Abstract
Cigarette smoke (CS) induces an inflammatory process in the lung that may underlie the development of chronic obstructive pulmonary disease (COPD). The nature and characteristics of this process have not been fully established in animal models. We aimed to evaluate the pulmonary inflammatory reaction and its involvement in structural changes in guinea pigs chronically exposed to CS. 19 Hartley guinea pigs were exposed to 7 cigarettes/day, during 3 or 6 months. 18 control guinea pigs were sham-exposed. Numbers of neutrophils, macrophages and eosinophils and lymphoid follicles were assessed in different lung structures. Airway and vessel morphometry, alveolar space size and collagen deposition were also quantified. After 6 months of exposure, CS-exposed guinea pigs showed increased numbers of neutrophils, macrophages and eosinophils in the airways, intrapulmonary vessels and alveolar septa, as well as lymphoid follicles. Increased numbers of muscularized intrapulmonary vessels were apparent at 3 months. After 6 months of exposure, the airway wall thickened and the alveolar space size increased. Collagen deposition was also apparent in airway walls and alveolar septa after 6 months’ exposure. The magnitude of airway wall-thickening correlated with the number of infiltrating inflammatory cells, and the extension of collagen deposition correlated with alveolar space size. We conclude that in the guinea pig, 6 months of CS exposure induces inflammatory cell infiltrate in lung structures, at an intensity that correlates with airway remodelling. These changes resemble those observed in COPD, thus endorsing the pathogenic role of CS and the usefulness of this animal model for its study.
Introduction
Chronic obstructive pulmonary disease (COPD) is characterized by progressive and not fully reversible airflow limitation, usually associated with a chronic inflammatory process in the airways and lung parenchyma in response to noxious particles or gases, in particular cigarette smoking (Citation1,2).
The pathogenesis of COPD involves the recruitment of neutrophils, macrophages, and lymphocytes to the lung, as well as the induction of oxidative stress, all of which result in lung parenchymal destruction and airway remodelling (Citation3–7). Full understanding of the role of inflammatory cells in COPD is difficult because this disease involves a mixture of processes (airways disease, emphysema and vascular abnormalities) with different patterns of inflammation and different pathologies.
Over the last 10 years in vivo models of lung inflammation and lung damage induced by cigarette smoke (CS) have been used to investigate mechanisms related to COPD. Their greatest advantage over other experimental models is the ability to use the primary disease-causing agent to model several key features of the disease in small animals. CS-exposure animal models therefore provide the strongest evidence for a causal link between inflammation and the structural changes associated with COPD.
The guinea pig develops, more closely than other animal models (Citation8), morphological and physiological alterations after exposure to CS that resemble those seen in COPD. The nature and characteristics of inflammatory cell infiltrate and its eventual relationship with structural changes occurring in the lung have not been fully characterized.
The present study aimed to investigate the nature and characteristics of the inflammatory cell infiltrate in the lungs of guinea pigs after two periods of CS exposure, and to assess its relationship with structural abnormalities in the airways, lung parenchyma and pulmonary vessels.
Methods
Additional information on methods is provided in the supplementary material.
Animals and experimental model
Thirty-seven male Hartley guinea pigs (∼300 g) were randomly divided into four groups. Nineteen guinea pigs were exposed to the smoke of 7 non-filtered research cigarettes (1R3F; Kentucky University Research; Lexington, KY, USA) per day, 5 days a week, using a nose-only system (Citation9) (Protowerx Design Inc; Langley, British Columbia, Canada). One group (n = 6) was exposed to CS for 3 months and the other group (n = 13) for 6 months. The other two groups were 18 control animals, sham-exposed to CS by placing them for the same length of time in the nose-only system over the same periods of time (n = 10 for 3 months and n = 8 for 6 months) without lighting the cigarettes. At the end of the 3- or 6-month period, the animals were sacrificed under anesthesia 24 h after the end of the experiments. The ethical review board for animal research of the University of Barcelona approved all the experimental protocols and the experiments were performed following institutional guidelines that comply with national and international laws and policies.
Lung tissue preparation
The lungs were removed and inflated intratracheally with 4% formaldehyde for 24 h under a constant pressure of 25 cm H2O. The lung tissue blocks were embedded in paraffin.
Characterization of inflammatory cells
The assessment of inflammatory cell infiltrate was performed on 5 μm serial sections stained with H&E to identify neutrophils and lymphoid follicles. The presence of eosinophils and macrophages was quantified on sections stained with Congo red and PAS, respectively (Citation10;Citation11).
The number of neutrophils, eosinophils and macrophages infiltrating the adventitia was counted in 10 airways (median of internal luminal perimeter (Pil), 971 μm) and in 10 pulmonary arteries (median of internal elastic lamina perimeter (Pim), 324 μm) per animal. Inflammatory cells in alveolar septa were counted in 20 fields, randomly selected at a magnification of x640. The results were expressed as the number of cells per alveolar septal area. The lung tissue sections were examined for the presence or absence of lymphoid follicles. The rate of guinea pigs with lymphoid follicles was calculated.
Morphometric measurements
Serial sections 5 μm thick were immunostained with a mouse monoclonal antibody against human smooth muscle α-actin (M0851; DakoCytomation, Glostrup, Denmark). Sites of primary antibody were revealed with an ABC system kit (PK-6102 kit; Vector Laboratories, Burlingame (CA), US) and DAB+chromogen solution as substrate (DakoCytomation).
Ten non-cartilaginous airways per animal were randomly selected and photographed using a bright field microscope (Leica Microsystems Imaging Solutions Ltd, Cambridge, UK) coupled to a digital camera (Leica). Images were processed using analysis software (Image-Pro Plus, Media Cybernetics, Carlsbad, Calif). For each airway, the outer and inner aspects of the muscular layer (Citation12) and the internal luminal perimeter (Pil) were outlined and the occupied areas were calculated. The thickness of the smooth muscle layer in airways was estimated as the difference in the delimited areas of the outer and inner aspects of the muscular layer normalized by the Pil. Similarly, the perimeter of adventitia was outlined and the area was computed. Pil was used as the internal reference measure (Citation13) to normalize all the airway dimensions. Pulmonary vessels with an external diameter <50 μm were analyzed in lung sections stained with orcein. Vessels with double elastic laminas were counted and expressed as the number per sq. mm of tissue.
Lung sections were assessed by immunohistochemistry with anti-α-actin and anti-desmin antibodies (Dako, Glostrup, Denmark). The immunoreactions to both antibodies were quantified as the number of positive vessels per sq. mm of tissue. The presence of emphysema was evaluated by measuring the mean linear intercept of alveolar septa in 20 randomly selected fields per slice using an image analysis system (Leica Qwin) in H&E-stained lung sections.
Assessment of fibrosis
Total collagen in lung structures was evaluated in paraffin-embedded sections stained with Sirius red. An average of 11 airways and 16 fields of alveolar septa per animal were randomly captured.
The percentage of alveolar septa occupied by collagen fibres and the area of muscular layer in airways occupied by collagen were determined under bright field microscopy. Thick and thin collagen fibres were identified by brightening to orange-red and green, respectively, under polarized light microscopy (Citation14). The areas for each type of fibre were evaluated by an image analysis system (Image-Pro Plus). Areas of fibrosis in the airways were normalized by Pil.
Data analysis
Results of normally distributed variables are shown as mean±standard deviation (SD) in tables and as mean±standard error of the mean (SEM) in figures. Results of non-normally distributed variables are expressed as median and interquartile range (IQR). Results in figures are showed as mean ± SEM.
Comparisons between groups were carried out by using a two-way analysis of variance (ANOVA), considering exposure and time as main factors. When significant, post-hoc pairwise comparisons were performed using the unpaired t-test or Mann-Whitney rank sum test to identify the source of variation, for normally and non-normally distributed data, respectively.
To investigate whether cell counts may differ according to the size of the airways or pulmonary vessels (Citation15), specific assessments were carried out in airways and vessels with Pil and Pim values, above (larger) or below (smaller) the median value. The occurrence of lymphoid follicles was determined by Fisher exact test, constructing a contingency table for each period of time. Relationships between variables were assessed using the Pearson's correlation test. A p-value lower than 0.05 was considered significant.
Results
Nineteen of the 24 CS-exposed animals and all the 18 non-exposed animals completed the exposure period and were used for the morphological analysis. The cause of death was attributed to severe bronchoconstriction during CS exposure. Animals showed normal behaviour and activity during the experimental procedures and they did not reveal any signs of respiratory infection or major abnormalities in the lungs after sacrifice.
Inflammatory cells
Compared with non-exposed animals, the number of neutrophils increased in airways and vessels after 6 months of CS exposure ( and ). This response was observed irrespective of the size of the airways or pulmonary vessels (). After 3 months of exposure, the number of neutrophils was already increased, suggesting a progression of the inflammatory process with prolonged CS exposure.
Figure 1. Neutrophilic infiltration in the lung of guinea pigs. Individual counts for neutrophils in the airways (A), pulmonary vessels (B) and alveolar septa (C) of controls and CS-exposed animals. Results are expressed as number of cells normalized by Pil in airways, Pim in vessels or square millimeter in alveolar septa. Horizontal bars represent median values. * p ≤ 0.05 CS-exposed vs. Control (Mann-Whitney rank sum test). Photomicrographs of an airway (D), a pulmonary vessel (E) and alveolar septa (F) of guinea pigs exposed to CS (H&E stain. Scale bar, 20 mm). Arrows show infiltrating neutrophils.
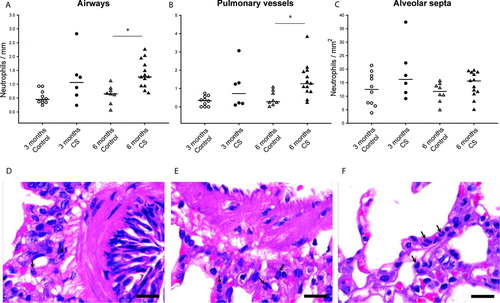
Table 1. Number of neutrophils, macrophages and eosinophils in lung structures after different periods of cigarette smoke exposure
CS-exposed animals showed an increased number of macrophages infiltrating alveolar septa, after both 3 and 6 months. The number of macrophages also increased in the adventitia of both pulmonary vessels and airways after 6 months of exposure ( and ).
Figure 2. Macrophage infiltration in the lungs of guinea pigs. Individual count for macrophages in the airways (A), pulmonary vessels (B) and alveolar septa (C) of controls and CS-exposed animals. Results are expressed as number of cells normalized by Pil in airways, Pim in vessels or square millimeter in alveolar septa. Horizontal bars represent median values. * p ≤ 0.05 CS-exposed vs. Control (Mann-Whitney rank sum test). Photomicrographs of an airway (D), a pulmonary vessel (E) and alveolar septa (F) of guinea pigs exposed to CS (PAS stain. Scale bar, 20 mm). Arrows show infiltrating macrophages.
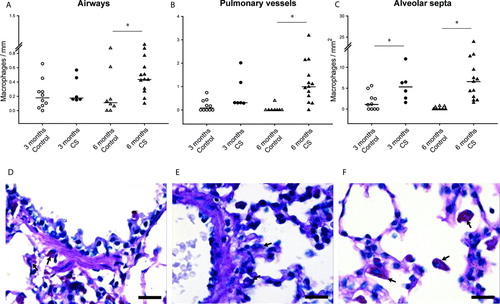
The number of eosinophils increased in airways and vessels, after both 3 and 6 months of exposure to CS. The number of eosinophils also increased in alveolar septa after 6 months. Eosinophilic infiltrate was greater in the airways than in the vessels. We did not observe any differences between larger and smaller airways ( and ).
Figure 3. Eosinophil infiltration in the lungs of guinea pigs. Individual count for eosinophils in the airways (A), pulmonary vessels (B) and alveolar septa (C) of controls and CS-exposed animals. Results are expressed as number of cells normalized by Pil in airways, Pim in vessels or square millimeter in alveolar septa. Horizontal bars represent median values. * p ≤ 0.05 CS-exposed vs. Control (Mann-Whitney rank sum test). Photomicrographs of an airway (D), a pulmonary vessel (E) and alveolar septa (F) of guinea pigs exposed to CS (Congo red stain. Scale bar, 20 mm). Arrows show infiltrating eosinophils.
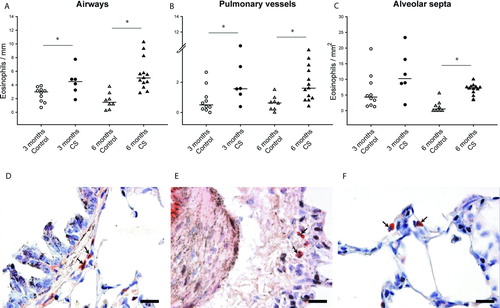
Lymphoid follicles were identified close to the airways and vessels in CS-exposed animals after 6 months of exposure (). Whereas 46% of CS-exposed animals showed follicles after 6 months of exposure, they were practically absent in sham-exposed animals (p < 0.05, Fisher exact test).
Figure 4. Pulmonary lymphoid follicles. Percentage of individuals with presence or absence of lymphoid follicles in lung tissue of control and CS-exposed groups (A).* p < 0.05 (Fisher exact test). Photomicrographs of lymphoid follicle (arrows) in peribronchial (B) and perivascular (C) lung tissue of CS-exposed guinea pigs at 6 months (H&E stain. Scale bar, 100 mm).
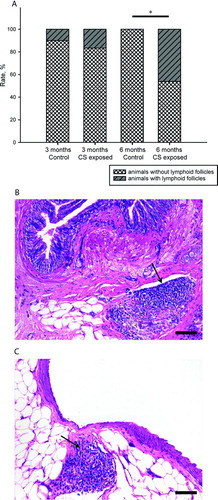
Morphometric assessments of airways
The morphometric measurements in airways from control and CS-exposed groups are shown in and . Airways were classified into larger and smaller airways according to whether they fell above or below the median value of Pil.
Figure 5. Morphometry of airways. Bar graphs show mean ± SEM of airway wall thickness, in all (A), larger (B) and smaller (C) airways in both control and CS-exposed guinea pigs, for 3 and 6 months of exposure. Immunohistochemistry for SMC a-actin in airways of a control (D) and a CS-exposed (E) animal. Scale bar, 100 mm. * p ≤ 0.05 CS-exposed vs. Control, ‡ p£0.05 6 months Control vs. 3 months Control and † p ≤ 0.05 6 months CS-exposed vs. 3 months CS-exposed (t-test).
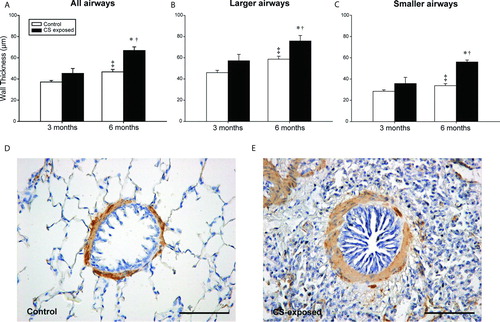
Table 2. Morphological characteristics of airways
Compared with the control group, the airway wall was thicker in the CS-exposed group after 6 months of exposure. This enlargement was apparent for both larger and smaller airways, although after 6 months of exposure the differences were more pronounced in smaller airways. It is worth noting that there was an enlargement of airway wall thickness with time, which was essentially due to the enlargement of the mucosa+submucosa, probably as a result of the maturation of lung structures during growth.
Adventitia. A significant thickening of the adventitia was observed after 6 months of CS-exposure; this was more prominent in the smaller airways.
Muscularis. A significant thickening was shown after 6 months of CS exposure, particularly in smaller airways; this was due to the increase in smooth muscle content, as shown by α-smooth muscle actin immunoreactivity.
Mucosa+submucosa. Significant enlargement of the inner wall, comprising the epithelium and the submucosa, was observed in CS-exposed animals as compared with controls, after both 3 and 6 months. There were no differences between the larger and smaller airways.
Emphysema. There was an increase in the mean distance between the alveolar septa in animals exposed to CS for 6 months (control vs. exposed: 64 ± 6 vs. 73 ± 5 μm, p = 0.001).
Morphological evaluation of pulmonary vessels
The morphometric measurements of intrapulmonary vessels are shown in . There was a trend towards a greater proportion of intrapulmonary vessels with double elastic laminas in animals exposed to CS during 6 months. The number of vessels with positive immunoreactivity to α-smooth muscle actin was higher in animals exposed to CS, after both 3 and 6 months. No differences were observed in the proportion of vessels with positive immunoreactivity to desmin ().
Table 3. Morphological characteristics of vessels
Fibrosis
Collagen deposition was evaluated under both bright field and polarized light microscopy in both the alveolar septa and the muscular layer of airways. The results for total collagen and both thick- and thin-fibre collagen are shown in and .
Figure 6. Collagen deposition in the lungs. Bar graphs show mean ± SEM of the percentage of area occupied by total collagen and thick and thin collagen fibres in the alveolar septa of control and CS-exposed guinea pigs (A). Photomicrographs of lung sections stained with Sirius red. Total collagen fibers were seen in red under bright field (B and D) and orange to red (thick collagen fibres) or green (thin collagen fibres) under polarized light (C and E). Scale bars, 100 mm. * p ≤ 0.05 CS-exposed vs. Control and † p ≤ 0.05 6 months CS-exposed vs. 3 months CS-exposed (Mann-Whitney rank sum test).
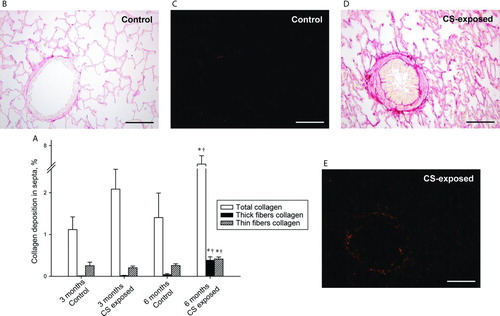
Table 4. Collagen deposition in airways
There was an increase in the proportion of collagen deposition in both the septa and the airways in animals exposed to CS for 6 months. The increase in collagen was more pronounced in smaller airways than in larger airways.
We analyzed the extension of thick- and thin-fibre collagen under polarized light. There was a greater deposition of thick-fibre collagen after 6 months of CS exposure in both the alveolar septa and the muscular layer of airways.
Correlations
The number of peribronchial neutrophils correlated with the thickness of the airway wall and its different layers, particularly in smaller airways ( and Supplementary Table 1), and also with the alveolar size (r = 0.43, p = 0.01). The number of macrophages infiltrating the airways correlated with the wall thickness of smaller airways ( and Supplementary Table 2) and the alveolar size (r = 0.37, p = 0.03). The macrophages in alveolar septa also correlated with the alveolar size (r = 0.45, p = 0.06). Moreover, the deposition of total () and thick-fibre collagen (r = 0.42, p = 0.01) in alveolar septa correlated positively with the degree of emphysema.
Figure 7. Correlations between inflammatory infiltrate and remodelling. Linear regression of neutrophils (A) and macrophages (B) with the thickness of total wall in smaller airways. Solid circles are CS-exposed animals independently of time of exposure.
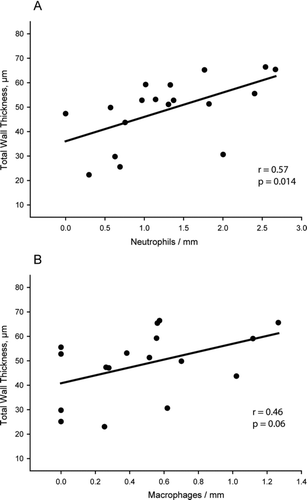
Figure 8. Emphysema in the lungs and correlation with fibrosis. Dot plot showing individual interseptal distance in each experimental group and mean (horizontal bars) (A). *p ≤ 0.05 (t-test). Linear regression of total collagen in septa with the mean linear intercept of alveolar septa (B). Solid circles are CS-exposed animals and open circles are sham-exposed animals independently of time of exposure.
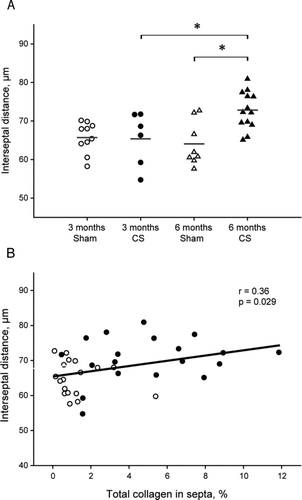
The number of peribronchial eosinophils correlated weakly with the thickness of the airway wall and its different layers (Supplementary Table 3). Only perivascular eosinophils correlated weakly with the number of α-actin-positive intrapulmonary vessels (r = 0.36, p = 0.03; ).
Supplementary Figure 9. Correlation between eosinophils and muscularization. Linear regression of perivascular eosinophils with the number of vessels per mm2 positive to a-actin in lung tissue. Solid circles are CS-exposed animals and open circles are sham-exposed animals independently of time of exposure.
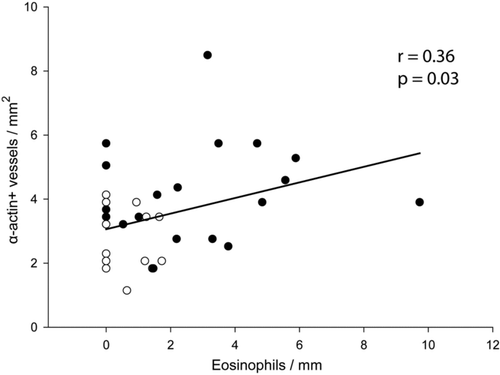
Discussion
The present study shows that guinea pigs chronically exposed to CS develop an inflammatory reaction in all their pulmonary structures, clearly apparent after 6 months of exposure, as well as morphological changes in those structures. The changes in airway structure were pronounced, especially in smaller airways, and correlated with the number of infiltrating neutrophils and macrophages. Animals exposed to CS also developed a muscularization of small pulmonary vessels that was unrelated to the neutrophilic or macrophagic infiltrate. In addition to thickening of airway walls, animals exposed to CS presented deposition of collagen in alveolar septa, which ran in parallel with the severity of the inflammatory process. After 6 months of exposure, emphysema was apparent, and this was associated with collagen deposition and an increased number of macrophages and neutrophils infiltrating the bronchial wall, and of macrophages in alveolar septa.
To our knowledge, this is the first longitudinal study evaluating the characteristics of the inflammatory cell burden in the various lung tissue structures of guinea pigs exposed to CS. Our data reveal that animals exposed to CS mimic some of the inflammatory characteristics shown in smokers, supporting the validity of this species as an experimental model of COPD.
Neutrophils
The results of the present study show that in control animals, neutrophils were preferentially located in the alveolar septa, whereas the number of infiltrating neutrophils in the airways was low. The number of neutrophils in the airways of CS-exposed animals increased progressively over time and was clearly apparent after 6 months of exposure. A consistent infiltrate was also found around pulmonary vessels, indicating that the inflammatory reaction affects these lung structures and can be detected after 3 months of exposure, while becoming clearly apparent after 6 months. These findings are consistent with studies in humans showing a non-uniform distribution of inflammatory cells in both smokers with and without COPD, and a correlation between neutrophil numbers and pack-years of smoking (Citation16). The accumulation of neutrophils is considered one of the key events in the pathogenesis of lung injury in smokers, particularly in the development of pulmonary emphysema (Citation17), because these cells can induce protease-antiprotease and/or oxidant-antioxidant imbalance(s).
In this respect, it is important to note that neutrophilic inflammatory reaction correlated with the thickness of the airway wall and its different layers, particularly in smaller airways, and with interseptal distance, suggesting that neutrophils may participate in airway remodelling and emphysema. Interestingly, Churg et al. (Citation18) demonstrated that the administration of metalloproteinase inhibitors can ameliorate morphological emphysema and enhance small-airway remodelling, thereby strengthening the role of neutrophil proteases in this condition. They did not, however, evaluate the inflammatory infiltrate to test the origin of metalloproteinases. The present study extends these previous results and identifies neutrophils as a key component in airway remodelling and emphysema.
Macrophages
An increased number of macrophages in the bronchial tree of subjects with COPD (Citation19) has been related to emphysema, suggesting they can also induce an elastolytic inflammatory response in airways exposed to CS (Citation20). In animals exposed to CS, we observed a greater increase in the number of macrophages in the alveolar septa compared with the airway adventitia. Moreover, there was a positive relationship between the alveolar size and the number of macrophages in the bronchial tree and alveolar septa, thereby providing evidence of their theoretical potential in this condition. Macrophages appear to play a pivotal role in the pathophysiology of COPD and can account for most of the known features of the disease (Citation21).
In humans, macrophages are located at sites of alveolar wall destruction and their numbers in the airways also correlate with the severity of COPD (Citation22). Studies of emphysematous lung tissue from human subjects have shown a direct relationship between alveolar macrophage density in the parenchyma and severity of lung destruction (Citation23). Our findings, and the distribution pattern of macrophages in CS-exposed animals, are similar to observations made in humans, emphasizing the critical role of CS in these cells.
Eosinophils
Apart from neutrophils and macrophages, there is evidence that eosinophils may also play a role in COPD and that patients with eosinophilic inflammation may represent a distinct phenotype of the disease. Although eosinophilic airway inflammation is usually considered a feature of asthma, its presence in large- and small-airway tissue samples and in 20%–40% of induced sputum samples has been demonstrated in patients with stable COPD. Airway eosinophilia increases during COPD exacerbations (Citation24–26).
In guinea pigs, eosinophils were the predominant cells and were homogenously distributed in airways, pulmonary vessels and septa, and they correlated weakly with morphological changes in smaller airways and small intrapulmonary vessels. We do not rule out the possibility that the eosinophilic reaction elicited by CS exposure may be a specific feature of the guinea pig, along with its enhanced bronchial hyperresponsiveness, but the presence of eosinophilic bronchitis in smokers who are not asthmatics suggests a potential role of CS in the recruitment of eosinophils in lung structures (Citation27).
Lymphocytes
Inflammation mediated by lymphocytes in the lung is considered a key component of COPD (Citation28;Citation29). These inflammatory cells, which persist long after ceasing to smoke (Citation30), are diffusely distributed and might play an important role in the onset and maintenance of a chronic inflammatory response throughout the lung. In addition, T and B cells aggregate into organized lymphoid follicles in close proximity to the airways and within the lung parenchyma (3;6). It has been reported that the number of airways containing lymphoid follicles is increased in severe COPD (GOLD stages 3 and 4) when compared with patients in stages GOLD 0 to 2 (Citation6). We evaluated the presence of lymphoid follicles in the lungs of guinea pigs. In our study, there was an increased proportion of lungs with lymphoid follicles in animals exposed for 6 months to CS. Moreover, there was a trend towards a positive correlation between follicle size and the wall thickness of smaller airways.
Lung fibrosis
Recent studies have demonstrated areas of lung fibrosis in some smokers. Furthermore, the association of emphysema with fibrosis has been recognized as a specific entity, with a poor outcome (Citation31–33). In guinea pigs exposed to CS, we observed collagen deposition in airways and alveolar septa. Moreover, some degree of fibrosis has been observed in the airways of smokers (Citation6;Citation12;Citation34). The mechanisms of fibrosis around the airways are not yet understood, but they probably involve an attempt to repair chronic inflammation. Our results concur with those of Wright et al. (Citation14), who showed increased amounts of thick collagen fibres in the small-airway walls in a model of guinea pig exposed to CS. We also observed marked fibrosis in alveolar septa, fitting with an increase in emphysema. These septal areas could be more susceptible to damage and repair overlapping emphysematous lesions (Citation33). Interestingly, we observed airway inflammation preceded by airway remodelling, supporting the role of inflammation in lung remodelling (Citation4,Citation35,Citation36). We observed an increase in thick collagen fibres, especially in smaller airways, in contrast to thin fibres. Thick collagen fibres have been associated with scarring and could modulate the stiffness of tissues (Citation37).
The study has some limitations. Inflammatory cells were identified by a combination of histochemical staining and standard morphological criteria. Immunostaining with monoclonal antibodies would have provided more specific identification of inflammatory cells. Unfortunately, most of the commercially available antibodies are not sensitive or specific enough for immunohistochemical characterization in the guinea pig. We conducted preliminary immunohistochemical analyses with several commercially available antibodies but they lacked the specificity, selectivity and reproducibility required by our experimental conditions. Accordingly, we used standard histochemical and morphological criteria to distinguish the inflammatory cell populations.
Conclusions
In summary, our study has fully characterized a pleiotropic inflammatory reaction induced by chronic CS exposure in the airways, pulmonary vessels and alveolar septa of the guinea-pig lung. The inflammatory reaction was composed of neutrophils, macrophages and eosinophils and persisted over time, especially in smaller airways and vessels. The intensity of the neutrophilic and macrophagic infiltrate correlates with smaller airway remodelling and emphysema, emphasizing the crucial role of these cells in morphological changes associated with COPD. The remodelling of small pulmonary vessels was not associated with neutrophil and macrophage infiltration but slightly correlated with that of eosinophils.
In animals exposed to CS, areas of collagen deposition were apparent in alveolar septa and smaller airways and were associated with the enlargement of the alveolar size. Therefore, CS-induced inflammatory reaction not only leads to lung damage but also to a repair process. All in all, this indicates that the effects of CS on the guinea pig lungs mimic those observed in patients diagnosed with COPD, further supporting the use of this animal model in studies of the clinical progression of COPD and therapeutic interventions.
Declaration of Interest
The authors report that they have no conflicts of interest. The authors are responsible for the writing of this paper. This manuscript was supported by grants from the Fondo de Investigación Sanitaria (04/1424), the European Commission (2005-018725) and Consorcios Estratégicos Nacionales en Investigación Técnica (CENIT).
Notice of corrections: Corrections have been made to the captions of Figures 1-3, 5 and 6 since the original online publication of this article on 18 June, 2012.
References
- Rivera RM, Cosio MG, Ghezzo H, Salazar M, Perez-Padilla R. Comparison of lung morphology in COPD secondary to cigarette and biomass smoke. Int J Tuberc Lung Dis 2008 Aug;12(8):972–7.
- Cosio MG, Saetta M, Agusti A. Immunologic aspects of chronic obstructive pulmonary disease. N Engl J Med 2009 Jun 4;360(23):2445–54.
- van der Strate BW, Postma DS, Brandsma CA, Melgert BN, Luinge MA, Geerlings M, Hylkema MN, van den BA, Timens W, Kerstjens HA. Cigarette smoke-induced emphysema: A role for the B cell? Am J Respir Crit Care Med 2006 Apr 1;173(7):751–8.
- Turato G, Zuin R, Miniati M, Baraldo S, Rea F, Beghe B, Monti S, Formichi B, Boschetto P, Harari S, Airway inflammation in severe chronic obstructive pulmonary disease: relationship with lung function and radiologic emphysema. Am J Respir Crit Care Med 2002 Jul 1;166(1):105–10.
- Retamales I, Elliott WM, Meshi B, Coxson HO, Pare PD, Sciurba FC, Rogers RM, Hayashi S, Hogg JC. Amplification of inflammation in emphysema and its association with latent adenoviral infection. Am J Respir Crit Care Med 2001 Aug 1;164(3):469–73.
- Hogg JC, Chu F, Utokaparch S, Woods R, Elliott WM, Buzatu L, Cherniack RM, Rogers RM, Sciurba FC, Coxson HO, The nature of small-airway obstruction in chronic obstructive pulmonary disease. N Engl J Med 2004 Jun 24;350(26):2645–53.
- Cosio MG, Majo J, Cosio MG. Inflammation of the airways and lung parenchyma in COPD: role of T cells. Chest 2002 May;121(5 Suppl):160S–5S.
- Wright JL, Churg A. A model of tobacco smoke-induced airflow obstruction in the guinea pig. Chest 2002 May;121(5 Suppl):188S–91S.
- Ferrer E, Peinado VI, Diez M, Carrasco JL, Musri MM, Martinez A, Rodriguez-Roisin R, Barbera JA. Effects of cigarette smoke on endothelial function of pulmonary arteries in the guinea pig. Respir Res 2009;10:76.
- Meyerholz DK, Griffin MA, Castilow EM, Varga SM. Comparison of histochemical methods for murine eosinophil detection in an RSV vaccine-enhanced inflammation model. Toxicol Pathol 2009 Feb;37(2):249–55.
- White HJ, Garg BD. Early pulmonary response of the rat lung to inhalation of high concentration of diesel particles. J Appl Toxicol 1981 Apr;1(2):104–10.
- Kuwano K, Bosken CH, Pare PD, Bai TR, Wiggs BR, Hogg JC. Small airways dimensions in asthma and in chronic obstructive pulmonary disease. Am Rev Respir Dis 1993 Nov;148(5):1220–5.
- James AL, Hogg JC, Dunn LA, Pare PD. The use of the internal perimeter to compare airway size and to calculate smooth muscle shortening. Am Rev Respir Dis 1988 Jul;138(1):136–9.
- Wright JL, Postma DS, Kerstjens HA, Timens W, Whittaker P, Churg A. Airway remodeling in the smoke exposed guinea pig model. Inhal Toxicol 2007 Sep;19(11):915–23.
- Hsia CC, Hyde DM, Ochs M, Weibel ER. An official research policy statement of the American Thoracic Society/European Respiratory Society: standards for quantitative assessment of lung structure. Am J Respir Crit Care Med 2010 Feb 15;181(4):394–418.
- Turato G, Zuin R, Saetta M. Pathogenesis and pathology of COPD. Respiration 2001;68(2):117–28.
- Janoff A. Elastases and emphysema. Current assessment of the protease-antiprotease hypothesis. Am Rev Respir Dis 1985 Aug;132(2):417–33.
- Churg A, Wang R, Wang X, Onnervik PO, Thim K, Wright JL. Effect of an MMP-9/MMP-12 inhibitor on smoke-induced emphysema and airway remodelling in guinea pigs. Thorax 2007 Aug;62(8):706–13.
- Di SA, Turato G, Maestrelli P, Mapp CE, Ruggieri MP, Roggeri A, Boschetto P, Fabbri LM, Saetta M. Airflow limitation in chronic bronchitis is associated with T-lymphocyte and macrophage infiltration of the bronchial mucosa. Am J Respir Crit Care Med 1996 Feb;153(2):629–32.
- Churg A, Wang RD, Tai H, Wang X, Xie C, Dai J, Shapiro SD, Wright JL. Macrophage metalloelastase mediates acute cigarette smoke-induced inflammation via tumor necrosis factor-alpha release. Am J Respir Crit Care Med 2003 Apr 15;167(8):1083–9.
- Shapiro SD. The macrophage in chronic obstructive pulmonary disease. Am J Respir Crit Care Med 1999 Nov;160(5 Pt 2):S29–S32.
- Di SA, Capelli A, Lusuardi M, Balbo P, Vecchio C, Maestrelli P, Mapp CE, Fabbri LM, Donner CF, Saetta M. Severity of airflow limitation is associated with severity of airway inflammation in smokers. Am J Respir Crit Care Med 1998 Oct;158(4):1277–85.
- Finkelstein R, Fraser RS, Ghezzo H, Cosio MG. Alveolar inflammation and its relation to emphysema in smokers. Am J Respir Crit Care Med 1995 Nov;152(5 Pt 1):1666–72.
- Gorska K, Krenke R, Korczynski P, Kosciuch J, Domagala-Kulawik J, Chazan R. Eosinophilic airway inflammation in chronic obstructive pulmonary disease and asthma. J Physiol Pharmacol 2008 Dec;59 Suppl 6:261–70.
- Saetta M, Di Stefano A, Maestrelli P, Turato G, Ruggieri MP, Roggeri A, Calcagni P, Mapp CE, Ciaccia A, Fabbri LM. Airway eosinophilia in chronic bronchitis during exacerbations. Am J Respir Crit Care Med 1994 Dec;150(6 Pt 1):1646–52.
- Snoeck-Stroband JB, Lapperre TS, Gosman MM, Boezen HM, Timens W, ten Hacken NH, Sont JK, Sterk PJ, Hiemstra PS. Chronic bronchitis sub-phenotype within COPD: inflammation in sputum and biopsies. Eur.Respir.J. 2008 Jan;31(1):70–7.
- Papi A, Romagnoli M, Baraldo S, Braccioni F, Guzzinati I, Saetta M, Ciaccia A, Fabbri LM. Partial reversibility of airflow limitation and increased exhaled NO and sputum eosinophilia in chronic obstructive pulmonary disease. Am J Respir Crit Care Med 2000 Nov;162(5):1773–7.
- Saetta M, Di Stefano A, Maestrelli P, Ferraresso A, Drigo R, Potena A, Ciaccia A, Fabbri LM. Activated T-lymphocytes and macrophages in bronchial mucosa of subjects with chronic bronchitis. Am Rev Respir Dis 1993 Feb;147(2):301–6.
- Cosio MG. Autoimmunity, T-cells and STAT-4 in the pathogenesis of chronic obstructive pulmonary disease. Eur Respir J 2004 Jul;24(1):3–5.
- Gamble E, Grootendorst DC, Hattotuwa K, O'Shaughnessy T, Ram FS, Qiu Y, Zhu J, Vignola AM, Kroegel C, Morell F, Airway mucosal inflammation in COPD is similar in smokers and ex-smokers: a pooled analysis. Eur Respir J 2007 Sep;30(3):467–71.
- Cottin V, Nunes H, Brillet PY, Delaval P, Devouassoux G, Tillie-Leblond I, Israel-Biet D, Court-Fortune,Valeyre D, Cordier JF. Combined pulmonary fibrosis and emphysema: a distinct underrecognised entity. Eur Respir J 2005 Oct;26(4):586–93.
- Katzenstein AL, Mukhopadhyay S, Zanardi C, Dexter E. Clinically occult interstitial fibrosis in smokers: classification and significance of a surprisingly common finding in lobectomy specimens. Hum Pathol 2010 Mar;41(3):316–25.
- Washko GR, Hunninghake GM, Fernandez IE, Nishino M, Okajima Y, Yamashiro T, Ross JC, Estepar RS, Lynch DA, Brehm JM, Lung volumes and emphysema in smokers with interstitial lung abnormalities. N Engl J Med 2011 Mar 10;364(10):897–906.
- Kranenburg AR, Willems-Widyastuti A, Moori WJ, Sterk PJ, Alagappan VK, de Boer WI, Sharma HS. Enhanced bronchial expression of extracellular matrix proteins in chronic obstructive pulmonary disease. Am J Clin Pathol 2006 Nov;126(5):725–35.
- O'Shaughnessy TC, Ansari TW, Barnes NC, Jeffery PK. Inflammation in bronchial biopsies of subjects with chronic bronchitis: inverse relationship of CD8+ T lymphocytes with FEV1. Am J Respir Crit Care Med 1997 Mar;155(3):852–7.
- Saetta M, Di Stefano A, Turato G, Facchini FM, Corbino L, Mapp CE, Maestrelli P, Ciaccia A, Fabbri LM. CD8+ T-lymphocytes in peripheral airways of smokers with chronic obstructive pulmonary disease. Am J Respir Crit Care Med 1998 Mar;157(3 Pt 1):822–6.
- Suki B, Ito S, Stamenovic D, Lutchen KR, Ingenito EP. Biomechanics of the lung parenchyma: critical roles of collagen and mechanical forces. J Appl Physiol 2005 May;98(5):1892–9.