Abstract
Cigarette smoking is the major risk factor for chronic obstructive pulmonary disease (COPD). Specific dopamine related gene alleles have previously been found to be associated with smoking initiation, maintenance and cessation. We investigated the association between specific dopamine related gene alleles and both change in smoking behavior and lung function change over time in individuals with mild-to-moderate COPD. Subjects included a subset of participants in the Lung Health Study (LHS), a smoking intervention study in smokers with mild to moderate COPD. Smoking status was determined and lung function performed at baseline and annually for 5 years. In post-hoc analyses, we assessed the association of the dopamine receptor (DRD2) TaqI A1+ allele (A1A1, A1A2 genotypes) and A1− allele (A2A2 genotype), and the dopamine transporter (DAT) 9R+ allele (9R9R and 9R10R genotypes) and 9R− allele (10R10R genotype) with both changes in smoking status and lung function in a subset of LHS subjects. No significant associations were noted between variants in these genes and success in smoking cessation. However, in exploratory analyses that did not adjust for multiple comparisons, sustained male (but not female) quitters with the DRD2 A1− allele and/or the DAT 9R+ allele showed an accelerated decline in FEV1 similar to that of continuing smokers over 5 years after quitting smoking. These preliminary findings suggest that dopamine-related genes may play a role in the progression of COPD, at least in the subset of male ex-smokers whose disease continues to progress despite sustained quitting, and warrants additional confirmatory and mechanistic studies.
Abbreviations | ||
DRD2 | = | D2 dopamine receptor |
DAT | = | Dopamine transporter |
LHS | = | Lung Health Study |
COPD | = | Chronic obstructive pulmonary disease |
FEV1 | = | Forced expired volume in 1 second |
FVC | = | Forced vital capacity |
UC | = | Usual Care group |
SI | = | Special Intervention group |
Introduction
Cigarette smoking is the major risk factor for chronic obstructive pulmonary disease (COPD) (Citation1), a progressive disease characterized by an accelerated rate of decline in forced expired volume in 1 sec (FEV1) (Citation2). The only intervention that has been definitively shown to reduce this excessive decline in lung function is smoking cessation with sustained abstinence (Citation2–4). Despite the proven benefits of smoking cessation on the course of COPD, a large proportion of symptomatic patients with this chronic smoking-related respiratory disorder continue to smoke. These continuing smokers with COPD are believed to experience greater difficulty in quitting smoking, compared to smokers without COPD (Citation5), possibly due to their generally higher levels of nicotine dependence compared to smokers in general (Citation6). Patients with mild to moderate COPD are generally heavier smokers with a greater number of pack-years of smoking and more previously unsuccessful quit attempts compared to smokers in general (Citation7).
Based on prior research that suggests a role for the minor Taq1 A1+ allele (A1A1, A1A2 genotypes) of the D2 dopamine receptor (DRD2) gene (Citation8,9), and the 9R− allele (10R10R genotype) of the independent dopamine transporter (DAT) gene (Citation10,11) in smoking initiation, maintenance and cessation, we hypothesized that variants in these genes might predict which smokers participating in the Lung Health Study (LHS), an early intervention trial involving intensive smoking cessation and relapse prevention counseling in smokers with mild to moderate COPD, would be most likely to succeed in quitting smoking. Although we did not find any significant associations between these gene variants and smoking cessation, we did notice an association of these genes with the annual decline in lung function over a 5-year follow-up period in a subset of the LHS participants who succeeded in quitting smoking and we made these latter findings a focus of this article.
Methods
Study population
The LHS I was a 10-center randomized clinical trial of 5,887 middle-aged smokers with the objective of determining whether an intervention program combining intensive smoking cessation counseling and an inhaled anticholinergic bronchodilator could slow the rate of decline in FEV1 over a 5-year follow-up period during which subjects underwent pre- and post-bronchodilator spirometry annually (Citation3). Entry criteria included a history of current regular smoking, ≥10 pack years of smoking, age 35–60 years, the presence of mild to moderate airflow obstruction (FEV1 / forced vital capacity (FVC) ratio <70%), a baseline FEV1 of 55–90% predicted, and the absence of other significant pulmonary or other medical illness. Eligible participants were randomized to receive “usual care” (UC) or “special intervention” (SI). The SI group received a state-of-the-art, 4-month intensive smoking cessation program followed by a 5-year relapse prevention program, along with physician advice and nicotine replacement. The UC group received no intervention except for only brief counseling on entry into the study. Informed consent was obtained from all participants at each of the 10 LHS clinical centers.
The LHS subpopulation for the present study comprised 621 individuals who demonstrated the “lowest” (n = 291) or “highest” (n = 330) extremes of smoking characteristics that were believed to be predictive of successful or unsuccessful quitting, respectively, based on the number of cigarettes smoked per day at baseline and the age of initiation of regular smoking. The “lowest extreme” comprised subjects in the SI group who were in 1) the lowest quintile of intensity of smoking at study entry (as defined by number of cigarettes smoked per day), 2) the highest quintile of age of starting smoking, and 3) the lowest quintile of continuous smoking behavior during the 5-year period of the study (all were sustained quitters, i.e., were abstinent at each annual visit).
The “highest extreme” comprised subjects in the SI group who were in 1) the highest quintile of intensity of smoking at study entry (as defined by number of cigarettes smoked per day), 2) the lowest quintile of age of starting smoking, and 3) the highest quintile of continuous smoking behavior despite intensive smoking cessation counseling (all were continuous smokers, i.e., reported smoking at each annual visit). These two groups were used to test the hypothesis that DRD2 TaqI and DAT gene alleles, singly and in combination, influence a smoker's ability to quit.
DRD2 TaqI and DAT gene allele analysis
Samples of peripheral blood white blood cells were obtained from LHS participants approximately 5 years after subjects were entered into the study and were stored at a repository at the University of Minnesota. Prior to the withdrawal of blood samples, subjects gave informed consent for the use of these samples for genetic analysis in an anonymized fashion using an informed consent form that was approved by each of the 10 institutions participating in the LHS. For the present study, samples for genetic analysis were received from the repository for the 621 participants in both “extreme” categories.
Approximately 50 to 100 micrograms of DNA were extracted from stored white blood cells frozen at –80°C. The DNA was isolated and purified by a standard procedure (Citation12). The white blood cells were digested with proteinase K in the presence of the detergent Sodium Dodecyl Sulfate (SDS). This digest subsequently underwent a series of extractions with phenol/chloroform. The DNA was precipitated from the resultant aqueous solution by the addition of ethyl alcohol to 70% volume. The precipitated DNA was then pelleted by centrifugation. The resultant DNA pellet was dried and reconstituted in a Tris-EDTA buffer for use as template for PCR (Citation13).
DRD2 TaqI A genotypes were determined by a previously described PCR procedure (Citation14). In addition, the 40 bp repeat polymorphism of the DAT gene was determined by the PCR procedure described by Vandenbergh et al. (Citation15). Based on the DRD2 Taq1 A genotypes, subjects were categorized as those who carried the A1+ allele (A1A1 and A1A2 genotypes) versus those who carried the A1− allele (A2A2 genotype only). Based on the DAT genotypes, subjects were classified as those who carried the 9R+ allele (9R9R and 9R10R genotypes) versus those who carried the 9R− allele (10R10R genotype), as described by Lerman et al. (Citation10) and Sabol et al. (Citation11).
FEV1 measurements
Pre- and post-bronchodilator (isoproterenol 200 μg total dose) FEV1 was measured at baseline and annually for 5 years for all LHS 1 participants, as previously reported (Citation3). FEV1, both in absolute volume (ml) and as percent of predicted for age, height, sex and race (Citation16) and bronchodilator responsiveness at baseline as percent increase in FEV1 after administration of isoproterenol over the prebronchodilator value were recorded. Post-bronchodilator FEV1 was used for analysis because the bronchodilator was expected to minimize inter- and within-subject variability caused by differences in airway responsiveness to internal and/or environmental factors.
At a separate visit, airway responsiveness to increasing doses of methacholine was determined at baseline (Citation17) and expressed as a 2-point slope, as described by O'Connor et al. (Citation18). The latter has been shown to be a predictor of the subsequent annual rate of decline in FEV1 (Citation17). Analyses were also performed separately for men and women since gender differences in the impact of changes in smoking status on lung function were observed in LHS I that could not be entirely adjusted by using gender-specific prediction equations (Citation19).
Smoking status
Self-reported smoking status was assessed at baseline and each annual visit and verified by end-expired CO measurements and salivary cotinine levels (Citation3).
Statistical analysis
Sustained quitters and continuing smokers over the 5-year trial duration were compared on selected baseline demographic, smoking and physiologic characteristics using a two-sample t-test for continuous variables and Chi square for the proportions of sustained quitters vs. continuing smokers who exhibited each DRD2 A1− allele and DAT 9R+ allele pattern. A mixed-effects model was used to analyze the effect of genotype on FEV1 over the 5 years of follow-up in sustained quitters and continuing smokers separately (Citation20). The dependent variable in all models was post-bronchodilator FEV1.
Independent variables included one of the genetic markers along with baseline values of age, FEV1 as percent of predicted, cigarettes per day and the two-point methacholine slope (Citation18). Separate analyses were performed for DRD2, DAT and a combination of DRD2 and DAT (coded “1” when either A1+ or 9R− was present, “2” when both were present, “0” when neither was present) and separately for men and women. Genetic markers were represented in two ways in all models: 1) as a main effect that tested differences in mean FEV1 over the 5 years between allele groups, and 2) as an interaction term between genetic marker and the year of the follow-up visit (as an ordered random effect) that represented the effect of the genetic markers on the annual rate of change in percent predicted FEV1. No adjustments were made for the multiple comparisons between genetic markers in the different subsets of subjects analyzed; therefore, all p values must be considered nominal.
A separate analysis was performed comparing the slopes (± SEM) of the post-bronchodilator FEV1 from year 1 to year 5 between individual subjects exhibiting the different allele patterns (DRD 2 A1+ vs. A1−; DAT 9R− vs. 9R+; and neither DRD2 A1+ nor DAT 9R− vs. both of these alleles vs only one of these alleles) in quitters and continuing smokers for men and women separately. Means of the slopes were compared between groups by unpaired t-test. Again, no adjustment was made for multiple comparisons, so that all p-values listed are nominal.
Statistical analysis was performed using SAS/PC version 8.1. (SAS Institute Inc., SAS Campus Drive, Cary, North Carolina 27513, USA).
Results
Baseline characteristics of the sustained quitters and continuing smokers are presented in . For both men and women, compared to the continuing smokers, the sustained quitters were slightly older, had initiated smoking at an older age and smoked fewer cigarettes per day at baseline, while methacholine responsiveness was similar between the two groups. For the men only, the sustained quitters had a slightly higher FEV1% predicted at baseline. For both men and women, the proportions of those with the DRD2 A1+, DRD2 A1−, DAT 9R+, and DAT 9R− alleles were similar between the sustained quitters and the continuing smokers.
Table 1. Characteristics of male and female sustained quitters and continuing smokers.
DRD2 and DAT alleles and lung function
From the mixed model analysis, for all of the changes in lung function analyzed (changes from baseline to year 5 or from year 1 to year 5 in pre- and post-bronchodilator FEV1 expressed in ml or as percent predicted), significant differences were found in male sustained quitters with differing DRD2 alleles (A1+ vs. A1−), DAT alleles (9R− vs. 9R+) and a combination of DRD2 and DAT alleles (A1+9R− vs. A1+9R+ vs. A1−9R− and A1−9R+). On the other hand, no differences were apparent in female sustained quitters nor in male or female continuing smokers.
In and , results of the mixed model analysis are shown only for adjusted post-bronchodilator FEV1 and the change in post-bronchodilator FEV1 from year 1 to year 5 (as reflected by the interaction terms between genetic marker and year) for men and women, respectively. Among continuing smokers, no significant differences in FEV1 were found between allele groups either for FEV1 or for the rate of decline in FEV1 through the 5-year follow-up time in either men or women.
Table 2a. Post-bronchodilator FEV1 over year 1 through year 5; results of mixed model analysis (males).
Table 2b. Post-bronchodilator FEV1 over year 1 through year 5; results of mixed model analysis (females).
Females who quit smoking in the first year of the study and sustained their non-smoking status throughout the follow-up period (“sustained quitters”) also did not demonstrate any significant differences in FEV1 between allele groups for either FEV1 or rate of change in FEV1. On the other hand, among male sustained quitters statistically significant differences in the rate of change in FEV1 from year 1 through year 5 were found, as indicated by the significant interaction between genetic maker and year ().
The annual changes in FEV1 in the male sustained quitters can be visualized in , which displays mean values (± SEM) of FEV1 at each annual visit from year 1 to year 5 stratified by the allele pattern as derived from the mixed model analysis. The FEV1 of male sustained quitters with the A1− or 9R+ allele declined significantly compared to those with the A1+ or 9R− allele, respectively ( and 1B). Furthermore, when participants were classified according to the combination of DRD2 and DAT alleles, those with both the A1− and 9R+ alleles showed a significant decline in FEV1 during the follow-up period compared to those with neither of these alleles ().
Figure 1. Predicted FEV1 (± SEM) from the mixed model at Year 1 to Year 5 in male sustained quitters stratified by the DRD2 A1+ or the DRD2 A1- allele (A), the DAT 9R+ or the DAT 9R- allele (B) and the combination of both the DRD2 A1- and DAT 9R+ or neither the DRD2 A1- nor the DAT 9R+ allele (C).
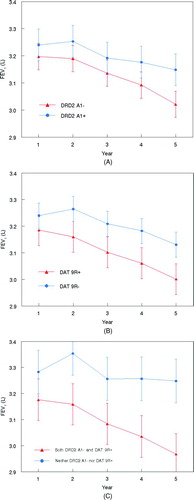
In a separate analysis, slopes of the percent predicted post-bronchodilator FEV1 by DRD2 allele (A1+ or A1−), DAT allele (9R− or 9R+) or the combination of DRD2 and DAT alleles (A1−/9R+ and A1+/9R−) were calculated for male and female quitters and continuing smokers separately (). For male quitters only, significant differences in the slopes of FEV1% predicted decline were found between those with the DRD2 A1+ vs. A1− allele and the DAT 9R− vs. 9R+ allele, the slopes being significantly more negative in those with the A1− or 9R+ allele. Moreover, those with both the DRD2 A1− and DAT R9+ alleles showed significantly greater declines in FEV1 than those with neither of these alleles, the latter having a positive slope of FEV1 change over time.
Table 3. Mean slopes (± SEM) of change in FEV1% predicted per year from Year 1 through Year 5 by DRD2 and DAT alleles and combinations thereof, gender and smoking status.
For illustrative purposes, observed% predicted FEV1 values from baseline to year 5 are shown in for the 183 male sustained quitters. From baseline to year 1, following smoking cessation early in the year, a slight improvement in% predicted FEV1 was observed in the entire group of subjects, irrespective of allele pattern. However, from year 1 to the subsequent years of the study, a progressive deterioration in lung function was found in the subgroup of quitters with the DRD2 A1− allele (), the DAT 9R+ allele () and the combination of DRD2 A1− and DAT 9R+ alleles ().
Figure 2. Observed percent predicted FEV1 (± SEM) at baseline and Year 1 to Year 5 in male sustained quitters stratified by the DRD2 A1+ or the DRD2 A1- allele (A), the DAT 9R+ or the DAT 9R- allele (B) and the combination of both the DRD2 A1- and DAT 9R+ or neither the DRD2 A1- nor the DAT 9R+ allele (C).
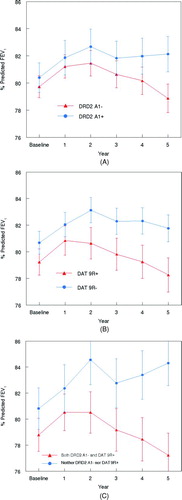
In contrast, lung function from year 1 to year 5 revealed relatively little deterioration or even a slight improvement in the subgroup of quitters with the DRD2 A1+ allele (), the DAT 9R− allele () and the combination of DRD2 A1+ and DAT 9R− alleles ().
Discussion
This study yielded interesting and unexpected findings in well-characterized smokers with mild-to-moderate COPD, who were carefully followed in the Lung Health Study for 5 years with a high rate of study completion. First, we failed to observe any association between dopamine receptor and transporter gene variants and the success or lack of success in achieving smoking cessation and sustained abstinence in smokers with either a low or high likelihood of success based on baseline smoking characteristics. These findings were unexpected in view of previous evidence, suggesting that specific dopamine receptor and transporter gene variants play a role in smoking initiation, maintenance and cessation (9–11,14).
Second, and more interestingly, we found that male (but not female) sustained quitters with the DRD2 A1−, the DAT 9R+ and a combination of DRD2 A1− and DAT 9R+ alleles showed an accelerated decline in lung function compared to the sustained quitters with the opposite allele pattern. The latter group of male sustained quitters (those with the DRD2 A1+ allele, the DAT 9R− allele and combination of DRD2 A1+ and DAT 9R− alleles) showed no change or a small improvement in their lung function from the 1st to the 5th annual visit, while in the former group (those with the DRD2 A1−, DAT 9R+ or A1−/9R+ combination) an accelerated rate of decline was observed that was closer to what was observed among the continuing smokers over the same time period. No association between these allele patterns and the rate of decline in lung function was observed in female sustained quitters or in continuing smokers of either sex.
Study limitations
A major limitation of the present study is that the analysis of the association between the different dopamine allele patterns and the rate of decline in FEV1 was post-hoc and did not adjust for the multiple comparisons that were made within separate subgroups of participants (sustained quitters and continuing smokers and men and women), so that our findings must be considered exploratory.
Another limitation is that DNA from only 621 (10.5%) of the total of 5887 participants in the LHS was analyzed. The reason we limited our study to these 621 individuals is that they comprised the “extreme” quintiles representing polar opposites with respect to the likelihood of success versus failure in stopping smoking and remaining abstinent consistent with the initial objective of our study.
This strategy was anticipated to provide us with adequate statistical power for demonstrating a significant association between the dopamine alleles of interest and smoking cessation if such an association existed. However, the end result of our analysis was a failure to demonstrate a significant difference in successful smoking cessation between these two extreme subgroups. At the same time, on the other hand, an unanticipated and potentially important finding was a possible association that merits further investigation between the dopamine alleles we examined and the rate of decline in FEV1 in a subset of these 621 individuals whose DNA we analyzed.
Although it would be of interest to analyze the DNA from the other participants in the LHS who were not included in our study we are unable to do so due to a lack of sufficient remaining DNA from most LHS participants, underscoring the need for further studies to confirm or refute these findings, considering their implications with respect to the potential influence of dopaminergic pathways on the course of COPD.
Rate of FEV1 decline in COPD and candidate genes
COPD progression is believed to result from inflammation that persists in some patients well after smoking cessation has occurred (Citation21). Other factors, such as genetic susceptibility, also play a role in COPD progression. In a recent review article, Silverman et al. (Citation22) summarized significant genetic associations with rapid decline in lung function in the Lung Health Study. The following candidate genes were found to show significant associations (attributable risk ranging from 3 to 10%): α1-ATZ (heterozygosity for the Z allele of α1 antitrypsin); mEH (microsomal epoxide hydrolase); β2AR (beta 2 adrenergic receptor); IL1RN A (interleukin 1 receptor antagonist); IL8 (interleukin 8); IL6 (interleukin 6); MMP-1 (matrix metalloproteinase 1); IL4R (interleukin 4 receptor); glutathione transferases (GSTP1, GSTT1 and GSTM1); heme oxygenase-1; catalase; and the nicotine receptor (CHRNA5) [see from Silverman et al. (Citation22)].
As noted by Silverman et al., other investigators have confirmed the contributions of microsomal epoxide hydrolase, heme oxygenase-1, and the glutathione S-transferases and have also implicated ADAM 33, cytochrome P450, and glutamate cysteine ligase. In addition to these genes, Hersh (Citation23) has also referenced GC (group-specific component of serum globulin 1F allele), CDC6 and LEPR as genes that have been shown to be associated with lung function decline in COPD; interestingly, a polymorphism in the CDC6 gene was found to be associated with decline in lung function in ex-smokers (Citation24). Polymorphisms in the DRD2 and DAT genes have not previously been studied in relation to progression of COPD.
Role of dopamine in the lung
There is some evidence for a direct or indirect role of dopamine in the lung. Activation of D2 dopamine receptors in the periphery can modulate neuronal activity in an inhibitory manner (Citation25). D2 dopamine receptors are present in the lung (Citation26) and reduce the ability of vagal sensory nerves to produce reflex mediated tachypnea that could influence the sensation of dyspnea (Citation27). In addition, D2 dopamine mRNA has been demonstrated in the ganglia of sensory nerves associated with reflex pathways (Citation28).
Further, dopamine receptor agonists have been shown to inhibit depolarization of the rat and human vagus (Citation29) and may thereby modulate vagally mediated COPD symptoms, including cough and increased mucus secretion, as well as lung function. Moreover, stimulation of D2 dopamine receptors present on sensory nerves in the lung is capable of suppressing reflex-mediated COPD symptoms (Citation30). On the other hand, to our knowledge, dopaminergic mechanisms have not thus far been demonstrated to play a role in the pathobiology of smoking-related lung injury or repair.
The results of our study suggest that variants in DRD2 and DAT genes may influence the progression or stabilization of COPD in male subjects who have been successful at maintaining smoking cessation for 5 years since male sustained quitters with the DRD2 A1− allele, DAT 9R+ allele and combination of DRD2 A1− and DAT 9R+ alleles had substantial declines in lung function when compared to the male quitters with the DRD2 A1+ allele, the DAT 9R− alleles and combination of DRD2 A1+ and DAT 9R− alleles, who showed either an improvement or only a minimal decline in lung function. However, these genetic profiles had no influence on progression of COPD in the continuing smokers of either gender, who exhibited an excessive decline in FEV1 with age irrespective of their DRD2 and DAT 9R allelic pattern.
Comparison of COPD in women and men has suggested various gender differences, including a potential difference in susceptibility to cigarette smoke (Citation31) and expression of the emphysema versus airways disease phenotype (Citation32,33), as well as differences in response to smoking cessation and relapse (Citation19). In this regard, it is interesting that we observed gender differences, favoring males over females, in the effect of the dopamine gene (DRD2, DAT) alleles on the course of FEV1 decline among sustained quitters with COPD.
There is support for a gender effect in associations of dopamine gene (DRD2, DAT) alleles with other disorders. Associations of the DRD2 A1 allele (A1A1, A2A2 genotype) with alcohol dependence (Citation34,35) and with smoking behavior (Citation36) has been found to be limited to males. The DAT 9R− allele (10R10R genotype), but not the 9R+ allele (9R9R, 9R10R genotype), was found to be a protective factor for male Parkinson's Disease (PD) patients but not for female PD patients (Citation37).
There is growing evidence of in vivo gender differences in D2− dopamine receptor characteristics. In a PET study, women had higher D2− dopamine binding potentials than men in the frontal cortex (Citation38) and lower D2− dopamine binding potentials in striatal regions (Citation39,40). It is suggested (Citation34, Citation38) that these differences may be reflected in gender-associated differences found in clinical disorders as indicated here and as we suggest in the present study.
A question may be raised as to whether a therapeutic intervention can be applied to mitigate the declining lung function in male DRD2 A1− allele (A2A2 genotypes) subjects who have ceased to smoke. Studies have shown that DRD2 A1− allele (A2A2 genotype) subjects have a higher number of brain D2 dopamine receptors than DRD2 A1+ allele (A1A1, A2A2 genotypes) subjects (Citation41–44). It is noteworthy that several studies have shown the DRD2 A1− allele (A2A2 genotype) to be associated with schizophrenia (for review see Citation45).
Further, D2 dopamine receptor antagonists are widely and successfully used to ameliorate symptoms of schizophrenia. Could the administration of D2 dopamine receptor antagonists block the decline in lung function in male subjects with the DRD2 A1− allele (A2A2 gentotype) who have ceased smoking? Future studies may be needed to address this issue.
Conclusions
We observed that specific dopaminergic gene variants are associated with excessive decline in lung function among male ex-smokers with COPD, suggesting that genes involved in the dopaminergic pathway might possibly influence the development and course of COPD. The mechanism of this association and how any such influence might interact with smoking behavior and gender is unknown and warrants investigation.
Declaration of Interests
None of the authors has any financial, consulting or personal relationships with other people or organizations that could influence or bias the authors’ work. The authors did not obtain any scientific writing assistance. This work was supported in part by contract N01-HR-46002 from the Division of Lung Diseases of the National Heart, Lung and Blood Institute.
References
- Davis RM, Novotny TE. The epidemiology of cigarette smoking and its impact on chronic obstructive pulmonary disease. Am Rev Respir Dis 1989; 140(3 Pt 2):S82–84.
- Fletcher C, Peto R. The natural history of chronic airflow obstruction. Br Med J 1977; 1(6077):1645–1648.
- Anthonisen NR, Connett JE, Kiley JP, Effects of smoking intervention and the use of an inhaled anticholinergic bronchodilator on the rate of decline of FEV1 –The lung health study. JAMA 1994; 272: 1497–1505.
- Scanlon PD, Connett JE, Waller L, Smoking cessation and lung function in mild-to-moderate chronic obstructive pulmonary disease: The Lung Health Study. Am J Respir Crit Care Med 2000; 161:381–390.
- Pride NB. Smoking cessation: effects on symptoms, spirometry and future trends in COPD. Thorax 2001; 56 (Suppl 2): ii7–10.
- Jiménez-Ruiz CA, Masa F, Miravitlles M, Smoking characteristics: differences in attitudes and dependence between healthy smokers and smokers with COPD. Chest 2001; 119:1365–1370.
- Buist AS, Connett JE, Miller RD, Chronic Obstructive Pulmonary Disease Early Intervention Trial (Lung Health Study): Baseline characteristics of randomized participants. Chest 1993; 103:1863–1872.
- Noble EP, St. Jeor ST, Ritchie T, D2 dopamine receptor gene and cigarette smoking: A reward gene? Medical Hypotheses 1994; 42:257–260.
- Noble EP. D2 dopamine receptor gene in psychiatric and neurologic disorders and its phenotypes: a review. Am J Med Genet (Neuropsychiatric Genetics) 2003; 116:103–125.
- Lerman C, Caporaso NE, Audrain J, Evidence suggesting the role of specific genetic factors in cigarette smoking. Health Psychol 1999; 18:14–20.
- Sabol SZ, Nelson ML, Fisher C, A genetic association for cigarette smoking behavior. Health Psychol 1999; 18:7–13.
- Old JM, Davies KE. Prenatal diagnosis of Duchenne muscular dystrophy by DNA analysis. J Med Genet, 1986; 23: 556–559.
- Saiki RK, Gelfand DH, Stoffer S, Primer-directed enzymatic amplification of DNA with a thermostable DNA polymerase. Science 1988; 239:487–491.
- Noble EP, Noble RE, Ritchie T, D2 dopamine receptor gene and obesity. Int J Eat Disord 1994;15:205–17.
- Vandenbergh DJ, Persico Am, Hawkins AL, Human dopamine transporter gene (DAT1) maps to chromosome 5p15.3 and displays a VNTR. Genomics 1992; 14:1104–1106.
- Crapo RD, Morris AH, Gardner RM. Reference spirometric values using techniques and equipment that meet ATS recommendations. Am Rev Respir Dis 1981; 123:659–664.
- Tashkin DP, Altose MD, Connett JE, Methacholine reactivity predicts changes in lung function over time in smokers with early COPD. Am J Respir Crit Care Med 1996; 153:1802–1811.
- O'Connor GT, Sparrow D, Weiss ST. A prospective longitudinal study of methacholine airway responsiveness as a predictor of pulmonary function decline: The Normative Aging Study. Am J Respir Crit Care Med 1995; 152:87–92.
- Connett JE, Murray RP, Buist AS, Changes in smoking status affect women more than men: Results of the Lung Health Study. Am J Epidem 2003; 157:973–979.
- Laird NM, Ware JH. Random effects models for longitudinal data. Biometrics 1982; 32:963–974.
- Hogg JC, Chu F, Utokaparch S, The nature of small airway obstruction in chronic obstructive pulmonary disease. N Engl J Med; 2004; 350:2645–2653.
- Silverman EK, Spira A, Pare PD. Genetics and genomics of chronic obstructive pulmonary disease. Proc Am Thorac Soc 2009; 6:539–542.
- Hersh CP. Pharmacogenetics of chronic obstructive pulmonary disease: challenges and opportunities. Pharmacogenomics 2010; 11:237–247.
- Takabatake N, Toriyama S, Igarashi A, A novel polymorphism in CDC6 is associated with the decline in lung function of ex-smokers in COPD. Biochem Biophys Res Commun 2009; 38:554–559.
- Missale C, Nash SR, Robinson SW. Dopamine receptors: from structure to function. Physiol Rev 1998; 78:189–225.
- Bruzzone P, D'Andrea V, Motta C, Occurrence of dopaminergic (D(2)) receptros within the rabbit pulmonary circulation. Pulm Pharmacol Ther 2001; 15:393–398.
- Jackson DM, Simpson WT. The effect of dopamine on the rapidly adapting receptors in the dog lung. Pulm Pharmacol Ther 2000; 13:39–42.
- Xie GX, Jones K, Peroutka SJ, Detection of mRNAs and alternatively spliced transcripts of dopamine receptors in rat peripheral sensory and sympathetic ganglia. Brain Res 1998; 785:129–135.
- Bierell MA, Crispino N, Hele DJ, Effect of dopamine receptor agonists on sensory nerve activity: possible therapeutic targets for the treatment of asthma and COPD. Br J Pharmacol 2002; 136:620–628.
- Bonnert RV, Brown RC, Chapman D, Dual D2-receptor and beta2-adrenoceptor agonists for the treatment of airway diseases. 1. Discovery and biological evaluation of some 7-(2-aminoethy)-4-hydroxybenzothiasol-2(3H)-one analogues. J Med Chem 1998; 41:4915–4917.
- Chen Y, Horne SL, Dosman JA. Increased susceptibility to lung dysfunction in female smokers. Am Rev Respir Dis 1991; 143:1224–1230.
- Dransfield MT, Washko GR, Foreman MG, Gender differences in the severity of CT emphysema in COPD. Chest 2007; 132:464–470.
- Martinez FJ, Curtis JL, Sciurba F, Sex differences in severe pulmonary emphysema. Am J Respir Crit Care Med 2007; 176: 243–252.
- Limosin, F, Gorwood P, Loze J-Y, Dubertet C, Gouya L, Deybach J-C, Adès J. Male limited association of the dopamine D2 gene Taq1A polymorphism and alcohol dependence. Am J Med Genet 2002; 112: 343–346.
- Lopez-Castroman J, Vaquero-Lorenzo C, Mercedez Perez-Rodriguez M, Gender effect on association between DRD2 polymorphism and substance dependence in a Spanish sample. Drug Alcohol Depend 2009; 101: 210–212.
- Munafo MR, Timpson NJ, David SP, Association of the DRD2 geneTaq1A polymorphism and smoking behavior: a meta-analysis and new data. Nicotine Tobacco Res 2009; 11: 64–76.
- Lin J-J, Yueh K-C, Chang D-C, The homozygote 10-copy genotype of variable number tandem repeat dopamine transporter gene may confer protection against Parkinson's disease for male, but not to female patients. J Neurol Sci 2003; 209: 87–92.
- Kaasinen V, Nagren K, Hietala J, Sex differences in extrastriatal dopamine D2− like receptors in the human brain. Am J Psych 2001; 158: 308–311.
- Farde L, Hall H, Pauli S, Variability in D2− dopamine receptor density and affinity; a PET study with (κC) raclopride in man. Synapse 1995; 20:200–208.
- Pohjalainen T, Rinne J, Nagren K, Sex differences in striatal dopamine D2 receptor binding characteristics in vivo. Am J Psych 1998; 155:768–773.
- Noble EP, Blum K, Ritchie T, Allelic association of the D2 dopamine receptor gene with receptor binding characteristics in alcoholism. Arch Gen Psych 1991; 48:648–654.
- Thompson J, Thomas N, Singleton A, D2 dopamine receptor gene (DRD2) Taq1A polymorphism: reduced dopamine D2 receptor binding in the human striatum associated with the A1 allele. Pharmacogenetics 1997; 7:479–484.
- Pohjalainen T, Rinne JO, Nagren K, The A1 allele of the human D2 dopamine receptor gene predicts low D2 receptor availability in healthy volunteers. Mol Psych 1998; 3:256–260.
- Jonsson EG, Nothen MM, Grunhage F, Polymorphisms in the dopamine D2 receptor gene and their relationships to striatal dopamine receptor density of healthy volunteers. Mol Psych 1999; 4:290–296.
- Ponce G, Perez-Gonzalez R, Aragues M, The ANKK1 Kinase Gene and psychiatric disorders. Neurotox Res 2009; 16:50–59.