ABSTRACT
Pursed-lips breathing (PLB) is often spontaneously performed by chronic obstructive pulmonary disease (COPD) patients. The aim of this study was to evaluate spontaneous PLB prevalence and to identify factors discriminating its use. Fifty-seven patients with COPD (FEV1 = 44.3 ± 17.4%pred) underwent pulmonary function testing and two incremental bicycle exercise tests. Peak workload (Wpeak), oxygen uptake (VO2peak), breathing pattern, and dyspnea (Borg scale) were measured in the first exercise test and spontaneous PLB performance in the second. Six patients spontaneously performed pursed-lips breathing during rest (PLBrest), exercise and recovery, 18 during exercise and recovery (PLBex), 7 during recovery only (PLBrec), 20 not at all (PLBno), and 6 performed other expiratory resistive maneuvers. PLBrest and PLBex patients exhibited a lower Wpeak, O2 uptake, and minute ventilation (VE), greater expiratory flow limitation and higher slopes relating dyspnea to VE or W (%predicted). PLBrest patients were more hypercapnic, had a lower exercise tolerance and diffusion capacity, and greater flow limitation and hyperinflation. PLBrec and PLBno patients were indistinguishable with regard to pulmonary function, dyspnea, and exercise performance. The most significant independent predictors of spontaneous PLB use during exercise were FEV1/FVC and the slope relating dyspnea to VE. Spontaneous PLB is most often performed by COPD subjects when ventilation is stimulated by exercise, and during recovery from exercise. Severity of airflow obstruction and the dyspnea experienced during exercise play an important role in determining whether or not PLB is spontaneously performed by COPD patients.
INTRODUCTION
Pursed-lips breathing (PLB) is a ventilatory maneuver whereby expiration is performed through constricted lips. Numerous studies have reported that patients with chronic obstructive pulmonary disease (COPD) often spontaneously perform PLB (Citation1–3), with some patients claiming to use it at rest and others only during or following exercise (Citation2). Given that PLB appears to help alleviate dyspnea, it has been suggested that such patients may adopt PLB when they are especially “short of breath” (Citation1, Citation3). However, the breathing maneuver does not appear to provide consistent symptom benefit in all COPD patients (Citation2, Citation4, Citation5). PLB has been proposed to reduce dyspnea by moving the expiratory pressure drop from the lungs forward to the lips, thereby decreasing airway collapse (Citation4). Studies have also shown that PLB performed at rest promotes a slower and deeper breathing pattern (Citation1, 2, Citation6) and improves PaO2 (Citation2) and arterial oxygen saturation (SaO2) (Citation6, 7). Still, others have reported the reduction in dyspnea occurring with PLB to be related to a decreased chest wall end-expiratory volume and a lower baseline forced expiratory volume in 1 sec (FEV1) (Citation8). Although patients may perform PLB in response to some level of dyspnea that is experienced, factors such as the severity of airflow limitation, functional impairment of the respiratory muscles, impaired gas exchange, and/or altered breathing pattern may also act to trigger the use of PLB in patients with COPD.
The purpose of this study was to determine the prevalence of PLB at rest, during bicycle exercise and during recovery from exercise in a group of patients with COPD. The second aim was to determine whether patients who spontaneously perform PLB could be distinguished from those who do not, with respect to their anthropometric characteristics, baseline pulmonary function, blood gases, breathing pattern adopted at rest and during exercise, exertional dyspnea, and/or variables associated with exercise performance.
MATERIALS AND METHODS
Population
A cross-sectional study was carried out in a convenience sample of patients with stable COPD recruited prospectively from the pulmonary outpatients clinics of a tertiary hospital (Notre-Dame Hospital, Montreal, QC, Canada). All patients were less than 80 years of age and had a post-bronchodilator forced expiratory volume in 1 second (FEV1)/forced vital capacity (FVC) <0.7. Stability was operationally defined as unchanged respiratory symptoms, no change in medications used, and no exacerbation in the previous 4 weeks. Subjects with known cardiovascular disease, neurologic or psychiatric illness, as well as neoplastic disease were excluded from the study. Likewise excluded were subjects with impaired lower extremity function and lip or oral deformities. The study was approved by the hospital's ethics committee and all subjects gave their written informed consent. To avoid bias, subjects remained naive to the fact that we were assessing the performance of PLB.
Experimental protocol and instrumentation
The study comprised of two separate experimental sessions. Patients were asked to abstain from smoking on the days of the study and to avoid eating for at least 2 hours prior to undergoing testing.
During the first session, subjects underwent pulmonary function testing (System 1085; Medical Graphics Corp, MN, USA) according to American Thoracic Society standards (Citation9). Lung volumes and spiromtery were determined using a whole-body plethysmograph (System 1085; Medical Graphics Corp, MN, USA). Maximum inspiratory and expiratory mouth pressures (PImax and PEmax) were measured at FRC and TLC, respectively (Medical Graphics Corp., St. Paul, MN, USA). Spirometry, lung volumes maximum pressures, and single-breath diffusion capacity for carbon monoxide (DLCO) were compared with reported norms (Citation10–13). Maximum voluntary ventilation (MVV) was estimated by multiplying FEV1 by 40.
During the first session, subjects also underwent a symptom-limited incremental exercise test on a bicycle ergometer (Model 400L; Medical Fitness Equipment; Maarn, the Netherlands). Subjects initially breathed at rest for 6 minutes while seated on the bicycle. This was followed by exercise whereby work rate was increased by 15 watts every 2 minutes and the pedaling rate maintained at 60 ± 5 revolutions per minute. Following exercise, patients were allowed a recovery period of at least 6 minutes while seated on the bicycle. During this test, subjects breathed through a mouthpiece with noseclips on and the mouthpiece was connected to a calibrated metabolic cart (CPX Medical Graphics Corp., Minneapolis, MN, USA). Oxygen consumption (VO2), carbon dioxide production (VCO2), minute ventilation (VE), tidal volume (VT), breathing frequency (fB), heart rate (HR), and transcutaneous SaO2 (Novometrix Pulse Oximeter 151A, Medical Systems Inc) were continuously measured during the last 3 minutes with subjects breathing at rest, during incremental exercise to Wpeak, and during recovery following exercise. All variables were averaged on-line at 30-second intervals and stored on the computer for a later analysis. During each minute of exercise, subjects were asked to estimate their breathlessness using a modified Borg scale. After completing the test, subjects were required to describe the symptoms that caused them to stop exercising.
The maximum exercise work rate (Wpeak) was defined as highest workload subjects could maintain for 1 minute. Wpeak was expressed relative to that predicted using the equations of Jones et al. (Citation14). The predicted normal values used for maximum exercise VO2 were those of Jones and Campbell (Citation15). During each minute of exercise, subjects were asked to estimate their breathlessness using a modified Borg scale. After completing the test, subjects were asked to describe the symptoms that caused them to stop exercising. They were also asked to grade their dyspnea using the Modified Medical Research Council Dyspnea Scale (MRC) (Citation16) and Oxygen Cost Diagram (OCD) (Citation17).
Second exercise test (PLB prevalence)
Spontaneously performed PLB prevalence was determined during the second experimental session (within 48 hours of the first session) with subjects repeating the exercise protocol, this time without use of a mouthpiece or facemask. During the entire protocol, a video camera was used to record the configuration of each subject's mouth, and nasal pressure (Pno) was measured using a nasal cannula positioned within the nostrils and connected to a differential pressure transducer. The Pno signal was acquired on-line onto a desktop computer at a sampling rate of 100 Hz. The determination of PLB performance was based on observations of an expiratory “pursed-lip” mouth configuration on video camera recordings made by two independent observers, and a concomitant Pno drop to 0 cm H2O during the expiratory phase of such breaths (). Obliteration of nasal flow during expiration has been shown to occur when the nasopharynx is occluded by the upward movement of the soft palate during PLB (Citation18). The observation of PLB performed for at least five consecutive breaths was required to categorize its occurrence during the period of resting breathing preceding exercise, during exercise and/or recovery.
Statistical analysis
The PLB groups were defined according to when PLB was spontaneously performed. Group comparisons of parametric data from the first exercise session were made using one-way analysis of variance (ANOVA) and the Tukey test for post hoc comparisons. Comparisons of the MRC dyspnea scores were performed using the Kruskal-Wallis ANOVA on ranks and the Dunn's test for post hoc contrasts. The slopes and intercepts of the relationship between exertional dyspnea scores (Borg) and VE, Wpeak and VO2 (% predicted maximum) from the initial incremental exercise test were determined by means of a linear regression analysis. A stepwise multivariate logistic regression was used to identify the contribution of the independent variables on the likelihood of spontaneous PLB performance during exercise, with the dichotomous outcome variable defined as the presence or absence of spontaneous PLB during exercise. Only variables significant by univariate analysis were included in the logistic regression model. The level of significance for all statistical tests was set to p < .05. A statistical analysis was performed with SPSS (SPSS; Chicago, IL, USA).
RESULTS
Fifty-seven COPD patients, 39 males and 18 females, aged 66 ± 7 years, completed the study. Of these, six (10.5%) were observed to use PLB during resting breathing (PLBrest); all six invariably also spontaneously performed PLB during both exercise and recovery. Eighteen patients (31.6%) began to PLB during exercise (PLBex) and they continued to PLB during recovery. Of these 18 patients, 7 began to PLB soon after exercise onset, 7 part-way through exercise, and 4 near the end of exercise. Seven patients (12.3%) were observed to PLB only during recovery from exercise (PLBrec). All such patients began to PLB almost immediately after stopping exercise, initially performing the breathing maneuver every breath, then gradually more intermittently before ceasing PLB altogether. The prevalence proportion of PLB in our patient sample was 10.5% (95% CI: 2.5%–18.5%) at rest, 42.1% (95% CI: 29.3%–54.9%) during exercise, and 54.4% (95% CI: 41.5%–67.3%) during recovery. Twenty patients (35.1%; 95% CI: 22.7%–47.5%) did not PLB at any time (PLBno).
PLB was used by patients over numerous consecutive breaths and there was 100% agreement between the independent observers assessing the video recordings for performance of the PLB technique. However, six patients (10.5%; 95% CI: 2.5%–18.5%) were observed to perform a variation of the PLB maneuver, such as grunting or hissing (one beginning at rest, and five during exercise), which also produced an absence of expiratory nasal airflow (Pno = 0 cm H2O) without evidence of a “pursed” lip configuration. These latter patients were excluded from a subsequent analysis because we could not ascertain that these manuevers were indeed similar to PLB. Consequently, a total of 51 patients were categorized according to when they were observed to begin PLB, generating four groups ().
Table 1. Characteristics of the 51 patients with COPD stratified according to the four PLB groups
Pulmonary function and physiological variables at rest
Spirometry results, presented in and , show significant group differences in the FEV1%predicted, FEV1/FVC%, and FEF25–75%predicted (ANOVA p < .001 for all three). With respect to lung volumes (), patients in PLBrest and PLBex groups revealed a higher FRC%pred compared with PLBno patients (p < .05) whereas the PLBrest group exhibited the highest RV/TLC% predicted (p < .05), the lowest diffusion capacity, and were significantly more hypercapnic than the other three groups (). PLBrest patients had a higher resting HR than PLBno patients. Groups PLBno and PLBrec were indistinguishable with respect to any baseline pulmonary function variables measured.
Figure 2. The average values (±SD) for the (a) FEV1 (% predicted), (b) FEV1/FVC (%), and (c) FEF 25–75 (% predicted) in the four PLB groups. P values indicate significance in post hoc pairwise comparisons.
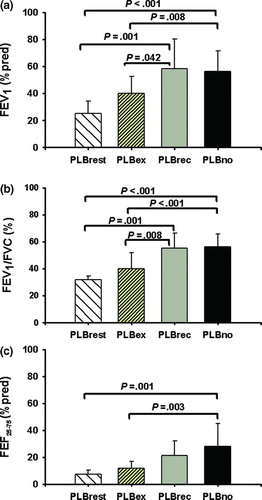
Figure 3. The relationship of (a) dyspnea measured using a modified Borg scale and VE and (b) dyspnea to W% predicted max. Lines represent the average slopes for the four PLB groups. Shaded areas represent ±SE for the slopes. Error bars are ±SE for dyspnea. Solid lines and cross hatched areas, PLBrest; long dashed lines and dark grey areas, PLBex; short dashed lines and light grey areas, PLBrec; dotted lines and diagonally lined areas, PLBno.
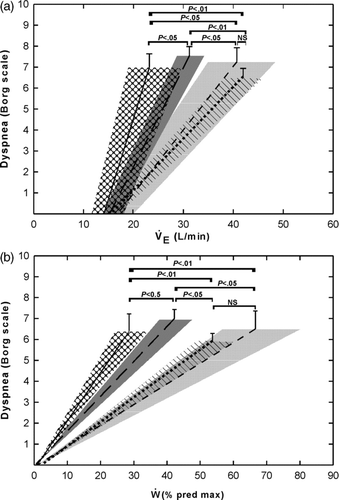
Physiological variables during exercise
As shown in , PLBrest patients had the lowest exercise tolerance, whereas the highest exercise levels and VO2 peak were achieved by PLBno and PLBrec patients. There were no group differences with regard to the respiratory and heart rates at end exercise. PLBrest patients desaturated more during exercise, and thus displayed the lowest SaO2 at end exercise ().
Table 2. Physiological parameters at end exercise in 51 patients with COPD stratified according to the four PLB groups
Breathlessness
Clinical dyspnea scales. The baseline dyspnea scores are presented in . In general, PLBrest and PLBex patients were functionally more limited by breathlessness than PLBrec and PLBno patients.
Dyspnea during incremental exercise. Out of the 57 patients studied, 72% stopped exercise due to dyspnea, 18% due to leg fatigue, and 11% secondary to both dyspnea and leg fatigue. The majority of patients in each of the four PLB groups stopped exercising due to dyspnea (). However, the average Borg dyspnea scores at end exercise were not significantly different across the four groups ().
The group means (± SE) of the individual slopes for the relationships of dyspnea versus VE and W% pred max are presented in , respectively. Highly significant individual correlation coefficients were obtained in all subjects for both the dyspnea/VE (r = .93 ± .05; range 0.78–0.99) and dyspnea/W% pred max (r = .96 ± .04; range 0.85–0.99) relationships. As shown in , the average slopes relating dyspnea to VE were significantly different in the four PLB groups (one-way ANOVA p < .01). PLBrest patients exhibited the greatest increases in dyspnea for any change in VE, whereas the smallest increases were observed in the PLBrec and PLBno groups. Similar results were obtained for the slopes of the relationship of dyspnea/W% pred max (; one-way ANOVA p < .01). When VE was expressed as a percentage of MVV, average slopes relating dyspnea to VE%MVV were similar across the four PLB groups.
Logistic regression
Presented in are the variables identified from univariate analysis as being significantly associated with the performance of spontaneous PLB during exercise by patients with COPD. From the pulmonary function tests, the greatest odds of performing PLB were predicted by the ratio of FEV1/FVC, such that for every 10% increase in FEV1/FVC, patients were 4.2 times less likely to PLB during exercise. With respect to exercise performance, a 10% reduction in either the O2 pulse (%pred) or the VO2 (%pred) at peak exercise was associated with a threefold increase in the likelihood of PLB being performed during exercise. The level of dyspnea experienced by patients, measured using the OCD, was found to be significantly related to PLB performance. However, the most significant predictor of PLB use was the slope of the relationship of dyspnea to VE during exercise. Patients exhibiting a greater dyspnea response to incremental exercise (dyspnea/VE slope > 0.3) were ∼23 times more likely to PLB than patients exhibiting a lower dyspnea response (slope ≤ 0.3) ().
Table 3. Univariate logistic regression models for the performance of PLB during exercise
presents the model found to be most predictive and parsimonious of spontaneous PLB performance during exercise in patients with COPD. Using this model, we found that when controlling for the confounding of FEV1/FVC, patients with a dyspnea/VE slope > 0.3 were ∼15 times more likely to PLB during exercise. Similarly, adjusting for the dyspnea/VE response, a 10% decrease in FEV1/FVC was associated with ∼3.6 times greater likelihood of PLB. Moreover, the model shows that patients with an FEV1/FVC ratio of 40% and exhibiting a dyspnea/VE slope > 0.3 were ∼55 times more likely to PLB compared with patients whose FEV1/FVC was 50% and dyspnea/VE slope was ≤0.3.
Table 4. Multivariate logistic regression model for the performance of PLB during exercise
DISCUSSION
A little over half of our COPD patients were observed to PLB at some point during testing: 10% performed spontaneous PLB at rest, 42% during incremental bicycle exercise, and 54% during recovery from exercise. Moreover, ∼10% of the patients performed other expiratory resistive maneuvers such as grunting, such that ∼65% of our patients breathed using maneuvers which involved increasing the resistance to expiration. Although several studies have claimed that PLB is frequently employed by COPD patients (Citation1–3), only one previous study serendipitously reported that 22% of the patients studied performed spontaneous PLB during an incremental shuttle walk test (Citation19).
Airflow limitation
In general, PLBrec patients were indistinguishable from PLBno patients. Such patients exhibited a moderate degree of airflow obstruction and tended to have normal or slightly elevated lung volumes, although the variability was quite large in both groups. These patients also exhibited low PaO2 values despite a normal DLCO, suggesting chronic bronchitis as the underlying pathophysiology. In contrast, PLBex and PLBrest patients were significantly more airflow limited than PLBno and PLBrec patients. PLBrest patients in our study exhibited severe airflow obstruction, higher resting heart rates, increased PaCO2 reduced DLCO/VA (%predicted), and the greatest increases in static lung volumes, which would indicate the presence of emphysema and suggests that these patients may have a relatively worse prognosis.
Exercise tolerance
Typical of patients with COPD, all four groups in our study demonstrated a reduced exercise tolerance (Citation20–24). The extent to which exercise capacity is reduced depends on the severity of airflow limitation (FEV1) (Citation20, Citation22, Citation23, Citation25). That FEV1 likely also contributed to the differences found in peak exercise VE in our study is substantiated by the fact that such differences disappeared when VE max was normalized to the predicted MVV.
PLBrest patients exhibited the smallest absolute tidal volumes; the largest volumes were obtained in PLBrec and PLBno patients. Even with normalization of such volumes to the vital capacity, PLBrest patients continued to exhibit the smallest mean VT/VC%. Moreover, a univariate analysis revealed that patients with a VT /VC ratio <37% were ∼17 times more likely to PLB during exercise than patients who had a VT/VC ≥ 37%. Studies have demonstrated that patients with more severe airflow limitation develop smaller increases in VT during maximum exercise (Citation26, 27) in part due to exercise-induced dynamic hyperinflation (Citation25, Citation27–30). In the presence of an already increased resting FRC, exercise-induced dynamic hyperinflation would cause VT to encroach upon the inspiratory reserve volume, and in some patients, to approach the limits of TLC, leading to a blunting of the VT response (Citation27, Citation30). Moreover, dynamic hyperinflation compromises the mechanical advantage of the inspiratory muscles, forcing them to work at a higher fraction of their force generating capacity, and has been associated with increased exertional breathlessness (Citation30, 31). We however did not measure dynamic hyperinflation in our study for fear that the performance of the inspiratory capacity maneuvers by our subjects would interfere with the natural occurrence or use of PLB in the second exercise session and also wanted to ensure that similar procedures were used in the two exercise runs. Thus, we can only speculate on the mechanism contributing to PLB performance in our patient population.
The univariate logistic regression model identified several factors as significantly associated with spontaneous PLB use during exercise in COPD patients (). However, the most predictive multivariate model comprised only two covariates: a measure of airflow obstruction (FEV1/FVC%) and the dyspnea experienced during incremental exercise (). Our results indicate that the key factor involved is not the absolute level of dyspnea perceived during exercise (dyspnea scores at end exercise averaged ∼7 in all four groups) but rather the rate of dyspnea increase with increasing minute ventilation. The more amplified the dyspnea response during exertion, the greater the likelihood of PLB being performed.
Study's limitations
The exercise protocol used in this study could have contributed to the findings obtained. Exercise workload was increased every 2 minutes and in order not to excessively prolong total exercise time, we opted to use 15 watt workload increments. We cannot dismiss the possibility that another exercise strategy could have resulted in an alternate pattern of PLB use.
Given that our subjects were recruited from a hospital center having no established pulmonary rehabilitation program, it is unlikely that patients who performed PLB in our study were taught the breathing maneuver. However, we did not enquire as to whether subjects had been exposed to the technique in some other context and thus cannot be certain that the PLB performed was self-taught (acquired) or the reason for its use.
The study did not set out to provide insights into mechanisms by which PLB may alter dyspnea. Consequently, we did not evaluate whether dyspnea was reduced during the second exercise session when PLB performance was evaluated. Furthermore, it would be impossible to separate the effect of no mouthpiece versus PLB on dyspnea during the second exercise session. Also, PLB was not necessarily performed throughout the entire exercise period and thus determination of slopes of dyspnea versus workload was not feasible. Whether the presence of nasal prongs in the second exercise test influenced the use of PLB is also unknown. For similar reasons, other exercise responses during the second exercise test, i.e. heart rate, oxygen saturation, were likewise not evaluated.
For the logistic regression analysis, we defined the dichotomous outcome variable as the presence or absence of spontaneous PLB during exercise, based on the fact that patients are most often taught to use PLB during exercise when the breathing maneuver is thought to optimally alleviate dyspnea. However, dichotomizing according to whether or not PLB was performed at any time versus not at all could have provided different results.
In summary, whereas only ∼10% of stable COPD in our sample were found to spontaneously PLB at rest, the proportion increased to 42% when ventilation was stimulated by exercise, and 54% during recovery after exercise. Only 12% of patients were found to commence PLB during recovery and they were indistinguishable from those who did not PLB at any time with respect to baseline anthropometric characteristics, pulmonary function, dyspnea, and exercise performance. Patients who performed PLB during exercise were more severely airflow limited, more functionally debilitated by dyspnea, and revealed lower exercise capacities than patients who did not. Patients who performed PLB at rest, however, revealed the greatest degree of airflow limitation and static hyperinflation, as well as chronic hypercapnia and a reduced DLCO.
Declaration of interest
The authors report no conflicts of interest. The authors alone are responsible for the content and writing of the paper.
ACKNOWLEDGMENT
J. Spahija was the recipient of a research fellowship award from the Fonds de la Recherche en Santé du Québec.
REFERENCES
- Thoman R, Stoker G, Ross J. The efficacy of pursed lips breathing in patients with chronic obstructive pulmonary disease. Am Rev Respir Dis 1966; 93:100–106.
- Mueller RE, Petty TL, Filley GF. Ventilation and arterial blood gas changes induced by pursed lips breathing. J Appl Physiol 1970; 28:784–789.
- Barach AL. Physiologic advantages of grunting, groaning, and pursed-lip breathing: adaptive symptoms related to the development of continuous positive pressure breathing. Bull NY Acad Med 1973; 49:666–673.
- Ingram RM, Schilder DP. Effect of pursed lips expiration on the pulmonary pressure–flow relationship in obstructive lung disease. Am Rev Respir Dis 1967; 96:381–388.
- Spahija J, de Marchie M, Grassino A. Effects of imposed pursed-lips breathing on respiratory mechanics and dyspnea at rest and during exercise in COPD. Chest 2005; 128:640–650.
- Breslin EH. The pattern of respiratory muscle recruitment during pursed-lip breathing. Chest 1992; 101:75–78.
- Tiep BL, Burns M, Kao D, Pursed lips breathing training using ear oximetry. Chest 1986; 90:218–221.
- Bianchi R, Gigliotti F, Romagnoli I, Chest wall kinematics and breathlessness during pursed-lip breathing in patients with COPD. Chest 2004; 125:459–465.
- American Thoracic Society. Standardization of spirometry, 1994 update. Am J Respir Crit Care Med 1995; 152:1107–1136.
- Morris JF, Koski A, Johnson LC. Spirometric standards for healthy nonsmoking adults. Am Rev Respir Dis 1971; 103:57–67.
- Crapo RO, Morris AH, Clayton PD, Nixon, CR. Lung volumes in healthy nonsmoking adults. Bull Europ Physiopath Resp 1982; 18:419–425.
- Crapo RO, Morris AH. Standardized single breath normal values for carbon monoxide diffusing capacity. Am Rev Respir Dis 1981; 123:185–189.
- Black LF, Hyatt RE. Maximal respiratory pressures: normal values and relationship to age and sex. Am Rev Respir Dis 1969; 99:696–702.
- Jones NL, Makrides L, Hitchcock C, McCartney N. Normal standards for an incremental progressive cycle ergometer test. Am Rev Respir Dis 1985; 131:700–708.
- Jones NL, Campbell EJM. Clinical exercise testing; 2nd ed. Philadelphia, PA: W.B. Saunders, 1982; pp. 249.
- Fletcher CM, Elmes PC, Wood CH. The significance of respiratory symptoms and the diagnosis of chronic bronchitis in a working population. Br J Med 1959; 1:257–266.
- McGavin CR, Artvinli M, Naoe H, Dyspnoea, disability, and distance walked: comparison of estimates of exercise performance in respiratory disease. Br Med J 1978; 2:241–243.
- Rodenstein DO, Stanescu DC. Absence of nasal air during pursed lips breathing. Am Rev Respir Dis 1983; 128:716–718.
- Garrod R, Dallimore K, Cook J, Davies V, Quade K. An evaluation of the acute impact of pursed lips breathing on walking distance in nonspontaneous pursed lips breathing chronic obstructive pulmonary disease patients. Chron Respir Dis 2005; 2:67–72.
- Babb TG, Viggiano R, Hurley R, Effect of mild-to-moderate airflow limitation on exercise capacity. J Appl Physiol 1991; 70:223–230.
- Marcus JH, McLean RL, Duffell GM, Exercise performance in relation to the pathophysiologic type of chronic obstructive pulmonary disease. Am J Med 1970; 49:14–22.
- Jones NL, Jones G, Edwards RHT. Exercise tolerance in chronic airway obstruction. Am Rev Respir Dis 1971; 103:477–491.
- Spiro SG, Hahn HL, Edwards RHT, An analysis of the physiological strain of submaximal exercise in patients with chronic obstructive bronchitis. Thorax 1975; 30:415–425.
- Lewis MI, Belman MJ, Monn SA, The relationship between oxygen consumption and work rate in patients with airflow obstruction. Chest 1994; 106:366–372.
- Babb TG, Long KA, Rodarte JR. The relationship between maximal expiratory flow and increases of maximal exercise capacity with exercise training. Am J Respir Crit Care Med 1997; 156:116–121.
- Koulouris NJ, Dimopoulou I, Valta P, Detection of expiratory flow limitation during exercise in COPD patients. J Appl Physiol 1997; 82:723–731.
- O’Donnell DE, Revill SM, Webb KA. Dynamic hyperinflation and exercise intolerance in chronic obstructive pulmonary disease. Am J Respir Crit Care Med. 2001; 164:770–777.
- Stubbing DG, Pengally LD, Morse JLC, Pulmonary mechanics during exercise in subjects with chronic airflow obstruction. J Appl Physiol 1980; 49:511–515.
- Dodd DS, Brancatisano T, Engel LA. Chest wall mechanics during exercise in patients with severe chronic air-flow obstruction. Am Rev Respir Dis 1984; 129:33–38.
- O’Donnell DE, Webb KA. Exertional breathlessness in patients with chronic airflow limitation. Am Rev Respir Dis 1993; 148:1351–1357.
- O’Donnell DE, Bertley JC, Chau LK, Qualitative aspects of exertional breathlessness in chronic airflow limitation. Am J Respir Crit Care Med 1997; 155:109–115.