Abstract
Introduction: Decreased airway distensibility (AD) in response to deep inspirations, as assessed by HRCT, has been associated with the severity of asthma and COPD. Aims: The current study was designed to compare the magnitude of AD by HRCT in individuals with asthma and COPD with comparable degrees of bronchial obstruction, and to explore factors that may influence it. Results: We enrolled a total of 12 asthmatics (M/F:7/5) and 8 COPD (7/1) with comparable degree of bronchial obstruction (FEV1% predicted mean±SEM: 69.1 ± 5.2% and 61.2 ± 5.0%, respectively; p = 0.31). Each subject underwent chest HRCT at FRC and at TLC. A total of 701 airways (range 20 to 38 airway per subject; 2.0 to 23.1 mm in diameter) were analyzed. AD did not differ between asthmatics and COPD (mean ± SEM: 14 ± 3.5% and 17 ± 4.3%, respectively; p = 0.58). In asthmatics, AD was significantly associated with FEV1% predicted (r2 = 0.45, p = 0.018). We found a significant correlation between the change in lung volume and the change in AD by HRCT (r2 = 0.64, p = 0.002). In COPD, we found significant correlations between AD and the RV% predicted (r2 = 0.51, p = 0.046) and the RV/TLC (r2 = 0.68, p = 0.01). Conclusions: AD was primarily affected by the dynamic ability to change lung volumes in asthmatics, and by static lung volumes in COPD.
Keywords: :
Introduction
In asthma and COPD, the functional alterations can be defined not only by the degree of bronchial obstruction but also by the magnitude of the dilatory response of the airways to deep inspirations (DIs). DIs exert their effects on airways through the radial traction that is applied to the airway wall by virtue of the interdependence between intraparenchymal airways and the surrounding parenchyma. Radial traction is sustained by a network of alveolar attachments to the airway walls: thus, any factors altering the airways and the parenchyma can cause a reduction in the distending forces leading to a reduced ability of DIs to bronchodilate (Citation1). Increased thickness of the airway wall, such as that observed in the more severe stages of asthma (Citation2, 3), or the loss of alveolar attachments, such as that documented in the emphysematous lungs (Citation4, 5), can impair the ability to distend airways. Both in asthmatics (Citation6, 7) and in COPD (Citation8, 9), we found that the severity of the disease, as assessed by lung function impairment, was associated with the decreasing bronchodilatory ability of DI.
DIs exert their effects on airways through the radial traction that is applied to the airway wall by virtue of the interdependence between intraparenchymal airways and the surrounding parenchyma. Radial traction is sustained by a network of alveolar attachments to the airway walls: thus, any factors altering the airways and the parenchyma can cause a reduction in the distending forces leading to a reduced ability of DIs to bronchodilate. Increased thickness of the airway wall, such as that observed in the more severe stages of asthma, or the loss of alveolar attachments, such as that documented in the emphysematous lungs, can impair the ability to distend airways. Scichilone et al. (Citation4), and Corsico et al. (Citation5), demonstrated that the number of destroyed alveolar attachments is correlated with blunted DI-induced bronchodilation in COPD subjects who had undergone surgical resection of lung parenchyma. However, the need of obtaining lung tissue severly limits the possibility of implementing research activities aimed at understanding the mechanisms involved in the pathogenesis of bronchial obstruction.
High resolution computed tomography (HRCT) has been used to obtain non-invasive assessment of airway measurements and parenchymal density in individuals with obstructive pulmonary diseases (Citation10–13). We hypothesized that the mechanism for decreased bronchodilatory ability of DIs would vary by the etiology of the disease. The current study was designed to determine which factors affected the magnitude of DI-induced AD as measured by HRCT in asthmatic and COPD subjects with comparable degree of bronchial obstruction.
Methods
Subjects
We enrolled unselected consecutive individuals who fulfilled the diagnostic criteria for either asthma (Citation14) or COPD (Citation15), respectively. Patients visiting the Outpatient Clinic for Respiratory Diseases of the University of Palermo, Italy, were asked to participate in the study. All the enrolled subjects were clinically stable at the time of the study. Asthmatics had partially or totally controlled disease, as assessed by the Asthma Control Test (ACT) at the time of the recruitment (ACT > 20 in all subjects). Before each study visit, all subjects were asked to abstain from inhaled, short-acting β-agonists or anticholinergic agents for at least 12 hours, or long-acting bronchodilators for at least 24 hours. The protocol was approved by the Ethics Committee of the University of Palermo, Palermo, Italy, and written informed consents were obtained.
The study was performed on 2 days. The first visit included clinical and functional assessments. On a separate occasion, within 7 days, the lung imaging was carried out. By design, all subjects were in stable clinical conditions at both study days.
Study design
Clinical and functional assessments
Clinical and demographic data included age, sex, duration of disease, frequency of exacerbations in the previous year, and current treatment. Functional assessment was made of static and dynamic lung volumes using the helium dilution technique and a computerized water-sealed spirometer (Biomedin; Padua, Italy). Measurements were made in accordance to the European Thoracic Society standardisation of lung volume measurements (Citation16).
Imaging assessment
Each subject underwent HRCT evaluation, which was performed by spiral computed tomography (multidetector 64 channels, Philips Medical System, Cleveland, OH), with a setting of 120 kVp, 200 mAs, a 0.9-mm slice thickness, a rotation time 0.5 s, and a reconstruction interval of 0.45 mm, pitch 0.923, during a single breath and moved caudally. Images were reconstructed at a window level of −600 Hounsfield units (HU) and a window width of 1600 HU. Two sets of scans were obtained: the first one was acquired at the end of the expiratory phase of the tidal volume (presumably functional residual capacity, FRC) and the second one at maximal lung inspiration (presumably total lung capacity, TLC). Subjects were trained to perform these maneuvers immediately prior to the scanning procedure. Any portion of the scan with motion artifact from breathing was discarded. We also made sure that the time course of the inspiration and the expiration phases were equal in all subjects, since it is known that this can affect the lung volume history (Citation17).
The change in airway diameter measured at TLC and at FRC (airway distensibility, AD) represented the main outcome of the study. Airway wall fraction (AWF, airway wall thickness as a fraction of the airway diameter) and lung density were measured to evaluate which radiological abnormalities potentially affected the main outcome. The occurrence of diffuse fibrosis of the lung and/or other abnormalities (parenchymal infiltrates, cystic lesions) potentially affecting the density of the lung represented an exclusion criterion. The validated Pulmonary Workstation 2 software package (VIDA Diagnostics, Inc, Iowa City, IA) was used (Citation18–20).
Airway measurements
AD was measured by applying the following formula to each individual airway:
In addition, we arbitrarily divided the airways into three groups by size: small (< 4 mm in airway diameter), medium (4–8 mm in airway diameter) and large (>8 mm in airway diameter), and AD was expressed for each airway size group.
Parenchymal measurements
measured total lung volume and parenchymal density on the HRCT scans as an estimate of parenchymal damage. Lung total volume was calculated automatically and expressed in cm3. Parenchymal density was expressed by lung attenuation parameters, such as mean lung density at FRC (Mean HUexp) and at TLC (Mean HUinsp), the percent of the lung with a low attenuation area at TLC below -950 HU (LAA-950) as an estimate of the degree of emphysema, and the percent of the lung with low attenuation area at FRC below −856 (RA–856) as an estimate of the degree of air trapping.
Data Analysis
Data analysis was performed using JMP 9.0.1 software (SAS Institute Inc., North Carolina). Wilcoxon sign rank tests were performed for paired comparisons, simple linear regressions, and step-wise linear regression where appropriate. Results are expressed as mean ± SEM. Significance level was set at p ≤ 0.05.
Results
A total of 12 asthmatics (M/F: 7/5) and 8 COPD (M/F: 7/1) were studied. Demographic and clinical lung function characteristics of the study subjects are presented in . By design, the magnitude of airway obstruction, as assessed by FEV1% predicted and FEV1/FVC%, was not different between the asthmatic and COPD subjects. FVC% predicted was significantly lower in COPD (p = 0.04). As expected, the diffusing capacity for CO was significantly lower in COPD group than in asthmatic group (p = 0.03). HRCT measured lung volumes and lung attenuations did not differ between groups ().
Table 1. Demographic and lung function characteristics of the study subjects
Table 2. HRCT measured lung volumes and lung attenuations in asthmatics and COPD subjects
A total of 701 airways (range 20 to 38 airway per subject; 2.0 to 23.1 mm in diameter) were analyzed. We did not find a significant difference neither in the mean AWF between groups (at TLC: p = 0.44; at FRC: p = 0.40), nor when airways were examined by size (p = 0.25, p = 0.40, p = 0.35 for small, medium and large airways, respectively).
The AD by airway size was similar between the two groups (). In addition, the HRCT-change in lung volume with inspiration from FRC to TLC did not differ between the asthmatic (40 ± 8%) and COPD (42 ± 10%) groups (p = 0.70). In the asthmatic group, AD significantly correlated with FEV1% predicted (r2 = 0.45, p = 0.018) (), but not with FVC% predicted (r2 = 0.23, p = 0.11), or FEV1/FVC (r2 = 0.24, p = 0.10). In addition, AD did not correlate with TLC% predicted (r2 = 0.02, p = 0.63), RV% predicted (r2 = 0.22, p = 0.12), or RV/TLC (r2 = 0.07, p = 0.40). Finally, no correlation was found between AD and DLCO% predicted (r2 = 0.02, p = 0.67). We also found a significant correlation between the change in lung volume as measured by HRCT (to the 1/3 power) and the change in AD (r2 = 0.64, p = 0.002, ).
Figure 1. Correlation between baseline lung function expressed as FEV1% predicted and the degree of airways distensibility by deep inspirations in the asthmatic group (r2 = 0.45, p = 0.018).
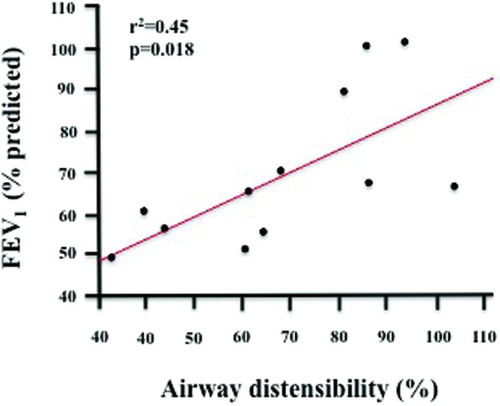
Figure 2. Correlation between the change in lung volume as measured by HRCT (to the 1/3 power) and the change in airways distensibility by deep inspirations in the asthmatic group (r2 = 0.64, p = 0.002).
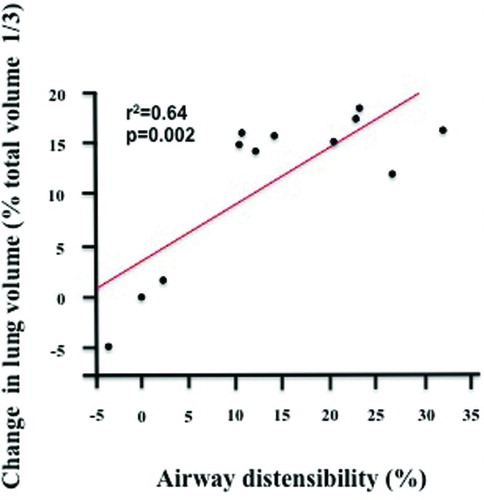
Table 3. Airway distensibility by size in asthmatics and COPD subjects
In the COPD group, no relationships were found between AD and FEV1% predicted (r2 = 0.01, p = 0.81), FVC% predicted (r2 = 0.05, p = 0.59), FEV1/FVC (r2 = 0.01, p = 0.88), TLC% predicted (r2 = 0.01, p = 0.93), or DLCO% predicted (r2 = 0.01, p = 0.79). However, significant correlations were found with RV% predicted (r2 = 0.51, p = 0.046) and RV/TLC (r2 = 0.68, p = 0.01, ). We found no relationship between the change in lung volume (to the 1/3 power) and the change in AD (r2 = 0.41, p = 0.08). Finally, AD was inversely correlated with the mean airway diameter at FRC (r2 = 0.58, p = 0.03).
Figure 3. Correlation between baseline lung function expressed as RV/TLC% predicted and the degree of airways distensibility by deep inspirations in the COPD group (r2 = 0.68, p = 0.01).
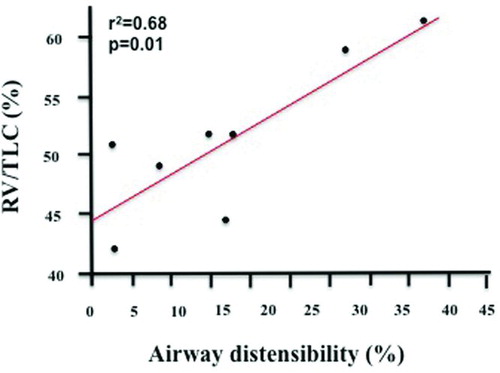
Next, we performed stepwise multiple linear regression analyses, and made two separate models for the asthmatic and the COPD subjects. For the asthmatic subjects, the variables that entered in the model were the RV% predicted (p = 0.03), the percent change in lung volume (p = 0.0009), and the mean airway diameter at TLC (p = 0.004). This model accounted for 88% of the variance in AD (r2 = 0.88, p = 0.0004). For the COPD subjects, the variables that entered in the model were the TLC% predicted (p = 0.02), and the RV/TLC (p = 0.008), the greater the RV/TLC the greater the AD. Also, RV% predicted entered the model and after correcting for the TLC and RV/TLC was in the anticipated negative direction, the greater the RV%, the lower was the AD. However, it did not reach statistical significance (p = 0.057). This model accounted for 94% of the variance in AD (r = 0.94, p = 0.0056).
Discussion
The present study was designed with the objective of evaluating the magnitude of AD by HRCT in individuals with asthma and COPD with similar degrees of airway obstruction. We also explored the factors that may influence AD in our study populations. Our primary finding was that the mean AD either overall or by airway size did not differ between the asthmatic and COPD subjects. However, the major determinants of AD were different for the two groups. In the asthmatics, the dynamic ability to change lung volume was the main factor that affected AD, yet in the subjects with COPD, the static lung volumes positively influenced the degree of AD, although the latter association appears to be counterintuitive.
Our finding of a lack of differences in AD between two different chronic respiratory diseases, asthma and COPD, is attractive and deserves some speculation. Studies incorporating the HRCT methodology to assess changes in airway diameter were commonly performed in asthmatics and in COPD separately (Citation1, Citation6, Citation8). In our study, using a multiple-regression model, we found that the AD in the asthmatic group was inversely correlated with the RV% predicted and was directly dependent on chances in lung volumes and the maximum size of the airways at TLC, which is consistent with our initial hypothesis. Whether by simple linear regression or by stepwise multiple regression, the change in lung volume was the most significant factor with regards to AD in the asthmatics. We infer from these results that for asthmatic individuals, AD was predominantly dependent on the change in lung volume. Indeed, one of the factors that influence the magnitude of the distending forces is the extent to which the lungs can inflate. In the presence of lung hyperinflation, a common state in moderate-to-severe airway obstruction in asthma, further lung inflation by DI may be impaired.
Contrary to the logical concept that increased thickness of the airway wall, such as that observed in the more severe stages of asthma, should induce greater stiffness of airways, which in turn would oppose airway distension, we were not able to demonstrate an association between airway wall thickness and AD. This observation is in line with the findings of Pyrgos et al. (Citation6). Thus, while airway walls have been shown to be thicker (Citation1), this increased thickness does not appear to impede AD. We infer from these findings and previous work (Citation6), that the remodeling processes in asthma that increase the airway wall thickness do not appear to change the basic force-tension dynamics in vivo, and do not appear to make airways more resistant to imposed stretch by DI.
In the COPD subjects, by simple linear regression, RV/TLC alone accounted for 82% of the variance in AD (r = 0.82, p = 0.01), and remained a significant independent predictor of AD in the stepwise regression model. However, it remains curious that this was a positive correlation, i.e. the greater the RV/TLC, the greater was the AD. This at first appears to be counterintuitive. While the correlation for air trapping as measured by the RA-856 was in the “right” direction (negative: the greater the air trapping, the less was the distensibility) the correlation was not significant (r = −0.45, p = 0.27). There are several possibilities for this counterintuitive finding. Brown et al. using a forced oscillation technique found that approximately 80% of total AD occurred between 75% TLC and TLC (Citation21). Therefore, as RV/TLC increases, RV increases greater than TLC increases, and the increase in TLC leads to an increase in lung volume, and thus AD will be on the steeper part of the distensibility versus lung volume portion of the curve (Citation21).
Another possibility may be mechanical. With increased RV/TLC in the subjects with COPD, the increased lung volumes may allow increased radial traction from the reduced numbers of parenchymal attachments that distend the airways with further inspiration. It is also important to note that the range of RV/TLC values for the COPD subjects were limited (range: 42 to 61; ). How AD would respond in individuals with COPD with more extreme values of RV/TLC will need to be examined. Another possible mechanism for the increased distensibility with increased RV/TLC may be that the airway smooth muscle has adapted to a new L0 from being in a chronically distended state (Citation22). The airways could be on a new passive length-tension curve due to the chronic hyperinflation in COPD.
In addition, in contrast to the asthmatic subjects, where dynamic factors were associated with AD, in the COPD subjects, static factors were associated with the AD, specifically the TLC% predicted and the RV/TLC ratio entered the stepwise model and were statistically correlated with the AD in the multiple regression model. While the relationship between the change in lung volume and AD was in the right direction it did not reach statistical significance in the simple linear regression model (r = 0.64, p = 0.08) and did not enter the stepwise regression model.
The study has some limitations. The relatively small number of subjects could potentially account for the lack of differences in terms of AD between groups. We envision this as a pilot study to determine which factors can affect the magnitude of AD in asthmatic and COPD subjects with comparable degrees of bronchial obstruction. In addition, the AD has been used as primary outcome in previous studies with similar study group size (Citation6, Citation8). Although the emphysema score was approximately 50% greater in the COPD compared to the asthma subjects, the emphysema scores, the air trapping scores, and the airway wall thicknesses were not statistically different between asthmatic and COPD subjects. There are some possible explanations for these findings. The most plausible explanation that can be advocated is that our COPD subjects were randomly selected for not showing extensive emphysematous abnormalities of the lungs.
This could account for the lack of differences in the emphysema score, and likely in the amount of air trapping. The possibility also exists that that air trapping may not be distinguishable from emphysema-associated changes at low lung volume. Similarly, the condition of hyperinflation in the asthmatic subjects may not be distinguishable from emphysema-associated changes in the COPD subjects at high lung volume on HRCT. These features could limit the ability of HRCT to discriminate between the two study groups.
In summary, our findings demonstrate that the extent of AD is similar in asthmatic and COPD individuals with a similar level of airway obstruction. While hyperinflation as measured by increased RV limits AD in asthma, it appears to favor distensibility in COPD. In asthmatic individuals, the reduction in AD was related to the degree of obstruction as measured by the FEV1, the greater the obstruction the greater was the loss of AD. Although the reduction in AD in individuals with COPD may be more acute, with the loss of parenchymal attachments reaching a critical level which leads to loss of AD. Thus, we infer from our results that the etiology of the reduced airway distensibility was disease specific. Therefore, treatment of the loss of bronchodilation due to reduced AD will require different approaches in asthmatic and COPD individuals.
Declaration of Interest
The authors report no conflicts of interest. The authors alone are responsible for the content and writing of the paper.
References
- Brown RH, Scichilone N, Mudge B, at al. High-resolution computed tomographic evaluation of airway distensibility and the effects of lung inflation on airway caliber in healthy subjects and individuals with asthma. Am J Respir Crit Care Med 2001; 163:994–1001.
- David H, Broide MB ChB. Immunologic and inflammatory mechanisms that drive asthma progression to remodeling. J Allergy Clin Immunol 2008; 121(3):560–572.
- Brightling CE, Gupta S, Gonem S, Lung damage and airway remodelling in severe asthma. Clin Exp Allergy 2012; 42(5):638–649.
- Scichilone N, Bruno A, Marchese R, Association between reduced bronchodilatory effect of deep inspiration and loss of alveolar attachments. Respir Res 2005; 6:55.
- Corsico A, Milanese M, Baraldo S, Small airway morphology and lung function in the transition from normality to chronic airway obstruction. J Appl Physiol 2003; 95:441–447.
- Pyrgos G, Scichilone N, Togias A, Bronchodilation response to deep inspirations in asthma is dependent on airway distensibility and air trapping. J Appl Physiol 2011; 110:472–479.
- Scichilone N, Marchese R, Soresi S, Deep inspiration-induced changes in lung volume decrease with severity of asthma. Respir Med 2007; 101:951–956.
- Scichilone N, La Sala A, Bellia M, The airway response to deep inspirations decreases with COPD severity and is associated with airway distensibility assessed by computed tomography. J Appl Physiol 2008; 105:832–838.
- Scichilone N, Marchese R, Catalano F, Bronchodilatory effect of deep inspiration is absent in subjects with mild COPD. Chest 2004; 125:2029–2035.
- Little SA, Sproule MW, Cowan MD, High resolution computed tomographic assessment of airway wall thickness in chronic asthma: reproducibility and relationship with lung function and severity. Thorax 2002; 57(3):247–53.
- Gupta S, Siddiqui S, Haldar P, Qualitative analysis of high-resolution CT scans in severe asthma. Chest 2009; 136(6):1521–1528.
- Aysola RS, Hoffman EA, Gierada D, Airway remodeling measured by multidetector CT is increased in severe asthma and correlates with pathology. Chest 2008; 134(6):1183–1191.
- Gupta S, Siddiqui S, Haldar P, Quantitative analysis of high-resolution computed tomography scans in severe asthma subphenotypes. Thorax 2010; 65(9):775–781.
- Global Initiative for Asthma. Global strategy for asthma management and prevention. Revised 2011. http://www.ginaasthma.com
- Global Initiative for Obstructive Lung Disease. Global strategy for the diagnosis, management and prevention of chronic obstructive pulmonary disease (updated 2010) 2011. Available from: http://www.goldcopd.org/guidelines-global-strategy-for-diagnosis-management.html
- Wanger J, Clausen JL, Coates A, Standardisation of the measurement of lung volumes. Eur Respir J 2005; 26:511–522.
- Santus P, Pecchiari M, Carlucci P, Bronchodilation test in COPD: effect of inspiratory manoeuvre preceding forced expiration. Eur Respir J 2003; 21(1):82–85.
- Tschirren J, McLennan G, Palagyi K, Matching and anatomical labeling of human airway tree. IEEE Trans Med Imaging 2005; 24:1540–1547.
- Tschirren J, Hoffman EA, McLennan G, Intrathoracic airway trees: segmentation and airway morphology analysis from low-dose CT scans. IEEE Trans Med Imaging 2005; 24:1529–1539.
- Tschirren J, Hoffman EA, McLennan G, Segmentation and quantitative analysis of intrathoracic airway trees from computed tomography images. Proc Am Thorac Soc 2005; 2:484-487, 503–504.
- Brown NJ, Salome CM, Berend N, Airway distensibility in adults with asthma and healthy adults, measured by forced oscillation technique. Am J Respir Crit Care Med 2007; 176:129–137.
- Gunst SJ, Wu MF. Selected contribution: plasticity of airway smooth muscle stiffness and extensibility: role of length-adaptive mechanisms. J Appl Physiol 2001; 90:741–749.25.