Abstract
Introduction: Whether smoking-induced lung inflammation subsides after smoking cessation is currently a matter of debate. We used computed tomography (CT) to evaluate the effect of smoking cessation on lung density in patients with COPD. Material and methods: Thirty-six patients quit smoking out of 254 current smokers with COPD who were followed with annual CT and lung function tests (LFT) for 2–4 years as part of a randomised placebo-controlled trial of the effect of inhaled budesonide on CT-lung density. Lung density was expressed as the 15th percentile density (PD15) and relative area of emphysema below -910 HU (RA-910). From the time-trends in the budesonide and placebo groups the expected CT-lung densities at the first visit after smoking cessation were calculated by linear regression and compared to the observed densities. Results: Following smoking cessation RA-910 increased by 2.6% (p = 0.003) and PD15 decreased by −4.9 HU (p = 0.0002). Furthermore, changes were larger in the budesonide group than the placebo group (PD15: −7.1 vs −2.8 HU. RA-910 3.7% vs 1.7%). These differences were, however, not statistically significant. The LFT parameters (FEV1 and diffusion capacity) were not significantly influenced by smoking cessation. Conclusion: Inflammation partly masks the presence of emphysema on CT and smoking cessation results in a paradoxical fall in lung density, which resembles rapid progression of emphysema. This fall in density is probably due to an anti-inflammatory effect of smoking cessation.
INTRODUCTION
Smoking is associated with inflammatory changes in the central and peripheral airways. Pigment-laden macrophages accumulate in the respiratory bronchiole of smokers at an early stage (Citation1). Other features of the inflammation are infiltration by neutrophils and T-lymphocytes, smooth muscle proliferation and goblet cell hyperplasia (Citation2). Chronic inflammation results in destruction of the alveolar walls and structural remodelling of the airways in 10–20% of smokers, who develop chronic obstructive pulmonary disease (COPD) (Citation3). Smoking cessation results in improvement of symptoms of COPD (Citation3–5) and reduction of the rate of decline in lung function (Citation3, Citation6). However, whether this improvement is the result of, or is associated with decreased inflammation in the airways is still controversial (Citation7). A number of cross-sectional studies have failed to demonstrate significant differences in airway inflammation of smokers and ex-smokers (Citation8–10), while other studies have shown that inflammation rapidly subsides after smoking cessation (Citation11) or reduction (Citation12).
On computed tomography (CT) scans, accumulation of cells or fluid in the alveoli or inflammatory thickening of the alveolar walls is represented by areas of ground glass opacity (Citation13). Ground glass opacity and micronodules are common findings in smokers (Citation14), and are associated with increased tissue attenuation. As COPD progresses, the most prominent lesion in heavy smokers is centrilobular emphysema (CLE), which is characterised by tissue destruction and low attenuation of lung tissue on CT scans (Citation15). There are limited data regarding the radiological changes associated with smoking cessation. Visual evaluation of high-resolution CT (HRCT) of a limited number of quitters has suggested the regression of micronodules and areas of ground glass opacity (Citation16); i.e., decrease in lung density. This observation has led us to propose that ongoing inflammation due to continuous exposure to tobacco smoke partly masks the features of emphysema leading to underestimation of its extent on CT scans.
To date, smoking cessation is the most effective available intervention in COPD. The anti-inflammatory effect of inhaled corticosteroids (ICS) is debatable, yet there is some evidence of that effect in COPD (Citation17, 18). In addition, a number of anti-inflammatory agents are currently under evaluation for the treatment of COPD (Citation19). Different approaches can be adopted to assess the efficacy of anti-inflammatory agents, including smoking cessation and ICS. We used two densitometric parameters, namely the 15th percentile density (PD15) and relative area of emphysema below -910 Hounsfield units (HU) (RA-910), derived from annual CT scans to objectively evaluate changes in lung density following smoking cessation in patients with COPD.
MATERIAL AND METHODS
Study population
The study population was comprised of 36 heavy smokers with COPD. They were among a larger cohort of smokers followed with annual CT-scans and lung function tests for 2–4 years to monitor the progression of emphysema. In the original study, a total of 254 active smokers with COPD were randomly assigned to twice-daily treatment with either 400 μg of budesonide or placebo (Citation20). At inclusion, all patients had a smoking history of ≥20 pack-years, and smoked at least 10 cigarettes per day during the last 6 months. Patients had symptoms of COPD for ≥2 years. COPD was defined as forced expiratory volume in 1 second (FEV1) between 35% and 70% of predicted and FEV1/forced vital capacity (FEV1/FVC) less than 60% pre-bronchodilator. Patients had no previous history of atopy or asthma.
Reversibility of ≥12% and 200 ml from baseline FEV1, 15 minutes after the administration of 1 mg terbutaline implied exclusion from the study. Patients with concomitant severe pulmonary, cardiac, renal or hepatic disease were excluded from the study. Bronchodilaters, mucolytics, and short courses of oral corticosteroids (maximum 3 courses of 4-week duration per year) and antibiotics were allowed during the study. All patients were advised to quit smoking and were offered group counselling in smoking cessation. This effort resulted in smoking cessation by 36 patients, whose data are presented in this paper. The study was approved by the Ethics Committee of Copenhagen Municipality and all patients gave informed written consent. The original study population, inclusion and exclusion criteria, and study design are discussed in details elsewhere (Citation20).
Lung function tests
Annual lung function tests (LFTs) were done within 2 months before or after CT scans. LFTs were performed according to European Respiratory Society (ERS) recommendations (Citation21). A pressure compensated flow plethysmograph (SensorMedics Vmax 229, Bilthoven, The Netherlands) was used with the patient seated and a noseclip applied. The diffusion constant for carbon monoxide (KCO) was measured by the single-breath technique. The diffusion capacity (DLCO) was calculated as the product of KCO and the alveolar volume. The latter was obtained from the dilution of methane (CH4) during the single-breath manoeuvre.
CT scans
From the beginning of the study in January 1999 to March 2001, CT was performed on a Seimens (Somatom Plus 4, Erlangen, Germany) spiral scanner. The Scanning parameters were: 10 mm slice thickness at 2 pitch, 140 kVp and 200 mAs. Images were reconstructed with a high-spatial frequency (hard or sharp) algorithm. From April 2001 onwards, CT was done as a low-dose, multi-slice scan on GE equipment (GE Medical Systems, LightSpeed QX/i, Milwaukee, Wisconsin, USA) with 4 detector rows. Image acquisition was performed with 5 mm collimation and 2.5 mm increment, rotation time 0.8 seconds, with a pitchx = 1.5. The voltage across the X-ray tube was 140 kVp, with tube current of 40 mA. Images were reconstructed using low (soft) frequency algorithm. Both scanners were calibrated weekly for air and with regular intervals with a water phantom. Scanning was performed at full inspiration in a caudo-cranial direction (z-axis) to avoid breathing artefacts at the level of the diaphragm. Scanning time ranged between 10–30 seconds. No contrast medium was injected.
Quantitative parameters of CT scans
The analysis software and analysis procedure have been described elsewhere (Citation22, 23). In brief, images were analysed with Pulmo-CMS software (Medical Imaging Systems, Leiden, The Netherlands) that generates a histogram of the pixel attenuation values of the whole lung. From the histogram various quantitative CT (densitometric) parameters were extracted, e.g., total lung volume, mean lung density, 15th percentile density (PD15) and relative area of emphysema below -910 HU (RA-910) (Citation23). PD15 and RA-910 are commonly applied measures to assess the severity of emphysema from CT-images. In the current study, both parameters were adjusted for volume changes and applied to assess the influence of smoking cessation and ICS on CT lung density. Throughout the paper, the phrase “fall in lung density” is used to describe increase in RA-910 and decrease in PD15.
Statistical analysis
An ad hoc analysis of the data of the 36 quitters was applied. The current analysis is based upon the regression analysis used in the original study (Citation20). The type of scanner was included as a covariate in the regression model to adjust for any changes in the CT-density parameters as a result of scanner change. In the original study, the slope of regression of PD15 in current smokers with COPD was −1.1 g/L/year in the ICS group and −1.8 g/L/year in the placebo group; the slope of regression of RA-910 was 0.4%/year in the ICS group and 1.1%/year in the placebo group; the slope of regression of FEV1 was −54 mL/year in the ICS group and −56 mL/year in the placebo group; and the slope of regression of DLCO was −0.38 mmol/kPa/min/year in the ICS group and −0.32 mmol/kPa/min/year in the placebo group (Citation20).
Table 1 Patient characteristics, LFTs and quantitative CT parameters at inclusion
From these time-trends in the ICS and placebo groups of the original population of smokers, the expected CT-lung densities and lung function parameters at the first visit after smoking cessation were calculated by linear extrapolation. These expected values were then compared to the measured (observed) values after smoking cessation. The difference between expected and observed values attributed to smoking cessation was then tested by Mann-Whitney tests. Continuous variables are shown as mean and standard deviation (SD) unless otherwise indicated.
Table 2 Change in CT lung density and lung function in association with smoking cessation in the whole study population (n = 36)
RESULTS
Characteristics of the study population
The study group consisted of 36 well-characterised patients with COPD (24 men and 12 women, mean age [SD] 64.8 [6.8] years). At inclusion, the patients were heavy smokers with a mean (SD) smoking history of 59 (Citation25) pack-years. They had had symptoms of COPD for >7 years. The degree of airway obstruction was moderate to severe with only partial reversibility to β2-agonists and oral corticosteroids. At baseline, mean PD15 was 89 (Citation20) g/L and mean RA-910 was 17.7(8.6)% indicating the presence of mild to severe emphysema (). The patients were followed up with annual CT scans and lung function tests for 2–4 years. The follow-up scanning was performed within 1 year after smoking cessation. shows the baseline characteristics of the study population.
Longitudinal changes in PD15 and RA-910
There was a significant fall in lung density following smoking cessation in the group as a whole ( and ). The change in PD15 was −4.9 HU (95% CI −7.3 to −2.6, p = 0.0002). The change in RA-910 was 2.6% (95% CI 0.9 to 4.3, p = 0.003).
Figure 1 Effect of smoking cessation on lung density in patients with COPD. Lung density is objectively quantitated from CT scans as PD15 (15th percentile density) in Hounsfield units (HU) and RA-910 (relative area of emphysema below -910 HU), both changed significantly with smoking cessation with a p-value of 0.0002 and 0.003, respectively. Error bars represent the standard error of the mean.
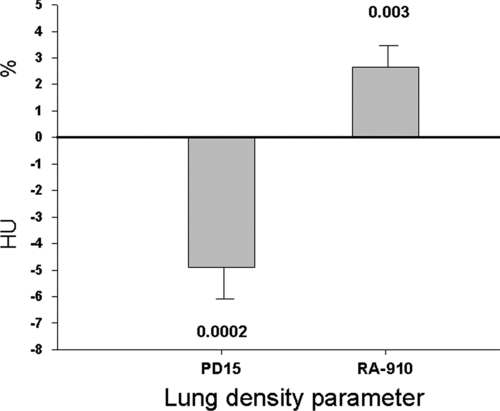
The fall in PD15 in association with smoking cessation was −7.1 HU (95% CI −10.8 to −3.4, p = 0.0009) in patients treated with budesonide, whereas the change in patients on placebo was −2.8 HU (95% CI −5.6 to 0.1, p = 0.06). The difference between the two did not reach statistical significance (p = 0.06). The increase in RA-910 in association with smoking cessation was 3.7% (95% CI 1.1 to 6.0, p = 0.007) in patients treated with inhaled budesonide, whereas the increase in patients on placebo was 1.7% (95% CI -0.8 to 4.2, p = 0.17). The difference between the two was not statistically significant (p = 0.28) ().
Longitudinal changes in FEV1 and DLCO
In the group as a whole, there was a mean increase in FEV1 of 0.072 L (95% CI -0.024 to 0.168) following smoking cessation. This increase was though not statistically significant (p = 0.14) ( and ). Neither was there a statistically significant difference between the change in FEV1 between patients on budesonide and patients on placebo (p = 0.23). The change in DLCO in the group as a whole was −0.15 (95% CI −0.56 to 0.27, p = 0.48) ( and ). There was no statistically significant difference between the change in DLCO between patients on budesonide and patients on placebo (p = 0.10).
Figure 3 Effect of smoking cessation on lung function in patients with COPD. Forced expiratory volume in one second (FEV1) and diffusion capacity (DLCO) did not change significantly with smoking cessation with a p-value of 0.14 and 0.48, respectively. Error bars represent the standard error of the mean.
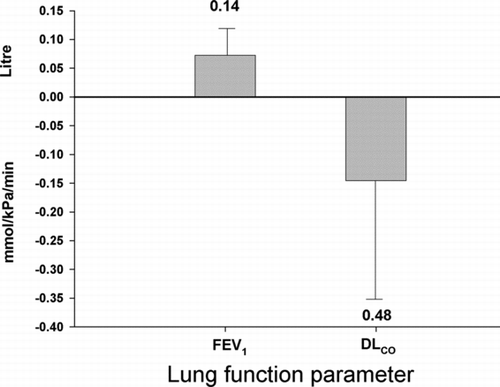
DISCUSSION
Recent guidelines recognise as part of the definition of COPD that the airway obstruction is associated with “abnormal inflammatory response” in the lung to noxious particles and gases (Citation24). Intuitively, one would expect the inflammatory response to subside, when the lung no longer is exposed to the irritants in cigarette smoke. Harada and colleagues (Citation25) exposed Syrian hamsters to smoke for 4 weeks and found that macrophage mobilisation from the lungs peaked as early as one week after smoking cessation; nevertheless, the number of macrophages in the smoke-exposed hamsters was consistently greater than in controls throughout the recovery period. Similar findings were shown in a longitudinal study of smokers (Citation11), where the recovery of macrophages in bronchoalveolar lavage (BAL) was assessed before and 1, 3, 6, 9 and 15 months after quitting smoking. The number of macrophages fell significantly already 1 month after smoking cessation with more pronounced decline at the following lavages. Moreover, decrease in the number of macrophages and neutrophils and elastase level was described after 2 months of smoking reduction from 51 to 19 cigarettes daily (Citation12).
There are few cross-sectional morphological studies comparing smokers with ex-smokers, and authors of these studies were unable to show a significant difference in the intensity of airway inflammation between the two groups (2, 8–10, 26). Wright and colleagues (Citation26) proposed that irritants in smoke induce a stereotypical inflammatory response in the airways, which does not subside after smoking cessation. Turato and colleagues (Citation9) showed that patients with persisting symptoms of chronic bronchitis 13 years after quitting had severe airway inflammation, which was not significantly different from symptomatic current smokers. These findings are in contrast to the above-mentioned studies and to the sound evidence that smoking cessation results in remarkable and sustained reduction in symptoms of cough, sputum production and wheeze (Citation3–5) and in reduction in the rate of decline of FEV1 (Citation3, Citation6, Citation27).
The radiological evidence of the morphologic changes in the lung following smoking cessation is limited, primarily because of the shortage of longitudinal CT data in smokers and particularly in quitters. Remy-Jardin and co-workers (Citation16) reported the morphologic changes as assessed by HRCT in 10 quitters before and after a mean duration of smoking cessation of 2.4 years. Areas of ground glass opacity and parenchymal micronodules, which are signs of bronchioalveolar inflammation, were found in 6 patients at baseline and only 2 at follow-up. The authors interpreted these changes as a demonstration of the beneficial influence of quitting on lung parenchyma (Citation16). However, persistence of CT abnormalities in 2 patients is supportive of the theory that smoke-induced inflammation may persist in a subgroup of patients, despite removal of the noxious agent.
In the past few years, CT lung densitometry has been used in longitudinal studies in patients with alpha-1 antitrypsin deficiency (Citation28) and smokers with COPD (Citation29). Lung densitometry has the advantages of being objective, reproducible and independent of the operator's expertise in pattern recognition. Many parameters can be extracted from the density histogram, yet we have chosen PD15 and RA-910, because they are representative of areas of lung damage by emphysema (Citation28–30). The current study shows that there was a significant fall in PD15 by 4.9 g/L (equal to 4.9 HU) within one year following smoking cessation. This can also be expressed in terms of increase in the area occupied by emphysema (relative area or emphysema index), which in the current study increased by 2.6% following quitting (). This paradoxical and sudden increase in the extent of emphysematous tissue can probably be explained by the mobilisation of inflammatory cells, especially the macrophages and neutrophils from the lung (Citation11, 12, Citation25), and by reduction in sputum production (Citation3, Citation5) resulting in “clean”, less dense lungs. Our data give no indication of the exact time of this fall in lung density, but it certainly occurs within one year; however, other data suggest that the fall occurs within 3 months following quitting (Citation11, 12). This aspect should be addressed by a prospective study preferably in combination with smoking cessation intervention.
Figure 4 Computer-aided comparison of the extent of emphysema before and after smoking cessation. The lung contours are first defined and subsequently aligned. Digital subtraction of the images with colour coding shows the abundance of areas with fall in lung density (blue) in comparison to areas with increase in lung density (yellow) (Citation31).
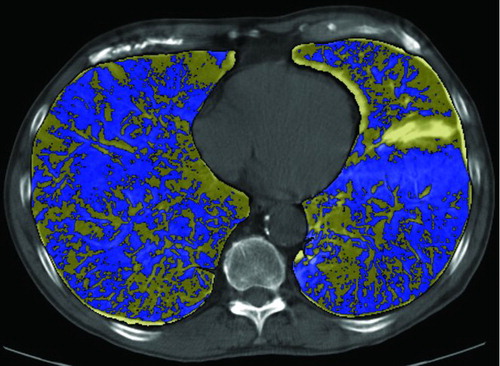
We observed a difference in the decline of PD15 following smoking cessation between patients randomised to budesonide and patients receiving placebo (). This difference was a non-significant trend, however, and does not allow the conclusion that ICS add to the clinical benefits of smoking cessation. A possible explanation of this lack of significance is the small number of patients and the short period of observation following quitting, which was limited to one year. A longer observation time is probably needed, because it is believed that the anti-inflammatory properties are more evident in long-term use of ICS in COPD, which is characterised by low-grade inflammation compared to asthma (Citation18).
The overall change in FEV1 in association with smoking cessation was 72 mL, which was not statistically significant (p = 0.14) due to the wide confidence interval. The beneficial influence of quitting on the rate of decline in FEV1 is well documented (Citation3,Citation6,Citation27). The mean improvement in FEV1 in the first year after quitting in the Lung Health Study was 47 mL (Citation6). Conversely, the influence of smoking cessation on diffusion capacity is less well documented. A study has shown that quitting resulted in stabilisation of diffusion capacity in middle-aged men over a period of 22 years compared to a significant fall in those men who continued smoking (Citation32). In the current study, diffusion capacity did not change significantly within one year of observation following smoking cessation, certainly because of the large within-subject variation in DLCO ().
The current study has two limitations, the first one is the change of CT scanner, which became necessary due to ethical considerations. During the study, new knowledge about the optimal scanning parameters for lung densitometry (Citation33) led us to modify the tube current in order to considerably reduce the radiation dose, a major ethical issue, when repeated scans are required. This change necessitated a statistical adjustment of the time trend of lung density parameters by including the type of scanner as a covariate in the regression equation. This limitation is addressed in details in the original study (Citation20), and has undoubtedly added to the level of uncertainty (i.e., the variance) of our results. The second limitation is the documentation of smoking cessation, which was solely based on the patients’ reports and was not confirmed by measurement of carbon monoxide in exhaled breath or cotinine in blood or urine.
Smoking cessation is the most effective and most important intervention available in the management of COPD. Yet a number of anti-inflammatory agents like phosphodiestrase-4 inhibitors (PDE4), interleukin-10 and others are currently under evaluation for the treatment of chronic inflammation in COPD (Citation19). There are indeed several methods to monitor changes in the inflammatory status in COPD like analysis of BAL fluid or induced sputum. We suggest the use of low-radiation-dose CT, which has the advantages of being a reproducible non-invasive method that allows both visual assessment of smoking-related lung pathology and quantitative measurement of lung density.
In conclusion, smoking cessation results in a paradoxical fall in lung density, which resembles rapid progression of emphysema. The reason of this fall in density is unclear, but might be due to reduced inflammatory burden after smoking cessation.
REFERENCES
- Niewoehner DE, Kleinerman J, Rice DB. Pathologic changes in the peripheral airways of young cigarette smokers. N Engl J Med 1974; 291(15):755–758.
- Saetta M, Turato G, Baraldo S, Goblet cell hyperplasia and epithelial inflammation in peripheral airways of smokers with both symptoms of chronic bronchitis and chronic airflow limitation. Am J Respir Crit Care Med 2000; 161(3 Pt 1):1016–1021.
- Fletcher C, Peto R, Tinker C, The natural history of chronic bronchitis and emphysema. First Ed. Oxford University Press; 1976.
- Brown CA, Crombie IK, Smith WC, The impact of quitting smoking on symptoms of chronic bronchitis: results of the Scottish Heart Health Study. Thorax 1991; 46(2):112–116.
- Kanner RE, Connett JE, Williams DE, Effects of randomized assignment to a smoking cessation intervention and changes in smoking habits on respiratory symptoms in smokers with early chronic obstructive pulmonary disease: the Lung Health Study. Am J Med 1999; 106(4):410–416.
- Scanlon PD, Connett JE, Waller LA, Smoking cessation and lung function in mild-to-moderate chronic obstructive pulmonary disease. The Lung Health Study. Am J Respir Crit Care Med 2000; 161(2 Pt 1):381–390.
- Willemse BWM, Postma DS, Timens W, The impact of smoking cessation on respiratory symptoms, lung function, airway hyperresponsiveness and inflammation. Eur Respir J 2004; 23:464–476.
- Wright JL, Lawson LM, Pare PD, Morphology of peripheral airways in current smokers and ex-smokers. Am Rev Respir Dis 1983; 127(4):474–477.
- Turato G, Di Stefano A, Maestrelli P, Effect of smoking cessation on airway inflammation in chronic bronchitis. Am J Respir Crit Care Med 1995; 152(4 Pt 1):1262–1267.
- Rutgers SR, Postma DS, ten Hacken NH, Ongoing airway inflammation in patients with COPD who do not currently smoke. Thorax 2000; 55(1):12–18.
- Skold CM, Hed J, Eklund A. Smoking cessation rapidly reduces cell recovery in bronchoalveolar lavage fluid, while alveolar macrophage fluorescence remains high. Chest 1992; 101(4):989–995.
- Rennard SI, Daughton D, Fujita J, Short-term smoking reduction is associated with reduction in measures of lower respiratory tract inflammation in heavy smokers. Eur Respir J 1990; 3(7):752–759.
- Remy-Jardin M, Remy J, Gosselin B, Lung parenchymal changes secondary to cigarette smoking: pathologic-CT correlations. Radiology 1993; 186(3):643–651.
- Remy-Jardin M, Remy J, Boulenguez C, Morphologic effects of cigarette smoking on airways and pulmonary parenchyma in healthy adult volunteers: CT evaluation and correlation with pulmonary function tests. Radiology 1993; 186(1):107–115.
- Goddard PR, Nicholson EM, Laszlo G, Computed tomography in pulmonary emphysema. Clin Radiol 1982; 33(4):379–387.
- Remy-Jardin M, Edme JL, Boulenguez C, Longitudinal follow-up study of smoker's lung with thin-section CT in correlation with pulmonary function tests. Radiology 2002; 222(1):261–270.
- Gizycki MJ, Hattotuwa KL, Barnes N, Effects of fluticasone propionate on inflammatory cells in COPD: an ultrastructural examination of endobronchial biopsy tissue. Thorax 2002; 57(9):799–803.
- Llewellyn-Jones CG, Harris TA, Stockley RA. Effect of fluticasone propionate on sputum of patients with chronic bronchitis and emphysema. Am J Respir Crit Care Med 1996; 153(2):616–621.
- Barnes PJ. Future Advances in COPD Therapy. Respiration 2001; 68(5):441–448.
- Shaker SB, Dirksen A, Ulrik CS, The effect of inhaled corticosteroids on the development of emphysema in smokers assessed by annual computed tomography. J Chron Obstruct. Pulmon. Dis. 2009; 6:104–111.
- Quanjer PH, Tammeling GJ, Cotes JE, Lung volumes and forced ventilatory flows. Report Working Party Standardization of Lung Function Tests, European Community for Steel and Coal. Official Statement of the European Respiratory Society. Eur Respir J Suppl 1993; 16:5–40.
- Stoel BC, Stolk J. Optimization and standardization of lung densitometry in the assessment of pulmonary emphysema. Invest Radiol 2004; 39(11):681–688.
- Shaker SB, Dirksen A, Laursen LC, Volume adjustment of lung density by computed tomography scans in patients with emphysema. Acta Radiol 2004; 45(4):417–423.
- Pauwels RA, Buist AS, Calverley PM, Global strategy for the diagnosis, management, and prevention of chronic obstructive pulmonary disease. NHLBI/WHO Global Initiative for Chronic Obstructive Lung Disease (GOLD) Workshop summary. Am J Respir Crit Care Med 2001; 163(5):1256–1276.
- Harada T, Basrur PK. Pulmonary macrophage mobilization in hamsters after cessation of smoking. Exp Anim 1998; 47(1):43–47.
- Wright JL, Hobson JE, Wiggs B, Airway inflammation and peribronchiolar attachments in the lungs of nonsmokers, current and ex-smokers. Lung 1988; 166(5):277–286.
- Anthonisen NR, Connett JE, Murray RP. Smoking and lung function of Lung Health Study participants after 11 years. Am J Respir Crit Care Med 2002; 166(5):675–679.
- Dirksen A, Dijkman JH, Madsen F, A randomized clinical trial of alpha(1)-antitrypsin augmentation therapy. Am J Respir Crit Care Med 1999; 160(5 Pt 1):1468–472.
- Soejima K, Yamaguchi K, Kohda E, Longitudinal follow-up study of smoking-induced lung density changes by high-resolution computed tomography. Am J Respir Crit Care Med 2000; 161(4 Pt 1):1264–1273.
- Muller NL, Staples CA, Miller RR, “Density mask”. An objective method to quantitate emphysema using computed tomography. Chest 1988; 94(4):782–787.
- Staring M, Bakker ME, Shamonin DP, Towards Local Estimation of Emphysema Progression Using Image Registration. SPIE Medical Imaging: Image Processing, Proceedings of SPIE, vol. 7259, pp. 72590O, Orlando, Florida, USA, February, 2009.
- Watson A, Joyce H, Pride NB. Changes in carbon monoxide transfer over 22 years in middle-aged men. Respir Med 2000; 94(11):1103–1108.
- Shaker SB, Dirksen A, Laursen LC, Short-term reproducibility of computed tomography-based lung density measurements in alpha-1 antitrypsin deficiency and smokers with emphysema. Acta Radiol 2004; 45(4):424–430.