ABSTRACT
The airways of patients with chronic obstructive pulmonary disease (COPD) are associated with increased numbers of inflammatory cells, in particular neutrophils and macrophages. Contained within the primary granules of neutrophils is the heme enzyme called myeloperoxidase (MPO) that has been used as a biomarker of neutrophilic inflammation in COPD and other inflammatory diseases. MPO is the only enzyme in the body that produces hypochlorous acid (HOCl), which effectively chlorinates tyrosine residues in proteins. The presence of 3-chlorotyrosine (3Cl-Tyr) in sputum of COPD patients has yet to be established. Spontaneously produced sputum was collected from 14 stable COPD patients, and ultra-centrifuged to prepare sol phase samples for analysis. Sputum 3Cl-Tyr levels were measured using Mass Spectrometry (LC-MSMS). Sputum MPO activity was measured using a standard chromogenic substrate assay. The Spearman rank correlation was used to analyse the data. We report for the first time the measurement of 3Cl-Tyr in sputum from stable COPD patients. The sputum levels of 3Cl-Tyr correlated well with sputum MPO activity (r = 0.88; p < 0.0001). The presence of 3Cl-Tyr in the sputum of stable COPD patients suggests an active process related to MPO that may play a role in the pathophysiology of this disease.
INTRODUCTION
Chronic obstructive pulmonary disease (COPD) is characterised by a slowly progressive and irreversible deterioration in lung function leading to airflow limitation, confirmed by spirometry (Citation1, 2). Inflammatory cells are associated with the pathogenesis of COPD and there are many reports of elevated neutrophil numbers in the airways and sputum of patients with COPD (Citation3–5). Other respiratory diseases such as bronchiectasis (BrX) (Citation6), cystic fibrosis (CF) (Citation7, 8), idiopathic pulmonary fibrosis (IPF) (Citation9) and acute respiratory distress syndrome (ARDS) (Citation10,11) are also associated with neutrophilic inflammation of the airway and with increased levels of myeloperoxidase (MPO) activity (Citation12–14). In these diseases MPO levels show good correlation with disease severity suggesting that neutrophils and/or their products play a central role in their pathophysiology.
MPO is a unique heme enzyme found mainly in the primary granules of neutrophils, but also in macrophages and monocytes (Citation15). Stimulation of neutrophils leads to activation of superoxide generating NADPH oxidase, as well as the release of MPO by degranulation (Citation16, 17). Superoxide produced from NADPH oxidase is rapidly dismutated to form hydrogen peroxide (H2O2), which is utilised by MPO along with physiological concentrations of chloride (Cl−) to produce hypochlorous acid (HOCl). HOCl (the active ingredient in bleach) is a crucial mediator in microbial killing, but can also evoke inflammation and cause oxidative damage to various cellular or extracellular constituents. For these reasons oxidants and/or their effects have been implicated in the inflammation and destruction of tissues in many diverse lung diseases. HOCl (and hence indirectly MPO) can also cause oxidative modification of tyrosine to form a biomarker specific for reactive chloride species—3-chlorotyrosine (3Cl-Tyr) (Citation18, 19).
The presence of 3Cl-Tyr in biological fluids is thus a footprint of MPO activity in vivo (Citation20). Levels of 3Cl-Tyr have been measured in inflammatory diseases such as CF (Citation21), ARDS (Citation22), atherosclerosis (Citation23), rheumatoid arthritis (Citation24) and renal failure (Citation25) supporting this hypothesis.
Since COPD is classically associated with neutrophilic inflammation and increased levels of MPO activity in airway secretions, we examined sputum from COPD patients for the presence of 3Cl-Tyr and investigated its relationship with airway inflammation and MPO activity.
METHODS
Patients and sputum collection
The study for collection of sputum collection from COPD patients was approved by the Research Ethics Committee of Hertfordshire (LREC number 06/Q0201/2).
COPD patients
Fourteen patients aged 50–78 years were recruited, from routine outpatient clinic visits, to the study. All had had a clinical diagnosis of COPD for at least 2 years and had moderate to severe disease according to GOLD criteria (Citation2). Patients were daily sputum producers and were current or ex-smokers with a smoking history of ≥ 10 pack years. All patients had an FEV1 of ≥26% and ≤50% predicted, an FEV1/FVC ≤70% and an increase in FEV1 of less than 12% predicted and/or less than 200 mL following inhalation of 400 μg of salbutamol. All patients had stable symptoms of COPD for at least 8 weeks prior to the study and had no changes in medications, including inhaled and oral corticosteroids, during this time. Concomitant lung disease was excluded by high-resolution computed tomography (HRCT).
Sputum collection and processing
Spontaneous sputum samples were collected over a 4-hour period from waking. Patients were instructed to rinse their mouth with water to minimise salivary contamination prior to sample collection. The samples were divided into two equal aliquots: the first was ultracentrifuged (50,000 g for 90 min at 4°C) to prepare a sol phase sample to determine levels of 3Cl-Tyr and MPO activity. The second was treated with dithiothreitol (DTT) to assess total cell numbers and cytospins were prepared for total and differential cell counts of squamous cells, neutrophils, eosinophils, macrophages and lymphocytes as previously described (Citation28). Where relevant, patients were asked to abstain from smoking from waking until sputum collection was complete.
Measurement of 3Cl-Tyr
Protein Hydrolysis
Hydrogen bromide (HBr) was used for protein hydrolysis because preliminary experiments demonstrated that 3Cl-Tyr was generated in L-Tyrosine exposed to hydrochloric acid (HCl). The patient sol phase sputum (100 μl) was prepared, as described in the previous section, and aliquoted into a 96-deep-well glass reaction plate (Zinesser). One hundred μl of 6 M HBr containing 1% phenol was then added to the reaction plate and incubated at 120°C for 24 h in an oil bath. The reaction plate was then placed on a heating block and the samples were concentrated to dryness under nitrogen. The dried samples were then reconstituted in 250 μl of distilled water and finally spun at 3400 rpm, to remove insoluble debris, prior to being transferred to a 96-well plate for LC-MSMS analysis.
Mass Spectrometric Analysis
The LC-MSMS analysis of 3Cl-Tyr was performed using a Finnigan TSQ Quantum Ultra triple-quadrupole mass spectrometer equipped with a Surveyor auto sampler and low flow flux pumps (Thermo Electron Corporation). Column separation was achieved by running an isocratic system of 20% methanol (0.1% Formic acid) and 80% water (0.1% Formic acid) on an Atlantis C18 3 μm 1×150 column (Waters) at a flow rate of 75 μl/min for 5 min.
The mass spectrometer was equipped with an electrospray ionisation interface operated in positive ion mode with a spray voltage of 3500 V. Capillary temperature was set to 350°C and nitrogen (99.99%; BOC) was used as sheath gas at a pressure of 40 mTorr. The 3Cl-Tyr was measured by selected reaction monitoring (SRM). Two transitions for 3Cl-Tyr were identified as being m/z 216.06 → 168.98 and m/z 216.06 → 134.9, the former being chosen for analysis due to its greater abundance and higher signal to noise ratio.
Argon (99.99%; BOC) was used as the collision gas and was maintained at a constant pressure of 1.5mTorr and collision energy for the chosen transition was 12V. Both Q1 and Q3 mass analysers were operated under unit resolution of 0.1 Da full width at half maximum and 0.7 Da full width at half maximum respectively. The described conditions were optimised to achieve the best sensitivity for 3Cl-Tyr and Xcaliber (Ver. 1.4; Thermofinnigan) was used to control the HPLC/TSQ system and acquire and process the data.
Determination of MPO activity
Sputum myeloperoxidase activity was measured by chromogenic substrate assay relative to a preparation of lysed neutrophils as previously described (Citation3, Citation8) and quantified against a pure standard. Ten microlitres of standard or sample were added to the wells of a microtitre plate, followed by 150 μl of 1 mg/ml (w/v) O-dianisidine dihydrochloride, 0.01% (v/v) 30% H2O2 in 50 mM K3HPO4 and 0.5% w/v hexadecyl trimethyl ammonium bromide (pH6). The plate was then incubated for 15 minutes at 25°C and the absorbance measured at 450nm using a Dynatech MR 5000 microplate reader. The MPO concentration was interpolated from the standard curve and expressed as mg/l. The lower limit of detection is 2.94 mg/l with an intra-assay coefficient of variation of 3.6% and recovery of pure MPO from spiked samples of 103%.
MATERIALS
Chemicals
All chemicals were purchased from Sigma-Aldrich (Gillingham, UK) unless stated otherwise.
Data analysis
For the purpose of investigating inter-relations between 3Cl-Tyr and other sputum measures all data were logged to normalise the distribution. Correlation coefficients were assessed using the Pearson and Spearman rank correlation methods.
RESULTS
Patient characteristics
Individual patient characteristics are outlined in . No patients were on medications known to modulate MPO activity or 3Cl-Tyr.
Table 1. Characteristics of all patients
Sputum collection
All patients were able to produce a sputum sample of sufficient volume to be able to conduct the required analyses. Sputum sample weights ranged from 2.2 to 28.3 g ().
Table 2. Table of sputum characteristics, cell counts, MPO activity and 3Cl-Tyr levels for individual patients
Total and differential cell counts in sputum
The median cell viability in the COPD sputum collections was 80% (IQR 39.2 –89.6%) and the median squamous cell contamination was 2.5% (IQR 0.25 –5.75%). One subject had a squamous cell contamination of 21% and as a result the neutrophil percentage or total count was not included from this patient's sputum sample. For the remainder, the mean total cell count was 5.42 (range 0.5 to 26.3) × 106/g and the mean neutrophil count was 4.42 (range 0.2 to 24.9) × 106/g (see ).
3Cl-Tyr and MPO levels in sputum sol phase
Levels of 3Cl-Tyr in sputum sol phase from stable COPD patients were quantified against a standard curve constructed using authentic 3Cl-Tyr in phosphate-buffered saline (PBS). The standards were hydrolysed alongside the sputum samples as described in the methods section. PBS was used due to unavailability of a suitable sputum blank matrix from control healthy subjects. An example of a 3Cl-Tyr standard curve is illustrated (). The 3Cl-Tyr peak in the sample chromatograph was identified according to the retention time of the authentic 3Cl-Tyr standard () and an example chromatograph of a COPD sputum sample is shown in Figure 2B. The lowest standard was 0.5 ng/ml equivalent to 50 pmol (10 μl sample injected into column). The signal to noise ratio of the detected 0.5 ng/ml peak in the standard solution was nearly 200 suggesting that a far lower limit of quantification (LOQ) was possible (). Detection of 3Cl-Tyr remained linear up to 1000 ng/ml (100 nM; data not shown). It was not deemed necessary to test beyond this concentration.
Figure 1. Showing a portion of a 3Cl-Tyr standard curve to emphasis its linear nature and LOQ. Standard curves were constructed upto 1000 ng/ml with linearity being maintained to this point
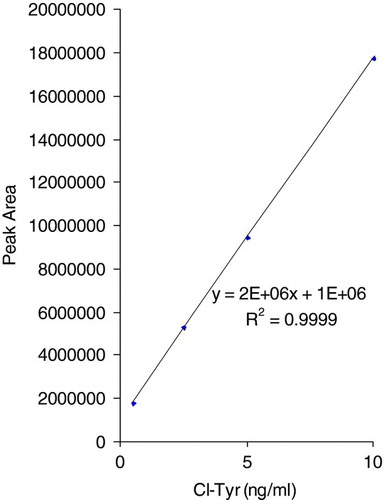
Figure 2. A) shows a chromatograph obtained from a 0.5 ng/ml 3Cl-Tyr in PBS standard. B) shows an example chromatograph obtained from a COPD sputum sample.
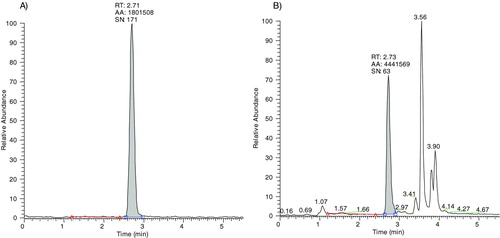
There was a wide range in the concentration of 3Cl-Tyr detected in the sputum sol phase from stable COPD patients (0–68 ng/ml; ). In 2 patients, no detectable 3Cl-Tyr was found in the collected sputum samples, and these contained the lowest levels of MPO activity. The MPO activity in the sputum sol phase also covered a wide range of activity ranging from 10.59 μg/mL to 174.19 μg/mL as summarised in .
Relationship of 3Cl-tyr levels with inflammation
MPO activity neutrophil numbers showed a significant correlation (r = 0.636 p < 0.05) and the data is summarised in Figure 3.
The 3Cl-Tyr showed a significant but weak correlation with neutrophil numbers in sputum (r = 0.37 p < 0.05) as summarised in . However, MPO activity correlated highly with 3Cl-Tyr (r = 0.88; p < 0.0001) as summarised in .
DISCUSSION
The current data uniquely shows a clear and strong correlation between MPO and 3Cl-Tyr in sputum from stable patients with COPD. The role of MPO in inflammatory diseases has gained increasing scientific interest over recent years (Citation27–29). It is the only enzyme in the body that produces HOCl, which is thought to be strongly associated with host defence and incidental cellular damage seen at the site of inflammation. The 3Cl-Tyr is thus a specific marker for MPO activity in vivo and has previously been measured using a variety of techniques, including high pressure liquid chromatography-electrochemical detection (HPLC-EC), nuclear magnetic resonance spectroscopy (NMR) and mass spectroscopy (MS) (Citation21, Citation30–32). Of these, the latter is the most reliable and sensitive assay and hence was chosen for the current study. Positive results were seen for all but 2 samples, both of which had very low levels of MPO activity confirming the validity of this method.
MPO is associated with neutrophilic inflammation in many diseases and did correlate with neutrophil numbers in the current study. However, the correlation (although significant) was relatively weak. This potentially reflects 2 issues, namely that other cells, such as macrophages, (though less frequent in the samples studied here) may contribute to the total amount of MPO (although the predominant cell in the airways of this patient group was the neutrophil median 80%) and/or MPO release from neutrophil azurophil granules reflects both cell number and state of activation. This may also explain the weak correlation of 3Cl-Tyr with neutrophil numbers. Nevertheless, the strong association between MPO and 3Cl-Tyr confirms their interdependence and the potential of 3Cl-Tyr as a footprint for MPO/HOCl mediated tissue damage.
Several studies have shown that MPO and 3Cl-Tyr are potential biomarkers for other inflammatory diseases (Citation35–37). From the data generated by this study, it is tempting therefore to suggest that 3Cl-Tyr is also a potential biomarker for MPO activity in COPD.
Although we have demonstrated a relationship between 3Cl-Tyr and neutrophil number and MPO activity, which is strongly suggestive of a relationship of 3Cl-Tyr with lung inflammation, we cannot categorically state from this study that 3Cl-Tyr is raised in COPD patients compared to age-matched control subjects. This is due to the inability to collect spontaneous sputum from control subjects as by definition they would not be healthy if they expectorate. However, others have shown MPO levels are raised in COPD patients compared to controls using induced sputum [6] and because of the strong relationship of MPO with 3Cl-Tyr and neutrophils, it is likely that 3CL-Tyr is also raised in COPD.
Figure 3. Correlation between MPO activity and neutrophil numbers in sputum. Each point represents one patient sample. r = 0.636, p = 0.02.
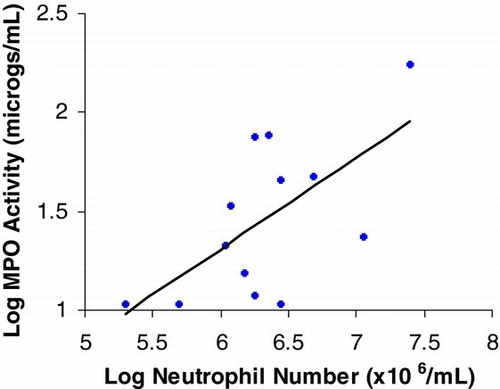
Figure 4. Correlation of 3Cl-Tyr with neutrophil number A and MPO activity B in sputum. Each point represents one patient sample. r = 0.37 (P < 0.05) for A and 0.88 (P < 0.0001) for B.
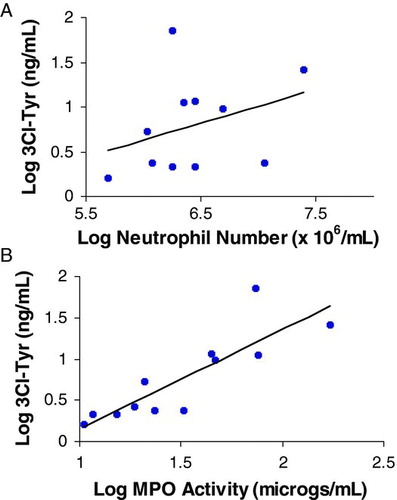
It was not possible to detect any relationship between sputum purulence and 3CL-Tyr as the number of patients included in this study was too few. This was also the case with smoking status –there were only 4 current smokers in the group of patients, so the numbers were insufficient to be able stratify them according to 3CL-Tyr levels.
We used high-speed ultracentrifugation as a means to process sputum in order to generate a supernatant for soluble mediator analysis. We purposely did not use DTT processing in order to solublise the sputum and use low speed centrifugation in order to generate a supernatant as we have previously shown that DTT dramatically reduces MPO activity (Citation26). Although it may be speculated that the high speed ultracentrifugation may disrupt already fragile neutrophils that are present in the sputum secretions, our own internal data (unpublished) indicates that although some cells may be disrupted, the intracellular granules where the MPO is stored are not disrupted and the high-speed centrifugation deposits these granules in a pellet at the bottom of the sample tubes removing them from any potential supernatant contamination.
The risk of artefactual chlorotyrosine formation was prevented by using HBr as the acid during the hydrolysis step. Furthermore, there were some sputum samples where there was no chlorotyrosine detected; this shows that the hydrolysis process did not cause tyrosine chlorination. To ensure that we were measuring 3CL-Tyr, we used selected ion monitoring to detect 3CL-Tyr in the sputum samples and the standards, then co-eluted the peak in the sputum with 3CL-Tyr standards. In addition, the mass spectrometer used was accurate to 0.1 Da.
In conclusion this study has demonstrated for the first time that the measurement of 3Cl-Tyr in the sputum of COPD patients correlates strongly with MPO activity. As such the data are indicative that MPO may contribute to tissue damage and consequently to the lung pathology in COPD, both directly and indirectly, and that 3Cl-Tyr may be a biomarker of MPO mediated damage in COPD.
ACKNOWLEDGMENTS
We thank Michael Dymond for statistical guidance in the analysis of these data and Dr Richard Weaver for his analytical expertise.
Declaration of interests
AstraZeneca supported this work. None of the authors has any potential financial conflict of interest related to this manuscript. The authors alone are responsible for the content and writing of the paper.
REFERENCES
- The COPD guidelines group of the standards of care committee of the BTS. BTS guidelines for the management of chronic obstructive pulmonary disease. Thorax 1997; 52:S1–28.
- Rabe KF, Hurd S, Anzueto A, Barnes PJ, Buist SA, Calverley P, Fukuchi Y, Jenkins C, Rodriguez-Roisin R, van Weel C, Zielinski J. Global Strategy for the Diagnosis, Management, and Prevention of Chronic Obstructive Pulmonary Disease: GOLD Executive Summary. Am J Respir Crit Care Med 2007; 176:532–555.
- Hill AT, Campbell EJ, Bayley DL, Hill SL, Stockley RA. Evidence for excessive bronchial inflammation during an acute exacerbation of chronic obstructive pulmonary disease in patients with alpha(1)-antitrypsin deficiency (PiZ). Am J Respir Crit Care Med 1999; 160:1968–1975.
- Riise GC, Ahlstedt S, Larsson S, Enander I, Jones I, Larsson P, Andersson B. Bronchial inflammation in chronic bronchitis assessed by measurement of cell products in bronchial lavage fluid. Thorax 1995; 50:360–365.
- Pesci A, Balbi B, Majori M, Cacciani G, Bertacco S, Alciato P, Donner CF. Inflammatory cells and mediators in bronchial lavage of patients with chronic obstructive pulmonary disease. Eur Respir J 1998; 12:380–386.
- Keatings VM, Barnes PJ. Granulocyte activation markers in induced sputum-comparison between chronic obstructive pulmonary disease, asthma and normal subjects. Am J Respir Crit Care 1997; 155:449–453.
- Angrill J, Agusti C, De Celis R, Filella X, RAÑÓ A, Elena M, De La Bellacasa JP, Xaubet A, Torres A. Bronchial Inflammation and Colonization in Patients with Clinically Stable Bronchiectasis. Am. J. Respir. Crit. Care Med. 2001; 164:1628–1632.
- Kettle AJ, Chan T, Osberg I, Senthilmohan R, Chapman ALP, Mocatta TJ, Wagener JS. Myeloperoxidase and protein oxidation in the airways of young children with cystic fibrosis. Am J Respir Crit Care Med 2004; 170:1317–1323.
- Garner HP, Phillips JR, Herron JG, Severson SJ, Milla CE, Regelmann WE. Peroxidase activity witin circulating neutrophils correlates with pulmonary phenotype in cystic fibrosis. J Lab Clin Med 2004; 144:127–133.
- Cantin AM, North SL, Fells GA, Oxidant-mediated epithelial cell injury in idiopathic pulmonary fibrosis. J Clin Invest 1987; 79:1665–1673.
- Lamb NJ, Gutteridge JMC, Baker C, Evans TW, Quinlan GJ. Oxidative damage to proteins of bronchoalveolar lavage fluid in patients with acute respiratory distress syndrome: Evidence for neutrophil-mediated hydroxylation, nitration and chlorination. Crit Care Med 2000; 27:1738–1744.
- Buss IH, Senthilmohan R, Darlow BA, Mogridge N, Kettele AJ, Winterbourne CC. 3-chlorotyrosine as a marker of protein damage by myeloperoxidase in tracheal aspirates from preterm infants: Association with adverse respiratory outcome. Pediatric Res 2003; 53:455–462.
- Hill AT, Bayley D, Stockley RA. The interrelationship of sputum inflammatory markers in patients with chronic bronchitis. Am J Respir Crit Care 1999; 160:893–898.
- Stockley RA, Bayley D, Hill SL, Hill AT, Crooks S, Campbell EJ. Assessment of airway neutrophils by sputum colour: correlation with airways inflammation. Thorax. 2001; 56:366–372.
- Klebanoff SJ. Oxygen metabolism and the toxic properties of phagocytes. Ann Intern Med 1980; 93:480–489.
- Babior BM. NADPH oxidase: an update. Blood 1999; 93:1464–1476.
- Mohammed JR, Mohammed BS, Pawluk LJ, Bucci DM, Baker NR, Davis WB. Purification and cytotoxic potential of myeloperoxidase in cystic fibrosis sputum. J Lab Clin Med 1988; 112: 711–720.
- Fu S, Wang H, Davies M, Dean R. Reactions of hypochlorous acid with tyrosine and peptidyl-tyrosyl residues give dichlorinated and aldehydic products in addition to 3-chlorotyrosine. J Biol Chem 2000; 275:10851–10858.
- Winterbourne CC. Biological reactivity and biomarkers of neutrophil oxidants, hypochlorus acid. Toxicol 2002; 181:223–227.
- Winterbourne CC, Kettle AJ. Biomarkers of myeloperoxidase-derived hypochlorus acid. Free Rad Biol Med 2000; 29:403–409.
- Saude EJ, Lacy P, Musat-Marcu S, Mayes DC, Bagu J, Man PSF, Sykes, BD, Moqbel R. NMR analysis of neutrophil activation in sputum samples from patients with cystic fibrosis. Magnet Reson Med 2004; 52:807–814.
- Lamb NJ, Gutteridge JMC, Baker C, Evans TW, Quinland GJ. Oxidative damage to proteins of bronchoalveolar lavage fluid in patients with acute respiratory distress syndrome: Evidence for neutrophil-mediated hydroxylation, nitration, and chlorination. Crit Care Med 1999; 27:1738–1744.
- Pennathur S, Bergt C, Shao BH, Byun J, Kassim SY, Singh P, Green PS, McDonald TO, Brunzell J, Chait A, Oram JF, O’Brien K, Geary RL, Heinecke JW. Human atherosclerotic intima and blood of patients with established coronary artery disease contain high density lipoprotein damaged by reactive nitrogen species. J Biol Chem 2004; 279:42877–42983.
- Wu SM, Pizzo SV. Alpha(2)-macroglobulin from rheumatoid arthritis synovial fluid: Functional analysis defines a role for oxidation in inflammation. Arch Biochem Biophys 2001; 391: 119–126.
- Himmelfarb J, McMenamin ME, Loseto G, Heinecke JW. Myeloperoxidase-catalysed 3-chlorotyrosine formation in dialysis patients. Free Rad Biol Med 2001; 31: 1163–1169.
- Woolhouse IS, Bayley DL, Stockley RA. Effect of sputum processing with dithiothreitol on the detection of inflammatory mediators in chronic bronchitis and bronchiectasis. Thorax 2002; 57:667–671.
- Kettle AJ, Winterbourn CC. Myeloperoxidase: a key regulator of neutrophil oxidant production. Redox. Report. 1997; 3: 3–15.
- Hoy A, Leininger-Muller B, Kutter D, Siest G, Visvikis S. Growing significance of myleoperoxidase in non-infectious diseases. Clin Chem Lab Med 2002; 40:2–8.
- Meyer KC. Neutrophils, myeloperoxidase, and bronchiectasis in cystic fibrosis: Green is not good. J Lab Clin Med 2004; 144:124–126.
- Hazen SL, Crowley JR, Muelller DM, Heinecke JW. Mass Spectrometric quantification of 3-chlorotyrosine in human tissues with attomole sensitivity-a sensitive and specific marker for myeloperoxidase-catalyzed chlorination at sites of inflammation. Free Rad Biol Med 1997; 23:909–916.
- Kettle AJ. Detection of 3-chlorotyrosine in proteins exposed to neutrophil oxidants. Meth Enzymol 1999; 300:111–120.
- Crow JP. Measurement and significance of free and protein-bound 3-nitrotyrosine, 3-chloroyrosine, and free 3-nitro-4-hydroxyphenylacetic acid in biologic samples: A high-performance liquid chromatography method using electrochemical detection. Meth Enzymol 1999; 301:151–160.
- Parr DG, White AJ, Bayley DL, Guest PJ, Stockley RA. Inflammation in sputum relates to progression of disease in subjects with COPD: a prospective descriptive study. Respir Res 2006; 7:136–146.
- Pesci A, Balbi B, Majori M, Cacciani G, Bertacco S, Alciato P, Donner CF. Inflammatory cells and mediators in bronchial lavage of patients with chronic obstructive pulmonary disease. Eur Respir J 1998; 12:380–386.
- Whiteman M, Spencer JPE. Loss of 3-chlorotyrosine by inflammatory oxidants: Implications for the use of 3-chlorotyrosine as a biomarker in vivo. Biochem Biophys Res Commun 2008; 371:50–53.
- Hazen SL. Systemic marker for monitoring anti-inflammatory and anti-oxidant activity of therapeutic agents. US Patent 0180218 A1, 2003.
- Trush MA, Egner PA, Kensler TW. Myeloperoxidase as a biomarker of skin irritation and inflammation. Fd Chem Toxic 1994; 32: 143–147.