L, Zhou JX, Shen ZW. Two novel antibacterial flavnonids from Myrsine Africana L. Chin J Chem 2007;25:1323–5.
- Monache GD, Monache FD, Marini-Bettolo GB. Flavonoids of Sophora tomentosa (Leguminosae). II. Isosophoranone, a new diprenylated isoflavanone. Gazz Chim Ital 1997;107:189–93.
- Vasänge M, Liu B, Welch CJ, et al. The flavonoid constituents of two Polypodium species (Calaguala) and their effect on the elastase release in human neutrophils. Planta Med 1997;63:511–17.
- Kanashiro A, Souza JG, Kabeya LM, et al. Elastase release by stimulated neutrophils inhibited by flavonoids: importance of the catechol group. Z Naturforsch C 2007;62:357–61.