Abstract
Background: Viral respiratory tract infections are implicated in the pathogenesis of chronic obstructive pulmonary disease (COPD). In lung tissue specimens from patients with stable, mild COPD and from control smokers without airflow obstruction, we determined the prevalence and load of nucleic acid from common respiratory viruses and concomitant inflammation of small airways measuring less than 2-mm in diameter. Methods: Frozen lung tissue obtained from patients with stable, mild COPD (n = 20) and control subjects (n = 20) underwent real-time quantitative PCR (qPCR) for 13 respiratory viruses, and quantitative histology for inflammation of small airways. The two groups were compared for viral prevalence and load, and airway inflammation. The relationship between viral load and airway inflammatory cells was also analyzed. Results: Viral nucleic acid were detected in lung tissue of 18/40 (45.0%) of the individuals studied and included seven co-infections that were characterized by a “dominant virus” contributing to most of the total measured viral load. Lung tissue of COPD patients had a significantly higher prevalence of viral nucleic acid (particularly influenza A virus), and increased inflammation of small airways by macrophages and neutrophils versus controls. In qPCR-positive individuals, linear regression analysis showed a direct correlation between viral load and airway neutrophils, and between influenza A virus load and airway macrophages. Conclusion: The lung tissue of patients with stable, mild COPD has a higher prevalence and load of respiratory viruses versus non-obstructed control subjects, and increased inflammation of small airways. Respiratory viruses may represent potential targets in COPD patient management.
Introduction
Chronic obstructive pulmonary disease (COPD) is projected to be the third-leading cause of death in the world (Citation1). Bronchioles (small conducting airways less than Citation2-mm in diameter) become the major site of airway obstruction in COPD through inflammation and remodeling (Citation2, 3) and a recent report based on microCT indicates the terminal bronchioles are extensively narrowed and destroyed before emphysematous destruction is detected (Citation4). Histological evidence from several studies supports the presence of both an innate and adaptive immune response in peripheral lung tissues in COPD patients (reviewed in (Citation5)). Although cigarette smoking is recognized as a major risk factor for the development of COPD, other factors must be involved because only 10–15% of heavy smokers have chronic airflow obstruction (Citation6).
Respiratory tract infections by bacteria or viruses have been implicated in triggering a majority of acute exacerbations that punctuate the natural history of COPD and become associated with a more rapid decline in lung function (reviewed in (Citation7)). There is increasing evidence that the human lung is not a sterile environment as previously thought: for example, recent work in characterizing the lung bacterial microbiome in humans has generated considerable interest and is stimulating intensive research activity (Citation8–10). Concerning respiratory viruses, the use of polymerase chain reaction (PCR)-based approaches to amplify virus-specific nucleic acid sequences has resulted in documentation of respiratory viruses in the lower respiratory tract in COPD patients during both acute exacerbations and in stable disease (Citation11–17).
The role of viruses in stable COPD is poorly understood, in part because investigators have used different approaches (typically sputum, nasopharyngeal swabs or aspirates, or bronchoalveolar lavage (BAL) fluids) to sample the human lower respiratory tract (Citation7). A recent study has reported that within the same patient, viral detection by PCR can yield different results in sputum vs. nasal/throat swabs (Citation18).
Previous work in an animal model has also shown that viral detection rates can differ depending on the type of specimen examined: during persistent infections in which there are low levels of virus present in the lungs, the rate of virus detection by PCR is higher in lung tissue specimens in comparison to BAL fluids obtained from the same animals (Citation19). Furthermore, PCR-based approaches have documented co-infections involving multiple respiratory viruses in some patients (Citation7). Although the significance of such co-infections is unclear in COPD, our group has reported that respiratory virus co-infections of children are characterized by a “dominant virus” that contributes to a large majority of the total measured viral load (∼1 order of magnitude higher than the next most abundant virus), such that co-infections are typically predominated by one virus (Citation20). Whether viral co-infections of the lungs of COPD patients are also characterized by a dominant virus is unknown.
To obtain an improved understanding of the prevalence of respiratory viruses in lungs in patients with stable COPD, we sought to determine the relationship between virus-specific nucleic acid sequences present in human lung tissue specimens and concomitant inflammation of small airways in the same core of lung used for viral diagnosis. Because COPD is a heterogeneous, progressive condition that ranges from mild (GOLD 1) to very severe (GOLD Citation4) (Citation21), we focused our study on lung tissue cores obtained from patients who have mild COPD, compared to a control group of cigarette smokers without chronic airflow obstruction.
The specific aims were to compare the COPD and control groups with respect to: 1) Prevalence and load of a panel of common respiratory viruses in lung tissue cores, measured using a panel of real-time, quantitative polymerase chain reaction (qPCR) protocols; 2) Volume fraction (Vv) of the airway wall tissue occupied by inflammatory cells in the same lung tissue cores that underwent qPCR; 3) Prevalence of co-infections in these lung tissue cores, and whether such co-infections were characterized by a dominant virus (Citation20); 4) Relationship between viral load and airway inflammatory cells in lung tissue cores.
Materials and methods
Patients and controls
compares the FEV1/FVC and FEV1 (percent predicted) in patients (n = 20) diagnosed with stable, mild COPD (GOLD 1) to control subjects (n = 20) well-matched for age, gender, and cigarette-smoking history. Three individuals (one from the control group, two from the COPD group) had used inhaled corticosteroids, and none had symptoms of an acute respiratory tract infection documented in the two weeks prior to surgery. Individuals from whom the lung tissue cores were obtained had the following diagnoses: adenocarcinoma (n = 13), squamous cell carcinoma (n = 12), large cell carcinoma (n = 9) and other (n = 6).
Table 1. Characteristics of study patients
Study design
The lung tissue used in this study was obtained from patients being treated for lung tumors by either lobectomy or pneumonectomy, archived in the Biobank of the UBC James Hogg Research Centre, Vancouver, BC, Canada. The frozen lung tissue specimens used were not from any one particular season or year. The methods used to obtain informed consent from the patients and to prepare these specimens are fully described elsewhere and were approved by the applicable Hospital and University review committees (Citation22). Briefly, fresh lung specimens were inflated with 50% (v/v) Tissue-Tek O.C.T. (Sakura Finetek USA Inc, Torrance, CA) in PBS, frozen in liquid nitrogen vapor, cut into contiguous 2-cm-thick slices, and cylindrical cores of tissue were removed from each slice and submitted to the Biobank.
Cores were retrieved based on criteria for selection of patients and controls described here. Consecutive frozen sections were cut from each lung tissue core on a cryomicrotome and were assigned as follows: sections 1–5, 8–12, 14–18 were allocated for qPCR analysis, and sections 6–7, 13, 19–20 were allocated for preparation of microscope glass slides for histological and immunohistochemical staining. This sectioning protocol was repeated in quintuplicate for each lung tissue core and at least two tissue cores were examined from each patient specimen.
qPCR panel for respiratory viruses
The qPCR panel used for quantification of viral load was based on a protocol developed in our laboratory (Citation20) (Supplementary ) and included 13 common respiratory viruses (influenza viruses A and B; parainfluenza virus (PIV)-1, -2, and -3; respiratory syncytial virus (RSV) A and B, human metapneumovirus; human coronavirus-229E, -OC43, and NL63; human rhinovirus, and adenovirus). RPP40, a gene proven to be expressed as a single copy per cell, was used as a human reference gene for normalization of qPCR load (Citation23). Briefly, total RNA from lung tissue was extracted using the RNEasy Mini Kit (Qiagen, Mississauga, Ontario, Canada) and DNA from designated aliquots of the same lung specimens was extracted using the QIAamp DNA Mini Kit (Qiagen). Target sequences that were based on previously published protocols (Citation20) were optimized for reference strains of viruses using Primer Express software (Applied Biosystems, Foster City, CA).
Synthetic controls generated from PCR product cloned into linearized plasmid were used as qPCR standards as this particular approach is the most stable and does not degrade as quickly as amplified PCR product alone (Citation24). These were produced for each virus for the generation of standard curves that allowed absolute quantification of cDNA or DNA from lung tissue (Citation20), with sensitivity to 101 copy-equivalents. All nucleic acid and standards were aliquoted and stored at −80°C unit use. RNA underwent reverse transcription using random hexamers and Superscript II reverse transcriptase (Invitrogen, Burlington, Ontario, Canada). PCR reactions were prepared in the pre-PCR clean room at Children's of Women's Health Centre of British Columbia, Vancouver, BC using positive displacement pipettes and aerosol barrier tips.
qPCR standards were carefully opened and added last to the plate to minimize the possibility of contamination. Standardized qPCR cycling conditions consisted of an initial incubation of 2 minutes at 50°C, 10 minutes at 95°C and 40 amplification cycles comprising of 15 seconds at 95°C and 1 minute at 60°C. Fluorescence was quantified in each well by the ABI PRISM7000 (Applied Biosystems). Results were reported as the median viral load per 1000 human cells per patient.
Quantitative histology for inflammation of small airways
The 20 cryosections from each core of lung tissue, mounted onto separate glass slides for histological examination, were used to randomly assign individual sections for immunostaining of: macrophages, dendritic cells, follicular dendritic cells, natural killer (NK) cells, CD4+ T-lymphocytes, CD8+ T-lymphocytes, B-lymphocytes, neutrophils and eosinophils (Citation3). Supplementary lists the antibodies and dilutions used for immunostaining of frozen lung sections. A point counting method was used to generate volume fraction (Vv) of inflammatory cells into airway wall tissue (Citation3) using ImagePRO Plus software version 4.0 (MediaCybernetics Inc., Bethesda, MD). No significant difference was found between the two different cores from the same individual (p > 0.05) and these measurements were averaged and reported as a single value per person.
Table 2. qPCR detection of respiratory viruses
Data analysis
Unless otherwise specified, continuous data were expressed as median (range) (Citation13). Statistical analyses were carried out using SPSS Statistics software v. 20 (IBM, Armonk, NY). For categorical data, a chi-square test was used to compare the proportions of patients positive for viruses between the control and COPD groups. The two groups were compared using the non-parametric Mann–Whitney U-test for continuous data that were not normally distributed, and Student's t-test was used for normally distributed data. For co-infections, one-way analysis of variance (ANOVA) was used to compare the mean percent contribution of the load of an individual virus to the total load measured by the qPCR panel. Linear regression analysis was used to examine the relationship between lung function (FEV1/FVC) and Vv of inflammatory cells within airway wall tissue, and between airway inflammatory cells and qPCR loads. Two-tailed p-value < 0.05 was considered to be statistically significant.
Results
Increased prevalence and load of respiratory viruses in lung tissue of patients with stable, mild COPD
The qPCR panel detected viral nucleic acid in 18/40 (45.0%) of the individuals studied. Out of the three persons who had been treated with corticosteroids, one tested positive for virus. The most common viruses detected by the qPCR panel were influenza A and human coronavirus 229E, each detected in 10/40 (25.0%) individuals. The prevalence of qPCR positivity of other viruses were: PIV-2 in 3/40 (7.5%), adenovirus in 2/40 (5.0%), influenza B in 1/40 (2.5%) and human rhinovirus in 1/40 (2.5%). Viral co-infections were documented in 7/40 (17.5%) of the study population, consisting of five dual infections and two triple infections. shows the results comparing qPCR results between the COPD and control groups. A significantly higher proportion of COPD patients were virus-positive by qPCR in comparison to control subjects (65.0% vs. 25.0%, p = 0.011). The proportion of individuals positive for influenza A virus was higher in COPD vs. controls (40.0% vs. 10.0%, p = 0.028).
In comparison to controls, COPD patients also had a significantly greater total viral load (p = 0.010), greater influenza A load (p = 0.019) and greater number of viruses per patient (p = 0.007) (). There were no significant differences for the load of the other individual viruses within the qPCR panel. Concerning co-infections, dual infection was documented in one control subject; COPD patients had four dual infections and two triple infections. In dual infections, the virus with the highest individual load contributed a significantly higher percentage toward total viral load than the second virus (81.5 ± 16.3% vs. 17.5 ± 16.3% (mean ± SD), p = 0.0003); in triple infections, the percent contribution of individual loads to total load were significantly different between the three viruses (72.9 ± 3.5% vs. 22.9 ± 7.1% vs. 5.5 ± 2.7% (mean ± SD), p < 0.001), indicating that co-infections of the lung were characterized by the presence of a dominant virus. Neither prevalence nor load of respiratory viruses was associated with a particular lung cancer diagnosis (Supplementary Tables and 4).
Table 3. Quantitative histology for airway inflammatory cells*
Increased inflammatory cells in the small airways of patients with stable, mild COPD
shows the results of quantitative histology for airway wall inflammation between the COPD and control groups. The small airways of COPD patients had a significantly higher median Vv of macrophages (p = 0.004) and neutrophils (p = 0.028) in comparison to the control subjects, with no significant differences observed between the two groups in the Vvs of the other inflammatory cell types studied, or in the thickness of airway wall or smooth muscle. Further analysis of COPD patients by qPCR status showed a trend toward increased Vv neutrophils in virus-positive vs. virus-negative patients (p = 0.12; not shown). Results of linear regression analysis () showed statistically significant inverse relationships between FEV1/FVC ratio and the Vv of macrophages (p = 0.044) and neutrophils (p = 0.047). There was a statistically significant direct relationship between the Vv of neutrophils and total viral load (p = 0.031) and in influenza A-positive individuals, between the Vv of macrophages and influenza A load (p = 0.038).
Figure 1. Linear regression analysis of the relationship between inflammatory cells and lung function, and viral load. A) FEV1/FVC vs. Volume fraction CD68 + macrophages (p = 0.044). B) FEV1/FVC vs. Volume fraction neutrophils (p = 0.047). C) Total viral load (normalized to RPP) vs. Volume fraction of neutrophils (p = 0.031). D) Influenza A viral load (normalized to RPP) vs. Volume fraction of CD68+ macrophages (influenza A-positive cases; p = 0.038).
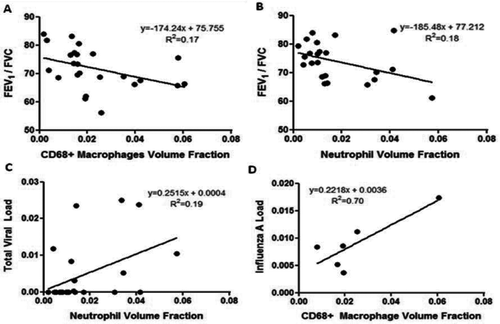
Discussion
This study examined the prevalence and quantitative loads of a panel of respiratory viruses and quantification of small airway inflammatory cell infiltrates in human lung tissue specimens, comparing patients with stable, mild COPD to control subjects (smokers without airflow obstruction) matched for age, gender and cigarette-smoking history. The lung specimens studied were archival specimens from our institutional Biobank, having originated from patients who underwent surgery for removal of lung tumors. It is unclear whether our findings in lung cancer patients are also applicable to COPD patients who do not have cancer; however, lung cancer and COPD are common co-morbidities (Citation25, 26) and the COPD and control groups studied were comparable to each other, such that the differences observed between the two groups could not be attributed to the presence or absence, or type of malignancy.
To our knowledge, this is the only study to date that has used qPCR to document viral nucleic acid in a substantial set of lung tissue specimens rather than nasal, BAL or sputum samples, upon which other human studies are based. The apparently high prevalence of viral nucleic acid documented in the lungs of clinically stable patients is consistent with our previous findings in an animal model of experimental RSV infection, where viral nucleic acid could be detected in the lung tissue—but not BAL fluid—of guinea pigs for at least 60 days after viral inoculation (Citation19). Although PCR is a powerful technique that is more sensitive than viral culture (Citation12), our results need to be interpreted with caution, since PCR can detect nucleic acid sequences that originate from inactivated viruses as well as from viable, infectious viruses, and in this study we could not definitively attribute positive qPCR results to the presence of replicating viruses within the lungs.
Our results showed that COPD patients had a significantly increased prevalence of respiratory viruses and higher total viral loads in lung tissue in comparison to controls. Concerning specific viruses, the detection rate for influenza A virus was not wholly unexpected, as results of two previous studies of human lung specimens (Citation11) and lower respiratory airway secretions (Citation27) using conventional PCR showed comparable rates of influenza A virus PCR-positivity to what we documented here by a different qPCR method. With regard to RSV, previous studies have reported a wide range of positive detection rates in stable COPD patients: in a longitudinal study of COPD patients during acute exacerations and when clinically stable.
Seemungal et al. (Citation12) documented RSV nucleic acid in 23.5% of nasal samples obtained from stable patients, yet other investigators have reported RSV PCR-positivityon the order of 0 – 3% during stable COPD (Citation13, Citation28, Citation29). In the current study, our inability to document RSV nucleic acid in the lung tissue cores examined could reflect differences in patient populations, and/or or the site/timing of sampling with respect to clinical stage or activity of disease. A limitation of our study is that the documentation of respiratory viruses was done at a single point in time and was not temporally related to acute exacerbations of COPD, which are very difficult to study using lung biopsies (Citation30).
The small airways of COPD patients had increased Vv of macrophages and neutrophils in comparison to the control group, and linear regression analysis showed that both of these cell types were inversely correlated with FEV1/FVC ratio. Moreover, the Vv of neutrophils was directly related to the total viral load in the same lung tissue cores studied; as was the Vv of macrophages and influenza A virus load in influenza A virus-positive individuals. These findings need to be interpreted with caution, since virus-negative COPD patients also had evidence of airway inflammation. The relationship between viral qPCR findings and inflammation of small airways in COPD requires a larger number of cases to determine the significance of the trend toward increased Vv of neutrophils with virus-positivity. Further characterization of the relationship between viral load and airway inflammation within a given GOLD stage of COPD, and their potential clinical significance, is an area of future research.
Our findings are also consistent with those reported in a mouse model of asthma and mild COPD, in which low level virus persistence in the lungs was documented after initial viral challenge, in association with a persistently augmented host innate immune response (Citation31). In this model, virus infection is considered to play a pathogenic role by stimulating the increased innate immune response. The presence of viruses during stable COPD has been suggested by others to contribute to the elevated inflammatory background seen in COPD (Citation7, Citation16). We postulate that viruses might act as a reservoir; in a similar manner as suggested for bacteria by Sethi and Murphy (Citation32) and Sethi et al. (Citation33), and that COPD patients are more susceptible to virus infection and persistence.
In addition to the presence or absence of viruses in the lungs, virus-host interactions may involve host genetic factors that could influence clinical manifestations, as evidenced by differences observed in RSV load and virus-associated airway inflammation and non-specific airway hyperresponsiveness between different inbred strains of guinea pigs during persistent lung infection (Citation34). Concerning viral co-infections, our results in adult lung tissues with COPD show evidence of a dominant virus that contributes a large majority of the total viral load (> 80% of the total load for dual infections; > 70% of the total load for triple infections), similar to what we have previously observed in the upper respiratory tract of symptomatic children, in which the dominant virus in that setting typically contributed ∼90% of the total measured viral load (Citation20). Further research is required to understand the potential implications of this emerging paradigm on the pathogenesis of airway inflammation in COPD, and on the effects of specific anti-viral interventions, for example whether elimination of one virus with a specific anti-viral therapy could facilitate increased replication and burden resulting from a second virus.
Our findings also confirm previous findings regarding the histopathological features of mild COPD in a different set of human lung tissue specimens than was originally studied (Citation3). Analysis of airway inflammation over the full range of FEV1 has shown that there is both increased extent (i.e. percentage of bronchiolar wall tissue containing inflammatory cells) and severity (i.e. the accumulated volume of inflammatory cells) in bronchiolar wall tissue related with progression of disease, with the differences for macrophages between GOLD 1 patients and the control subjects (smokers with normal lung function) greater than for B-cells, CD4+ and CD8+ T-cells (Citation3).
Targeting cells involved in innate immunity is being considered as a strategy in the management of COPD, with a recent study reporting that guinea pigs treated with a pharmacological inhibitor of myeloperoxidase, an inflammatory mediator produced by neutrophils and macrophages, are protected from progression of smoking-associated COPD (Citation35). Regarding the possible role of viruses in linking innate and adaptive immunity, Tate et al. (Citation36) reported that neutrophils can sustain effective CD8+ T-cell responses in influenza virus-infected mice.
The current findings and our group's previous documentation of influenza A virus nucleic acid in the human lung and in lower respiratory tract specimens (Citation11, Citation15) corresponds with literature that reviews influenza virus in COPD, as a relevant target for clinical intervention through anti-viral therapy and/or immunization (Citation37). Regarding the potential relationship between the bacterial microbiome and respiratory viruses, Ichinohe et al. (Citation38) reported that commensal organisms comprising the bacterial microbiome in the gastrointestinal tract can regulate the mucosal immune response of the respiratory tract to influenza virus infection in mice. Extending this intriguing concept to humans is another area of potential study.
In summary, the results of this study show that lung tissue specimens of patients with stable, mild COPD differ from cigarette smokers without chronic airflow obstruction by having a higher prevalence and load of common respiratory viruses. In addition, the small airways from the same areas of lung tissue that were tested for viruses showed increased inflammation by macrophages and neutrophils in COPD patients when compared to controls. Viral infections may represent a target for intervention in the management of patients with COPD.
Declaration of Interest Statement
None of the authors have any relationships to declare that could influence the findings of this manuscript. Funding was provided by the British Columbia Lung Association and Michael Smith Foundation for Health Research. The study sponsors played no role in the study design; in the collection, analysis and interpretation of data; in the writing of the report; and in the decision to submit the paper for publication.
Acknowledgments
Authors Soraya Utokaparch and Marc A. Sze contributed equally to this work.
References
- Murray CJ, Lopez AD. Alternative projections of mortality and disability by cause 1990–2020: Global Burden of Disease Study. Lancet 1997; 349:1498–1504.
- Kuwano K, Bosken CH, Paré PD, Bai TR, Wiggs BR, Hogg JC. Small airways dimensions in asthma and in chronic obstructive pulmonary disease. Am Rev Respir Dis 1993; 148:1220–1225.
- Hogg JC, Chu F, Utokaparch S, The nature of small-airway obstruction in chronic obstructive pulmonary disease. N Engl J Med 2004; 350:2645–2653.
- McDonough JE, Yuan R, Suzuki M, Small-airway obstruction and emphysema in chronic obstructive pulmonary disease. N Engl J Med 2011; 365:1567–1575.
- Hogg JC, Timens W. The pathology of chronic obstructive pulmonary disease. Annu Rev Pathol 2009; 4:435–459.
- Fletcher C, Peto R. The natural history of chronic airflow obstruction. Br Med J 1977; 1: 1645–1648.
- Beasley V, Joshi PV, Singanayagam A, Molyneaux PL, Johnston SL, Mallia P. Lung microbiology and exacerbations in COPD. Int J COPD 2012; 7:555–569.
- Erb-Downward JR, Thompson DL, Han MK, Analysis of the lung microbiome in the “healthy” smoker and in COPD. PLoS ONE 2011; 6: e16384.
- Sze MA, Dimitriu PA, Hayashi S, The lung tissue microbiome in chronic obstructive pulmonary disease. Am J Respir Crit Care Med 2012; 185:1073–1080.
- Han MK, Huang YJ, Lipuma JJ, Significance of the microbiome in obstructive lung disease. Thorax 2012; 67:456–463.
- Hogg JC, Macek V, Dakhama A, The prevalence of common respiratory viruses in human lungs. In: Marone G, Austen KF, Lichtenstein LM (Eds.) Asthma and Allergic Diseases: Physiology, Immunopharmacology, and Treatment. Academic Press, San Diego CA, 1998: 321–333.
- Seemungal T, Harper-Owen R, Bhowmik A, Respiratory viruses, symptoms, and inflammatory markers in acute exacerbations and stable chronic obstructive pulmonary disease. Am J Respir Crit Care Med 2001; 164:1618–1623.
- Rohde G, Wiethege A, Borg I, Respiratory viruses in exacerbations of chronic obstructive pulmonary disease requiring hospitalisation: a case-control study. Thorax 2003; 58:37–42.
- Qiu Y, Zhu J, Bandi V, Biopsy neutrophilia, neutrophil chemokine and receptor gene expression in severe exacerabations of chronic obstructive pulmonary disease. Am J Respir Crit Care Med 2003; 168:968–975.
- Tan WC, Xiang X, Qiu D, Epidemiology of respiratory viruses in patients hospitalized with near-fatal asthma, acute exacerbations of asthma, or chronic obstructive pulmonary disease. Am J Med 2003; 115:272–277.
- Wedzicha JA. Role of viruses in exacerbations of chronic obstructive pulmonary disease. Proc Am Thor Soc 2004; 1:115–120.
- Singh M, Lee SH, Porter P, Human rhinovirus 2A induces TH1 and TH2 immunity in patients with chronic obstructive pulmonary disease. J Allergy Clin Immunol 2012; 125:1369–1378.
- Falsey AR, Formica MA, Walsh EE. Yield of sputum for viral detection by reverse transcriptase PCR in adults hospitalized with respiratory illness. J Clin Microbiol 2012; 50:21–24.
- Dakhama A, Chan NG, Ahmad HY, Usefulness of bronchoalveolar lavage for diagnosis of acute and persistent respiratory syncytial virus lung infections in guinea pigs. Pediatr Pulmonol 1998; 26:396–404.
- Utokaparch S, Marchant D, Gosselink JV, The relationship between respiratory viral loads and diagnosis in children presenting to a pediatric hospital emergency department. Pediatr Infect Dis J 2011; 30:e18–23.
- Rabe KF, Hurd S, Anzueto A, Global strategy for the diagnosis, management, and prevention of chronic obstructive pulmonary disease: GOLD executive summary. Am J Respir Crit Care Med 2007; 176:532–555.
- Gosselink JV, Hayashi S, Chau E, Cooper J, Elliott WM, Hogg JC. Evaluation of small sample cDNA amplification for microdissected airway expression profiling in COPD. COPD 2007; 4:91–105.
- McNees AL, White ZS, Zanwar P, Vilchez RA, Butel JS. Specific and quantitative detection of human polyomaviruses BKV, JCV, and SV40 by real time PCR. J Clin Virol 2005; 34(1):52–62.
- Dhanasekaran S, Doherty TM, Kenneth J; TB Trials Study Group. Comparison of different standards for real-time PCR based absolute quantification. J Immunol Meth 2010; 354(1–2): 34–39.
- Young RP, Hopkins RJ, Christmas T, Black PN, Metcalf P, Gamble GD. COPD prevalence is increased in lung cancer, independent of age, sex and smoking history. Eur Respir J 2009; 34:380–386.
- Powell HA, Iyen-Omofoman B, Baldwin DR, Hubbard RB, Tata LJ. Chronic obstructive pulmonary disease and risk of lung cancer: the importance of smoking and timing of diagnosis. J Thorac Oncol 2013; 8:6–11.
- Macek V, Dakhama A, Hogg JC, Green FH, Rubin BK, Hegele RG. PCR detection of viral nucleic acid in fatal asthma: is the lower respiratory tract a reservoir for common viruses? Can Respir J 1999; 6:37–43.
- Falsey AR, Formica MA, Hennessey PA, Criddle MM, Sullender W, Walsh EE. Detection of respiratory syncytial virus in adults with chronic obstructive pulmonary disease. Am J Respir Crit Care Med 2006: 173:639–643.
- Papi A, Bellettato CM, Braccioni F, Infections and airway inflammation in chronic obstructive pulmonary disease severe exacerbations. Am J Respir Crit Care Med 2006; 173:1114–1121.
- Pare PD, Hegele RG, Hogg JC. The lung pathology of acute exacerbations of COPD. Lung Biol Health Dis 1996; 92:13–22.
- Kim EY, Battalie JT, Patel AC, Persistent activation of an innate immune axis translates respiratory viral infection into chronic lung disease. Nature Med 2008; 14:633–640.
- Sethi S, Murphy TF. Infection in the pathogenesis and course of chronic obstructive pulmonary disease. New Engl J Med 2008: 359:2355–2365.
- Sethi S, Mallia P, Johnston SL. New paradigms in the pathogenesis of chronic obstructive pulmonary disease II. Proc Am Thorac Soc 2009; 6:532–534.
- Sutton TC, Tayyari F, Khan MA, Manson HE, Hegele RG. T helper 1 background protects against airway hyperresponsiveness and inflammation in guinea pigs with persistent respiratory syncytial virus infection. Pediatr Res 2007; 61(5 Pt 1):525–529.
- Churg A, Marshall CV, Sin DD, Late intervention with a myeloperoxidase inhibitor stops progression of experimental chronic obstructive pulmonary disease. Am J Respir Crit Care Med 2012; 185:34–43.
- Tate MD, Ioannidis LJ, Croker B, Brown LE, Brooks AG, Reading PC. The role of neutrophils during mild and severe influenza virus infections of mice. PLoS One 2011; 6:e17618.
- Mallia P, Johnston SL. Influenza infection and COPD. Int J Chron Obstruct Pulmon Dis 2007; 2:55–64.
- Ichinohe T, Pang IK, Kumamoto Y, Microbiota regulates immune defense against respiratory tract influenza A virus infection. Proc Natl Acad Sci USA 2011; 108:5354–5359.