Abstract
Background: Abnormalities of autonomic function have been reported in patients with chronic obstructive pulmonary disease. The effect of the exercise training in heart rate recovery (HRR) has not been established in patients with COPD. Objective: To assess the effects of 8-weeks’ endurance training program on parasympathetic nervous system response measured as heart rate recovery in a sample of moderate-to-severe COPD patients. Methods: We recruited a consecutive sample of patients with COPD candidates to participate in a pulmonary rehabilitation program from respiratory outpatient clinics of a tertiary hospital. HRR was calculated, before and after training, as the difference in heart rate between end-exercise and one minute thereafter (HRR1) in a constant-work rate protocol. Results: A total of 73 COPD patients were included: mean (SD) age 66 (8) years, median (P25-P75) post-bronchodilator FEV1 39 (29–53)%. The prevalence of slow HRR1 (≤12 beats) at baseline was 63%, and was associated with spirometric severity (mean FEV1 35% in slow HRR1 vs 53 in normal HRR1, p < 0.001). After 8-weeks training, HRR1 improved from mean (SD) 10 (7) to 12 (7) beats (p = 0.0127). Multivariate linear regression models showed that the only variable related to post-training HRR1 was pre-training HRR1 (p < 0.001). Conclusions: These results suggest that training enhances HRR in patients with moderate-to-severe COPD. HRR is an easy tool to evaluate ANS such that it may be a useful clinical marker of parasympathetic nervous system response in patients with COPD.
Introduction
The Autonomic Nervous System (ANS) is responsible in several physiological processes. ANS plays an important role in the cardiovascular response to aerobic exercise. The parasympathetic nervous system is related with conservation of energy and is responsible to reduce both heart rate and blood pressure after their post-acute exercise increase (Citation1, 2). Heart rate recovery (HRR) after exercise reflects changes in ANS tone that occur immediately after exercise cessation (Citation3). Abnormalities of autonomic function have been reported in patients with chronic obstructive pulmonary disease (COPD) (Citation4–8) and in other chronic conditions such as chronic heart failure (Citation9), sleep apnea (Citation10), diabetes (Citation11), and obesity (Citation12). Although the causes of autonomic abnormalities still remain unsettled, it has been reported that regular aerobic training increases parasympathetic nervous system response in healthy population (Citation1, Citation13). Moreover, there is some evidence that endurance training improves autonomic function measured as HRR in patients with cardiovascular disease (Citation14–16).
Research in COPD regarding the effects of exercise training on autonomic nervous system is scarce. Costes et al. (Citation17) reported that a program of exercise training in COPD patients improved spontaneous baroreflex sensitivity, a method of autonomic function assessment. Borghi-Silva et al. (Citation18) also showed beneficial effects on ANS following an endurance training program in a cohort of patients with COPD, as compared to usual-care COPD patients, in terms of heart rate variability. However, both heart rate variability and baroreflex sensitivity are measures that require specific equipment and/or complex tests to assess ANS function. HRR immediately after exercise has been proposed as an easy and responsive measure of parasympathetic nervous system response in the clinical practice (Citation19–21). Moreover, it has also been proposed as a marker of endurance training effects (Citation22). In addition, HRR is an important clinical marker since it relates to exercise capacity (Citation23) and to mortality not only in the general population (Citation2, Citation23, Citation24) also in patients with cardiovascular disease (Citation25) and patients with COPD (Citation5).
The aim of the present study was to assess the effects of endurance training on the parasympathetic nervous system response in patients with COPD. Our contention was that the exercise program could improve the parasympathetic nervous response measured as HRR in patients with moderate-to-severe COPD.
Methods
Study design
This was a prospective experimental study. All patients participated in an 8-weeks training program, and all measurements were done before and after training.
Subjects
A consecutive sample of COPD patients from the respiratory outpatient clinics of a tertiary hospital, candidates to participate in a rehabilitation program from October 2007 to October 2010 were approached to participate in this study. Inclusion criteria were: a) diagnosis of COPD according to the spirometric criteria by the Global Initiative for Chronic Obstructive Lung Disease (GOLD) (Citation26); b) being clinically stable at the time of study, without episodes of exacerbation or oral steroid treatment in the previous 6 weeks; c) referring compliance with therapy; and, d) no participation in a rehabilitation program for at least 12 months prior to study initiation. Exclusion criteria were: a) coexistence of severe chronic disease states, such as cardiovascular, musculo-skeletal, and neurological disorders precluding performance of exercise tests; and b) diagnosis of any type of cancer. The Human Ethics Committee of the Hospital Clinic de Barcelona (Spain) approved the study and informed consent was signed by all subjects.
Measurements
The main measure before and after the training program was HRR after constant-work rate cycle ergometry test (Citation27) at 80% of pre-training peak work-load (Wpeak). After 2 minutes breathing at rest, followed by 1 minute pedaling unloaded, the work rate was increased until reaching 80% of pre-training Wpeak. The subjects were asked to pedal as long as possible against the constant load, until exhaustion. Pulmonary gas exchange and ventilatory measurements were obtained from calibrated signals derived from rapid response gas analyzers (CardiO2 cycle Medical Graphics Corporation, St. Paul, MN, USA) and a mass flow sensor. The following variables were recorded breath by breath: pulmonary oxygen uptake (VO2), pulmonary carbon dioxide output (VCO2), respiratory exchange ratio (RER), minute ventilation (VE), tidal volume (VT), and respiratory rate (RR). Heart rate (HR) was determined using 3 lead on-line electrocardiogram and oxygen saturation by pulse oximetry (SaO2). The endurance time (ET) was registered using an analogical timer.
The HRR was defined as the difference in heart rate between the end of exercise and one minute unloading thereafter (HRR1). In order to describe the population, pre-training HRR1 was divided in slow (≤12 beats) or normal (>12 beats), according to a previous study (Citation23), in which this cut-off value maximized the log-rank test to determine abnormal values, and was related to the risk of mortality in healthy adults. The percentage of chronotropic response (CR) was calculated as follows: CR = ((end exercise HR –resting HR)/(220 –age –resting HR)) × 100 (Citation28). Given the importance of reducing errors in HR measurement, this outcome was obtained from the monitor of the electrocardiogram and recorded using Medical Graphics Corporation software. Quality control also included checking HR data for each patient through comparison of recorded value with the paper report registered during the cycle ergometry.
In addition to a clinical history and physical examination, the following tests were included: (i) pulmonary function tests (MasterScreen; Jaeger, Wüerzburg, Germany) (Citation29, 30) and arterial blood gases (Ciba Corning 800, USA), providing data about post-bronchodilator forced expiratory volume in the first second (FEV1), inspiratory capacity to total lung capacity ratio (IC/TLC), and arterial oxygen and carbon dioxide partial pressures (PaO2, PaCO2); (ii) standard incremental cycling exercise test (Citation27); (iii) 6-minute walking test (6MWT) (Citation31); (iv) body mass index (BMI) and body composition analysis estimating fat free mass index (FFMI) by bioelectrical impedance analysis (Quantum X, RJL Systems instruments, Mi, USA); and, (v) routine blood investigations including C-reactive protein (CRP). All these measurements were performed in the morning and at two days differing less than one week. Patients were allowed to take their regular medication, included inhaled therapy.
Training program
Patients with COPD exercised on a cycle ergometer (Jaeger ER 550; Wüerzburg, Germany) three days per week for eight weeks. Training sessions with cycle ergometer, of one hour duration, consisted of: (i) warm-up: 5 minutes of cycling at 30% Wpeak; (ii) exercise: 40 minutes cycling of interval training combining 2 minutes at high work rate, 70–100% Wpeak, with 3 minutes at 40–50% Wpeak; followed by (iii) cold-down consisting of 5 minutes cycling at 30% Wpeak. The rate of pedalling during the sessions was kept at 60–70 rpm. The progress of work rate during the training period was decided on an individual basis to maximize the training effect, using a Borg score of 4 to 6 for dyspnea or fatigue as a target (Citation32). During the first 2 weeks of the program, cycling at high work rate was set at least 60–70% Wpeak. Thereafter, it was increased by 5% every week up to a maximum of 100% of pre-training Wpeak during the last 2 weeks of the training program. SaO2 and HR were continuously monitored (Pulsox-300i, Konica Minolta, Osaka, Japan) during the training sessions. Moreover, lower limb fatigue and dyspnea were assessed using the Borg scale. In addition, 2 out of 3 days per week they also trained upper limbs strength.
Statistical analysis
Sample size estimations using GRANMO 5.2 (Citation33), suggested that 73 patients would allow us to recognize as statistically significant a difference ≥4 units between the before and after training HRR1 values, assuming HRR1 standard deviation to be 9 (Citation5), accepting an alpha risk of 0.05 and a beta risk of 0.20 in a two-sided test. Anticipating a drop-out rate of 40%, we estimated a minimum of 67 patients to approach.
Baseline characteristics of the sample are presented as mean (SD), median (P25-75) and n (%), according to the distribution of each variable, and compared between slow and normal HRR1 by means of paired Student's t, Wilcoxon, Chi2, or Fisher's exact tests. The effects of the intervention on HRR1 were measured by comparing its distribution before and after training using paired Student's t-test. To test whether the effects of the training program on HRR1 differed according to individual characteristics, we built multivariate lineal regression models with HRR1 after training as the outcome, adjusted for HRR1 before training, and including other relevant individual characteristic as covariates.
These individual characteristics covered: age, FEV1, IC/TLC, PaO2, PaCO2, diabetes, arterial systemic hypertension, chronic heart failure, obesity, hypercholesterolemia, baseline resting heart rate, baseline Watts peak, baseline VO2 peak, baseline VE peak, and baseline heart rate peak. These variables were included in the final model if they were related to both the exposure and the outcome, or modified (>10% change in the coefficient) the estimates for the remaining variables. Statistical analyses were performed using Stata statistical software program (version 10.1; Stata Corp LP; College Station, Texas, USA). In all tests, statistical significance was set at a p-value lower than 0.05.
Results
A total of 121 patients were identified as eligible. From them, 11 patients did not accept to participate in the study, 28 patients did not finish the rehabilitation program, and 9 patients were excluded from the analysis because of presence of cardiac arrhythmias or technical problems during HRR1 measurement. Thus, the total number of participants completing the study was 73 patients. There were no differences between participants and non-participants in age (66 vs 65 years, p = 0.332), gender (90% vs 86% men, p = 0.436), smoking habit (21% vs 38% current smokers, p = 0.074), or FEV1 (41% vs 37%, p = 0.485), respectively.
The main characteristics of the 73 participants are displayed in : 92% men, mean age 66 (8) years, 20% current smokers, mean BMI 27.9 (5.2) kg/m2, and median (P25-P75) post-bronchodilator FEV1 39 (29–53)%. All patients were on fixed combination inhaled therapy, and under treatment for their corresponding co-morbid conditions if present. shows the distribution of HRR1 values across GOLD stages. The prevalence of slow HRR1 (≤12 beats) before training (baseline) was 63%, and was associated with COPD severity: 30%, 64%, and 86% in GOLD stages 2, 3 and 4, respectively (p < 0.001). Overall, patients with slow HRR1 presented poor clinical and functional status (Tables and ).
Table 1. Baseline anthropometric and functional characteristics of 73 COPD subjects included in the pulmonary rehabilitation program, according to baseline (pre-training) HRR1 status
Table 2. Baseline exercise tolerance characteristics of 73 COPD subjects included in the pulmonary rehabilitation program, according to baseline (pre-training) HRR1 status
After 8 weeks of the endurance training program, patients significantly improved exercise capacity so that endurance time increased from mean (SD) 282 (131) to 713 (627) seconds (p < 0.001). Similarly, HRR1 improved from 10 (7) to 12 (7) beats (p = 0.0127) measured in constant-work test (), which corresponds to a median increase of 14% with respect to baseline HRR1. There were no differences in HRR1 at baseline or in changes in HRR1 between patients with co-morbidities and/or in antihypertensive therapy or treatments of left ventricular failure. Moreover, there were no differences in response to the training program according to baseline HRR1 groups except for HRR1 improvement, which was higher in subjects with slow HRR1 at baseline ().
Figure 2. Mean and 95% CI of HRR1 before and after 8-weeks of endurance training in 73 COPD patients.
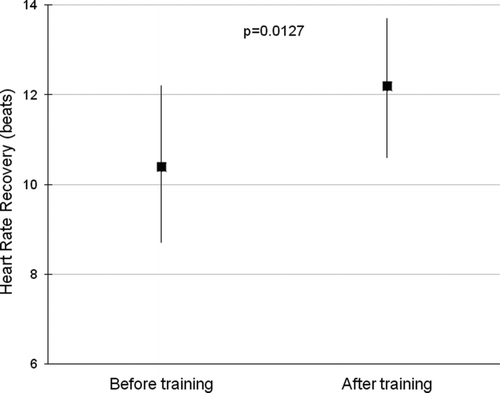
Table 3. Changes in exercise tolerance characteristics of 73 COPD subjects after 8-weeks of pulmonary rehabilitation, according to baseline (pre-training) HRR1 status
Baseline HRR1 was associated with post-training HRR1 in a linear regression model (coefficient: change of 0.6 beats in post-training HRR1, for each beat of baseline HRR1, p < 0.001). Multivariate linear regression models showed that, after adjustment for baseline HRR1, no association was observed between any of the potential covariates (age, FEV1, IC/TLC, PaO2, PaCO2, diabetes, arterial systemic hypertension, chronic heart failure, obesity, hypercholesterolemia, baseline resting heart rate, baseline Watts peak, baseline VO2 peak, baseline VE peak, baseline heart rate peak, changes in VE/VO2, or changes in VE/VCO2) and post-training HRR1.
Discussion
Our study shows that regular exercise training improves parasympathetic nervous system response measured as HRR1 after constant-work rate exercise test using cycle ergometer at 80% of pre-training Wpeak in patients with COPD. In addition, and after considering demographic, clinical and functional variables, baseline heart rate recovery was the only variable able to predict training-induced changes in heart rate recovery.
These results are in agreement with previous studies. Borghi-Silva et al. (Citation18) and Camillo et al. (Citation34) reported the effects of the endurance training on autonomic nervous function measured as heart rate variability in patients with COPD enrolled in exercise training program. Costes et al. (Citation17) also showed the beneficial influence of exercise training on spontaneous cardiac baroreflex sensitivity in patients with COPD. These measurements, however, are sophisticated and need complex calculations for their clinical interpretation. Consequently, Lacasse et al. (Citation5) proposed the HRR assessment as an easy marker to evaluate parasympathetic nervous function in COPD patients. Similar results were obtained by Georgiopoulou et al. (Citation35) in a small sample of moderate COPD patients after 36-session exercise-based cardiopulmonary program. The current study confirms the acceptability of HRR as an easy, rapid and responsive tool to evaluate the effect of the exercise training on parasympathetic nervous response in a larger sample and more severe patients with COPD.
We found that HRR1 was related to FEV1, but FEV1 itself did not influence the effects of training on HRR1. This is in agreement with previous data showing that slow HRR is more prevalent in patients with very severe COPD (Citation5, Citation36). In contrast, Camillo et al. (Citation4) stated that ANS function (measured as heart rate variability) was not associated to COPD severity in 31 patients, but correlation coefficients were not shown in the manuscript. In agreement with previous studies, we found an association between low PaO2 values and slow HRR1 at baseline (Citation6, Citation37). We also observed an association between baseline HRR1 status and systemic inflammation measured by CRP. That is, those patients with slow HRR1 presented higher levels of CRP.
It is of note that our study provides the first evidence of an association between low-grade chronic systemic inflammation and ANS function in COPD patients. This is supported by the knowledge that parasympathetic activity inhibits the inflammatory response (Citation38), and akin to a previous study (Citation39) that reported a significant relationship between acute inflammation and deterioration of HRR1 in healthy subjects. All things considered, more research should be warranted about ANS function in COPD patients. Moreover, COPD recommendations and guidelines should consider the inclusion of ANS function as a valuable clinical outcome (Citation8).
It could be argued why HRR was measured after submaximal rather than maximal exercise. Actually, the influence of the exercise protocol and the impact of work-rate on HRR remain unsettled. Cole et al. showed that HRR was a strong predictor of mortality when measured at either maximal (Citation23) or submaximal (Citation24) exercise protocols. However, Pierpont et al. (Citation20), based on a detailed study of HR decay curves over 5 minutes, suggested that the use of HRR after submaximal exercise was acceptable, while assessment of HRR after peak exercise would be inadequate due to inconsistent decay curves at peak work rate. Our findings of changes in HRR1 after submaximal exercise using cycle ergometry agree with this previous literature.
It could be argued if a training-induced mean change of 2 beats in HRR1 has any clinical implication. A recent study in patients with myocardial infarction showed very similar results (the training-induced change in mean HRR was from 17.5 beats at baseline to 19 beats after training, p = 0.011) (Citation40) to the current study. The same investigation found that a slow HRR after training (defined as <12 bpm) was independently related to a higher risk of cardiac mortality (Citation40). Altogether, our data suggest that the interrelationships between parasympathetic nervous system response, exercise training and clinical outcomes, deserve further investigations in patients with COPD or similar chronic diseases.
Study limitations
Our study has some shortcomings. Firstly, our investigation does not include a control group. However, the evidence that endurance training improves other measurements of parasympathetic nervous system in COPD patients supports that our observed changes can be attributed to the training program rather than a placebo-like effect. In addition, our opinion is that HRR is not expected to change in a period of only 8-weeks in stable COPD patients (also stable regarding their co-morbidities) without other pathophysiological changes or drug/non-drug interventions. Secondly, the possibility of selection bias was discarded given the lack of differences between patients who participated in the study and those who did not. The inclusion of patients with co-morbidities is considered strength of the present study since it ensured external validity, a factor that was missing in previous studies.
Conclusions
We conclude that endurance training enhances HRR, a marker of parasympathetic nervous system response, in moderate-to-severe COPD patients. HRR is easy to perform in a conventional training program, and it can be a useful clinical marker of ANS function in these patients.
Declaration of Interest Statement
None of the authors has a conflict of interest. The authors are responsible for the writing and the content of this paper.
Acknowledgments
We wish to thank C. Gistau, M. Simó, M. Palomo, B. Valeiro and F. Burgos from the Pulmonary Function Laboratory of Hospital Clinic of Barcelona for their support and technical assistance during this study.
References
- Freeman JV, Dewey FE, Hadley DM, Autonomic nervous system interaction with the cardiovascular system during exercise. Prog Cardiovasc Dis 2006; 48(5):342–362.
- Hautala AJ, Karjalainen J, Kiviniemi AM, Physical activity and heart rate variability measured simultaneously during waking hours. Am J Physiol Heart Circ Physiol 2010; 298(3):H874–H880.
- Lahiri MK, Kannankeril PJ, Goldberger JJ. Assessment of autonomic function in cardiovascular disease: physiological basis and prognostic implications. J Am Coll Cardiol 2008; 51(18):1725–1733.
- Camillo CA, Pitta F, Possani HV, Heart rate variability and disease characteristics in patients with COPD. Lung 2008; 186(6):393–401.
- Lacasse M, Maltais F, Poirier P, Post-exercise heart rate recovery and mortality in chronic obstructive pulmonary disease. Respir Med 2005; 99(7):877–886.
- Stewart AG, Waterhouse JC, Howard P. Cardiovascular autonomic nerve function in patients with hypoxaemic chronic obstructive pulmonary disease. Eur Respir J 1991; 4(10):1207–1214.
- Stewart AG, Waterhouse JC, Howard P. The QTc interval, autonomic neuropathy and mortality in hypoxaemic COPD. Respir Med 1995; 89(2):79–84.
- Volterrani M, Scalvini S, Mazzuero G, Decreased heart rate variability in patients with chronic obstructive pulmonary disease. Chest 1994; 106(5):1432–1437.
- Nolan J, Batin PD, Andrews R, Prospective study of heart rate variability and mortality in chronic heart failure: results of the United Kingdom heart failure evaluation and assessment of risk trial (UK-heart). Circulation 1998; 98(15):1510–1516.
- Wiklund U, Olofsson BO, Franklin K, Autonomic cardiovascular regulation in patients with obstructive sleep apnoea: a study based on spectral analysis of heart rate variability. Clin Physiol 2000; 20(3):234–241.
- Poirier P, Bogaty P, Philippon F, Preclinical diabetic cardiomyopathy: relation of left ventricular diastolic dysfunction to cardiac autonomic neuropathy in men with uncomplicated well-controlled type 2 diabetes. Metabolism 2003; 52(8):1056–1061.
- Poirier P, Hernandez TL, Weil KM, Impact of diet-induced weight loss on the cardiac autonomic nervous system in severe obesity. Obes Res 2003; 11(9):1040–1047.
- Imai K, Sato H, Hori M, Vagally mediated heart rate recovery after exercise is accelerated in athletes but blunted in patients with chronic heart failure. J Am Coll Cardiol 1994; 24(6):1529–1535.
- Kligfield P, McCormick A, Chai A, Effect of age and gender on heart rate recovery after submaximal exercise during cardiac rehabilitation in patients with angina pectoris, recent acute myocardial infarction, or coronary bypass surgery. Am J Cardiol 2003; 92(5):600–603.
- La Rovere MT, Mortara A, Sandrone G, Autonomic nervous system adaptations to short-term exercise training. Chest 1992; 101(5 Suppl):299S–303S.
- Dimopoulos S, Anastasiou-Nana M, Sakellariou D, Effects of exercise rehabilitation program on heart rate recovery in patients with chronic heart failure. Eur J Cardiovasc Prev Rehabil 2006; 13(1):67–73.
- Costes F, Roche F, Pichot V, Influence of exercise training on cardiac baroreflex sensitivity in patients with COPD. Eur Respir J 2004; 23(3):396–401.
- Borghi-Silva A, Arena R, Castello V, Aerobic exercise training improves autonomic nervous control in patients with COPD. Respir Med 2009; 103(10):1503–1510.
- Lauer MS. Autonomic function and prognosis. Cleve Clin J Med 2009; 76 Suppl 2S18–S22.
- Pierpont GL, Stolpman DR, Gornick CC. Heart rate recovery post-exercise as an index of parasympathetic activity. J Auton Nerv Syst 2000; 80(3):169–174.
- Shetler K, Marcus R, Froelicher VF, Heart rate recovery: validation and methodologic issues. J Am Coll Cardiol 2001; 38(7):1980–1987.
- Borresen J and Lambert MI. Autonomic control of heart rate during and after exercise : measurements and implications for monitoring training status. Sports Med 2008; 38(8):633–646.
- Cole CR, Blackstone EH, Pashkow FJ, Heart-rate recovery immediately after exercise as a predictor of mortality. N Engl J Med 1999; 341(18):1351–1357.
- Cole CR, Foody JM, Blackstone EH, Heart rate recovery after submaximal exercise testing as a predictor of mortality in a cardiovascularly healthy cohort. Ann Intern Med 2000; 132(7):552-555.
- Jolly MA, Brennan DM, Cho L. Impact of exercise on heart rate recovery. Circulation 2011; 124(14):1520–1526.
- Rabe KF, Hurd S, Anzueto A, Global strategy for the diagnosis, management, and prevention of chronic obstructive pulmonary disease: GOLD executive summary. Am J Respir Crit Care Med 2007; 176(6):532–555.
- Roca J, Rabinovich R. Clinical exercise testing. In: Lung Function Testing. Eur Respir Mon 2005; 31:146–165.
- Lauer MS, Okin PM, Larson MG, Impaired heart rate response to graded exercise. Prognostic implications of chronotropic incompetence in the Framingham Heart Study. Circulation 1996; 93(8):1520–1526.
- Roca J, Burgos F, Sunyer J, References values for forced spirometry. Group of the European Community Respiratory Health Survey. Eur Respir J 1998; 11(6):1354–1362.
- Roca J, Burgos F, Barbera JA, Prediction equations for plethysmographic lung volumes. Respir Med 1998; 92(3):454–460.
- ATS statement: guidelines for the six-minute walk test. Am J Respir Crit Care Med 2002; 166(1):111–117.
- Nici L, Donner C, Wouters E, American Thoracic Society/European Respiratory Society statement on pulmonary rehabilitation. Am J Respir Crit Care Med 2006; 173(12):1390–1413.
- Marrugat J, Vila J, Pavesi M, [Estimation of the sample size in clinical and epidemiological investigations]. Med Clin (Barc ) 1998; 111(7):267–276.
- Camillo CA, Laburu VM, Goncalves NS, Improvement of heart rate variability after exercise training and its predictors in COPD. Respir Med 2011; 105(7):1054–1062.
- Georgiopoulou VV, Dimopoulos S, Sakellariou D, Cardiopulmonary rehabilitation enhances heart rate recovery in patients with COPD. Respir Care 2012; 57(12):2095–2103.
- Seshadri N, Gildea TR, McCarthy K, Association of an abnormal exercise heart rate recovery with pulmonary function abnormalities. Chest 2004; 125(4):1286–1291.
- Ba A, Delliaux S, Bregeon F, Post-exercise heart rate recovery in healthy, obeses, and COPD subjects: relationships with blood lactic acid and PaO2 levels. Clin Res Cardiol 2009; 98(1):52–58.
- Tracey KJ. The inflammatory reflex. Nature 2002; 420(6917):853–859.
- Jae SY, Heffernan KS, Park SH, Does an acute inflammatory response temporarily attenuate parasympathetic reactivation? Clin Auton Res 2010; 20(4):229–233.
- Hai JJ, Siu CW, Ho HH, Relationship between changes in heart rate recovery after cardiac rehabilitation on cardiovascular mortality in patients with myocardial infarction. Heart Rhythm 2010; 7(7):929–936.