Abstract
Background: The purpose of this study was to evaluate the longitudinal relationship between functional exercise capacity, assessed through standardized 12-minute walk test (12MWT), and various lung function parameters obtained using spirometry, body plethysmography and diffusing capacity (DLco) measurements in patients with COPD. Methods: Spirometry, body plethysmography and DLco-measurements were performed at baseline in 84 subjects with moderate to very severe COPD and at follow-up visit (n = 34) after 5 years. Functional exercise capacity was determined using standardized 12MWT. Results: Patients were characterized at baseline by FEV1 of 1.2 ± 0.4 L (41 ± 13% predicted), RV of 3.4 ± 1.0 L (187 ± 58% predicted) and DLco of 3.8 ± 1.2 mmol/min/kPa (51 ± 16% predicted). A decrease of 12MWD was found between baseline and follow-up (928 ± 193 m vs. 789 ± 273 m, p < 0.001). DLco and 12MWD at baseline were the only independent predictors of 12MWD at follow-up in a multiple logistic regression model that also included all other lung function parameters, gender, age and BMI. Decline in 12MWD was mainly explained by deterioration in DLco. Furthermore, DLco value at baseline had the highest explanatory value for the loss in 12MWD after 5 years (R2 = 0.18, p = 0.009). Conclusions: In a 5-year longitudinal study, DLco-measurements at baseline were the most important predictors of declining exercise capacity in COPD patients. These results suggest that integration of DLco in the clinical workup provides a more comprehensive assessment in patients with COPD.
Keywords:
Introduction
Chronic obstructive pulmonary disease (COPD) is a global health challenge, as it is the only leading cause of death with rising mortality and morbidity, and predicted to be the third cause of death worldwide by the year 2030 (Citation1). A fixed post-bronchodilator ratio of < 0.7, for forced expiratory volume in one second (FEV1) to forced vital capacity (FVC) (FEV1/FVC), is required to make the diagnosis, with the severity of airflow obstruction defined by FEV1 as the only lung function parameter delineating the disease category in the Global Strategy for the Diagnosis, Management, and Prevention of COPD (GOLD) guidelines (Citation2). However, disease progression in COPD is characterized not only by progressive airflow limitation but also increased hyperinflation, worsening exertional dyspnea with an accompanying decline in functional exercise capacity (Citation3). Increasing evidence demonstrates that parameters of airflow obstruction do not reflect the level of lung hyperinflation and does not fully reflect the exercise capacity and the exertional dyspnea in COPD patients (Citation4, 5).
Walk tests are used to assess functional exercise capacity in COPD patients as walking increases oxygen consumption and desaturation in patients with COPD more than cycling with similar work-rate profiles and may more closely resemble a patient's normal activities (Citation6). Among the most commonly used submaximal exercise tests to determine COPD patients’ functional capacity are the 6- or 12-minute walk tests (6MWT or 12MWT) (Citation7–9). These walk tests are inexpensive and practical tests for evaluating the functional status of patients with COPD and evidence shows that poor performance in walk test has prognostic value in patients with COPD (Citation10, 11).
Pulmonary gas exchange across the alveolar-capillary membrane, measured as carbon monoxide diffusion capacity (DLco), provides quantitative and qualitative assessment of gas transfer in the lungs. Mechanistically, a reduced DLco in COPD can reflect the alveolar-capillary membrane damage subsequent to emphysema (Citation12), increased pressure in the pulmonary circulation (Citation13) or secondary to heart failure (Citation14) and therefore is not necessarily linked to the degree of airway obstruction. A number of publications suggest that DLco is closely linked to impaired exercise capacity, elevated inflammatory biomarkers and arterial oxygen desaturation in patients with COPD (Citation15–17). Furthermore, DLco has been found to be an independent predictor of mortality in COPD patients (Citation18). However the impact of DLco on walking distance and walking distance changes over time has been limitedly investigated in COPD patients.
The purpose of our study was to evaluate both cross-sectional and longitudinal associations between functional exercise capacity assessed through standardized 12MWT and lung function parameters in patients with COPD, obtained using spirometry, body plethysmography and DLco-measurements. The goal was to determine which lung function parameters have the best predictive value for limitation and change in functional exercise capacity.
Materials and methods
Participants
A total of 89 patients with moderate-to-very severe COPD were consecutively invited to take part in the study when being referred for physical training to the Physiotherapy Unit of the Respiratory Department of the University Hospital in Uppsala, Sweden or to the Physiotherapy Unit of the Pulmonary Section at the Central Hospital in Västerås, Sweden. The patients were invited to participate in an 8-week pulmonary rehabilitation program (training twice weekly) as described in detail elsewhere (Citation19). The pulmonary function and exercise tests were performed before and 5 years after the rehabilitation program.
Here, 84 patients met the following criteria:
had FEV1 < 80% predicted, FEV1/FVC ratio < 0.7 after bronchodilatation, and a total lung capacity of >70% predicted.
were in a non-acute phase of their disease and receiving a stable drug regimen.
performed 12MWT.
performed DLco–measurement.
All study participants were ex- or current smokers. Participants had no coexisting medical conditions that would interfere with physiological testing, exercise or the ability to comprehend written or oral instructions. Exclusion criteria included the presence of cardiac disease, claudication limiting exercise capacity and musculoskeletal problems.
The patients who were recruited in Uppsala center (n = 72) were invited to take part in the follow-up survey 5 years after the base line visit. Thirty-four patients agreed to participate in the follow-up and performed all tests. The non-participants in the follow-up (n = Citation38) were distributed as following: 21 patients declined further participation in lung function and exercise testing, 14 patients had died and 3 patients could not be contacted.
The 12-minute walk test
The 12-minute walk tests were performed, as described elsewhere (Citation20), in a 34-m, level corridor. Initially, two tests were performed for practice, and a third served as baseline. During follow-up, only one test was performed. The subjects were asked to cover as much ground as possible in 12 minutes at their own speed and to pause if necessary. To prohibit bias, no encouragement was given to the subjects and the physiotherapist did not walk alongside the patients. The patients were told the time at standard intervals (Citation4, Citation6, Citation8, Citation10 and Citation11 minutes). The 12MWT was performed in all 84 individuals at baseline and in 34 individuals at the follow-up study after 5 years (mean ± SD: 5.2± 0.25 years).
Pulmonary function tests
Spirometry was performed using a Masterlab Trans Spirometer (Erich Jaeger AG, Würzburg, Germany). Lung volumes were obtained with a Masterlab Body Plethysmograph (Erich Jaeger AG, Würzburg, Germany). The normal values according to Hedenström et al were used (Citation21, 22). DLco was measured using the single-breath technique using Masterlab Transfer (Erich Jaeger AG, Würzburg, Germany) (Citation23). The corrected values for actual hemoglobin values were used in the calculations.
All lung function testing was performed by highly experienced technicians following the standards outlined by ATS/ERS (Citation23–25). Post-bronchodilatory values were used in the calculations, except for DLco where pre-bronchodilatory values were used as no DLco -maneuver was done after bronchodilatation. Post-bronchodilatory lung function values were measured 30 minutes after the patients inhaled 5 mg salbutamol and 0.5 mg ipratropium via a nebulizer. All subjects performed all lung function tests at baseline. A total of 28 of 34 individuals performed post-bronchodilatory spirometry at follow-up, while 25 of 34 individuals performed DLco and body plethysmography.
The lung function parameters retrieved from the pulmonary function test were: FEV1, FVC, vital capacity (VC) and inspiratory capacity (IC) from spirometry, total lung capacity (TLC), residual volume (RV), airway resistance (Raw), functional residual capacity (FRC), inspiratory fraction (IC/TLC) and RV/TLC-ratio from body plethysmography, and diffusing capacity corrected for hemoglobin level.
Statistical analysis
Statistics were generated using computer software programs (STATA 12.1, StataCorp, College Station, TX, USA). Means ± standard deviations (SDs) were used to present the descriptive statistics.
The patients were divided in tertiles according to 12 min walk distance (12MWD) or changes in 12MWD over 5 years (∆12MWD) and a trend analysis of lung function was performed across these groups, with lung function parameters as predictors and 12MWD as an outcome.
Simple linear regression was used to analyze the correlation between different single lung function parameters and 12MWD and this was presented as the square of the correlation coefficient (r2). Stepwise regression model which included lung function parameters demonstrating statistically significant correlation to 12MWD, as well as sex, age, BMI, participating in the pulmonary rehabilitation program and smoking habit was used to determine the most important lung function predictors of 12MWD. Baseline 12MWD was additionally introduced in the specific model analyzing the relation between lung function parameters at baseline and 12MWD at follow-up. Standardized regression coefficients (R2) were calculated for the lung function parameters in multiple linear regression models, presented in Table . A p value < 0.05 was considered statistically significant.
Table 1. Demographic characteristics and comprehensive indices of pulmonary function for all COPD patients evaluated at baseline visit
Table 2. Indices of lung function parameters for COPD patients participating in both baseline and follow-up visits, n = 34
Table 3. Baseline lung function values in lower, middle and upper tertiles of 12-minute walk distance at baseline
Table 4. Stepwise regression models of determinants of 12-minute walk distance
Ethics
The study was approved by the Medical Ethics Committee of Uppsala University, number 01–159, and all patients gave written informed consent.
Results
Population characteristics
Patient characteristics and lung function parameters for the whole group as well as participants and non-participants in the follow-up study are described in Table . The majority of the participants were ex-smokers at baseline (77%) and only one patient stopped smoking during the follow-up period. Participating subjects in the follow-up were characterized by higher FEV1 and DLco in absolute values, but not in percent of predicted. A significantly higher 12MWD at baseline was found in participating subjects. No differences between subjects who declined/ were not able to participate in follow-up and subjects who died before follow-up were found regarding DLco (2.9 ± 1.1 mmol/s/kPa for decliners vs 2.8 ± 1.1 mmol/s/ kPa for deceased subjects, p = 0.86), 12MWD (826 ± 158 m for decliners vs 750 ± 192 m for deceased subjects, p = 0.20) or FEV1 (1.0 ± 0.3 L for decliners vs 0.9 ± 0.3 L for deceased subjects, p = 0.30).
Lung function parameters at inclusion and follow-up after 5 years are presented in Table for the 34 participants who performed 12MWT both at baseline and follow-up. A significant decrease between study visits was found for VC, FVC, FEV1, DLco and 12MWD and a significant increase was found for TLC, FRC, RV and RV/TLC whereas IC, IC/TLC and Raw remained unchanged at follow-up.
12MWD in relation to lung function at baseline visit
Higher DLco, FEV1, IC, and VC-values were associated with increased 12MWD, while having higher RV, RV/TLC and Raw was associated with decreased 12MWD (Table ). In simple linear regression analysis, the strongest correlation to 12MWD was found with FEV1, followed by Raw, RV/TLC, IC and DLco with r2 values at 0.36, 0.27, 0.23, 0.21 and 0.18 respectively (Figure ). Other lung function parameters were also significantly associated with 12MWD, with the exception of FRC and TLC.
Figure 1. Explanatory value (expressed in r2-values from simple linear regression models) of each of the lung function parameters at baseline for the patients’ exercise capacity at baseline. *Indicates significant relation (p < 0.05).
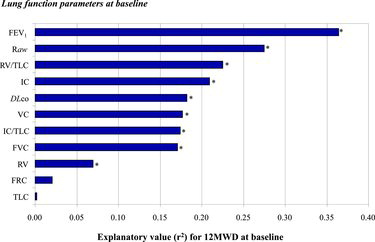
FEV1, BMI and DLco were the main determinants of 12MWD with an explanatory value (R2) of 37% in a stepwise regression model including sex, age, smoking and completed physical rehabilitation program as shown in Table . None of the lung volumes obtained using body plethysmography contributed to the explanatory value of the model.
12MWD in relation to lung function at follow-up visit
A lower level of DLco, FEV1, IC, FVC and VC was found across lower 12MWD tertiles at the 5-year follow-up (p value for trends < 0.05). In simple linear regression analysis, the strongest correlation to 12MWD was found with DLco (r2 = 0.57), followed by FEV1 (r2 = 0.402) and RV/TLC (r2 = 0.399) (Figure ).
Figure 2. Explanatory value (expressed in r2-values from simple linear regression models) of each of the lung function parameters at follow-up for the patients’ 12MWD at follow-up. *Indicates significant relation (p < 0.05).
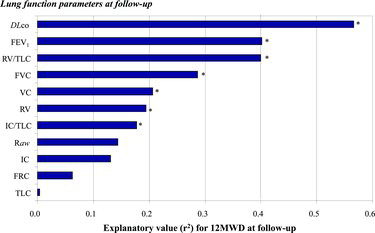
Stepwise regression analysis including above mentioned confounders revealed DLco and RV as the main lung function determinants of 12MWD with an explanatory value (R2) of 79% (Table ).
Decline in 12MWD in relation to changes in lung function
When a simple linear regression model was used, changes in diffusing capacity (∆DLco) had the strongest correlation to change in 12MWD (r2 = 0.30) followed by VC, FVC, and FEV1 (r2 = 0.29, r2 = 0.29 and r2 = 0.24, respectively). ∆DLco and ∆FVC were the main predictors of ∆12MWD in a stepwise regression analysis including sex, age, smoking, BMI and completed physical rehabilitation program, with an explanatory value (R2) of 48% (Table ).
Decline in 12MWD in relation to lung function at baseline
In simple linear regression analysis with loss of 12MWD over time as the outcome, a low DLco at baseline appeared as the only significant predictor (p = 0.002), with the highest predictive value (r2 = 0.26) (Figures and ). DLco was found independently associated with changes in 12MWD over time (p = 0.003) in a stepwise regression model (Table ).
Discussion
In the present study we found that the decline of functional exercise capacity in COPD subjects, assessed through 12MWD, was mainly explained by the deterioration of diffusion capacity of carbon monoxide. DLco was a consistent and strong predictor of 12MWD at both baseline and follow-up visits, while none of the lung volumes obtained using body plethysmography offered additional information on exercise capacity. Furthermore, DLco at baseline related to change in 12MWD over time and 12MWD at follow-up, suggesting a prognostic value of DLco for deterioration of exercise capacity in COPD patients.
The strength of our study is the standardized methodology with all lung function and exercise testing parameters collected by the same research technicians in a clinical physiology laboratory setting. This is, to our knowledge, the first longitudinal study that has data on complete lung function testing and walking distance. Another advantage is the preponderance of female subjects in our material, as many previous studies concerning exercise ability in COPD patients have included more men than women (Citation26).
A limitation of our study is the fact that we used the resting lung function parameters to predict 12MWD, as it is suggested that increasing lung hyperinflation during exercise (dynamic hyperinflation) is more closely related to exercise intolerance and clinical dyspnea than airflow limitation and static hyperinflation (Citation27). Another limitation of the present study is the low number of subjects that could be reexamined at follow-up, 5 years later. This is, however, an inherent problem with long-term studies in COPD patients (Citation28, 29).
For example, in the Inhaled Steroids in Obstructive Lung Disease in Europe trial, among the 751 patients, 349 (47%) dropped out during the survey duration at 3 years (Citation30). Considering the observational period of 5 years, the fact that our study protocol included exercise testing, and the targeted moderate-to very-severe COPD patients in the our investigation, the dropout rate is in line with the above named study. However, generalization of the result should be done with caution due to the limited number of subjects in our study and further investigation is required to prove the consistency of our results.
In our study, an increased loss of DLco over time had the best predictive value for an increased loss in 12MWD. No previous studies to date have investigated this relationship. Decreased DLco, considered to reflect qualitative impairment of the alveolar-capillary membrane, is associated with conditions of diffuse alveolar-capillary damage, reduced alveolar surface or decreased capillary density (Citation31) and does not necessarily reflect the grade of airflow obstruction in COPD patients. Dransfield et al. reported, in a sub-study of National Lung Screening Trial, that emphysema was present in all stages of COPD and that deteriorated FEV1 was not a significant predictor of the severity of emphysema (Citation32). In another study, Shaker et al reported that almost 90% of patients with COPD showed signs of emphysema in their CT-scan regardless of disease severity (Citation33). Furthermore, ex-smoking subjects without airway obstruction have abnormal DLco (Citation34). These findings suggest that the magnitude of diminished diffusion surface in COPD patients is often underestimated. Moreover, COPD often co-exists with numerous other conditions such as heart failure (Citation35) and pulmonary arterial hypertension (Citation13), which are poorly reflected in measurements of expiratory air flow limitation, but are associated with an impaired DLco.
Interestingly, our subset of patients did not demonstrate any significant FEV1 decline during the follow-up period. This might be explained by the fact that the majority of the patients were already ex-smokers and had relatively low starting FEV1-values, which can lead to a more modest deterioration of FEV1 (–100 ml with a p-value of 0.13) (Citation36). However there was a significant decline in DLco, despite smoke cessation, that could be related to progression of emphysema, increased ventilation heterogeneity, increase in pulmonary artery pressures and/or eventual development of pulmonary hypertension and either newly-onset or aggravated heart failure. Echocardiography and high resolution computed tomography could have offered more information on these aspects, but unfortunately these were not possible to include in study protocol.
DLco was a consistent and strong predictor of 12MWD at both baseline and follow-up visits. These results are in line with the report of an association between 6MWD and DLco in the few previous studies available in literature (Citation37–39). Furthermore, recent studies suggest that abnormal DLco relates to poorer 6MWD even in ex-smoking subjects without COPD (Citation34). We have also previously reported an association between DLco and peak exercise capacity in patients with COPD, assessed using cycle ergometry (Citation15). Therefore DLco appears to be an important predictor of exercise capacity, disregarding the type of functional assessment, and together with this consistent predictive value both at baseline and follow-up, implies that DLco provides a more comprehensive understanding of COPD patients’ cardiopulmonary limitations, which has also been suggested by other investigators (Citation15, Citation40).
The lung volumes measured using body plethysmography had a low predictive value for the 12MWD. This is in accordance with Owen et al who reported that while FEV1 and DLco were significantly lower in patients with exercise-induced desaturation, FRC, TLC and RV were not significantly related to desaturation during exercise (Citation17).
We have previously studied the relation between exercise capacity, measured using cycle ergometry, and lung function parameters in COPD patients. However, functional exercise capacity assessed through walk tests better reflects the daily activities of COPD patients (Citation41). The walk test has over the last decade gained more importance as a reliable tool in COPD patient-assessment and a dependable predictor of mortality (Citation42, 43). Although most studies have used the 6-minute walk test, 12MWD has been shown to be more closely associated with maximal oxygen uptake in COPD than walk tests using shorter time intervals (Citation8). Similarly, in rehabilitation of stroke subjects, 12MWT was found to have the best sensitivity to change over the course of the inpatient rehabilitation stay (Citation44).
The predictive value of an impaired DLco for an accelerated deterioration in 12MWD is a novel finding. This, together with the recent reports on the predictive value of impaired DLco for COPD mortality and exacerbations (Citation18, Citation45), implies that DLco should be used to a greater extent in the routine evaluation of patients with COPD. This could also give relevant clinical information in order to initiate a more integrated approach to the patient's cardiopulmonary status and possibly identify treatable underlying conditions, such as coexistent heart failure (Citation46). Even if performance of DLco-measurements is still confined to expensive devices, available only in respiratory physiological laboratories, the appearance of newer, portable devices (Citation47) might open for a broader clinical use of DLco-measurements in the COPD outpatients’ clinic.
In conclusion, our findings indicate that the DLco-measurements, along with standard assessment of airway obstruction, give a more comprehensive assessment of the functional exercise capacity in patients with COPD at a specific time point, and also have a prognostic value for deterioration of exercise capacity.
Abbreviations | ||
12MWT = | = | 12-minute walk test |
12MWD = | = | 12-minute walk distance |
6MWT = | = | 6-minute walk test |
BMI = | = | Body mass index |
COPD = | = | Chronic obstructive pulmonary disease |
DLco = | = | Diffusing capacity of the lung for carbon monoxide |
FEV1 = | = | Forced expiratory volume in one second |
FRC = | = | Functional residual capacity |
FVC = | = | Forced vital capacity |
IC = | = | Inspiratory capacity |
Raw = | = | Airway resistance |
RV = | = | Residual volume |
SD = | = | Standard deviation |
TLC = | = | Total Lung Capacity |
VC = | = | Vital capacity |
Declaration of Interests Statement
This study was supported financially by the Swedish Heart & Lung Foundation. The authors alone are responsible for the content and writing of the paper.
References
- Decramer M, Janssens W, Miravitlles M. Chronic obstructive pulmonary disease. Lancet 2012; 379(9823):1341–1351.
- Klein JS, Gamsu G, Webb WR, Golden JA, Muller NL. High-resolution CT diagnosis of emphysema in symptomatic patients with normal chest radiographs and isolated low diffusing capacity. Radiology 1992; 182(3):817–821.
- Jones PW, Agusti AG. Outcomes and markers in the assessment of chronic obstructive pulmonary disease. Eur Respir J 2006; 27(4):822–832.
- Cooper CB. Exercise in chronic pulmonary disease: limitations and rehabilitation. Med Sci Sports Exerc 2001; 33(7 Suppl):S643–646.
- Pauwels RA, Buist AS, Calverley PM, Jenkins CR, Hurd SS. Global strategy for the diagnosis, management, and prevention of chronic obstructive pulmonary disease. NHLBI/WHO Global Initiative for Chronic Obstructive Lung Disease (GOLD) Workshop summary. Am J Respir Crit Care Med 2001; 163(5):1256–1276.
- Pepin V, Saey D, Whittom F, LeBlanc P, Maltais F. Walking versus cycling: sensitivity to bronchodilation in chronic obstructive pulmonary disease. Am J Respir Crit Care Med 2005; 172(12):1517–1522.
- McGavin CR, Gupta SP, McHardy GJ. Twelve-minute walking test for assessing disability in chronic bronchitis. Br Med J 1976; 1(6013):822–823.
- Bernstein ML, Despars JA, Singh NP, Avalos K, Stansbury DW, Light RW. Reanalysis of the 12-minute walk in patients with chronic obstructive pulmonary disease. Chest 1994; 105(1):163–167.
- Butland RJ, Pang J, Gross ER, Woodcock AA, Geddes DM. Two-, six-, and 12-minute walking tests in respiratory disease. Br Med J (Clin Res Ed) 1982; 284(6329):1607–1608.
- Cote CG, Pinto-Plata V, Kasprzyk K, Dordelly LJ, Celli BR. The 6-min walk distance, peak oxygen uptake, and mortality in COPD. Chest 2007; 132(6):1778–1785.
- Polkey MI, Spruit MA, Edwards LD, Watkins ML, Pinto-Plata V, Vestbo J, Calverley PM, Tal-Singer R, Agusti A, Bakke PS, Coxson HO, Lomas DA, MacNee W, Rennard S, Silverman EK, Miller BE, Crim C, Yates J, Wouters EF, Celli B; Evaluation of COPD Longitudinally to Identify Predictive Surrogate Endpoints (ECLIPSE) Study Investigators. Six-minute-walk test in chronic obstructive pulmonary disease: minimal clinically important difference for death or hospitalization. Am J Respir Crit Care Med 2013; 187(4):382–386.
- Morrison NJ, Abboud RT, Ramadan F, Miller RR, Gibson NN, Evans KG, Nelems B, Müller NL. Comparison of single breath carbon monoxide diffusing capacity and pressure-volume curves in detecting emphysema. Am Rev Respir Dis 1989; 139(5):1179–1187.
- Minai OA, Chaouat A, Adnot S. Pulmonary hypertension in COPD: epidemiology, significance, and management: pulmonary vascular disease: the global perspective. Chest 2010; 137(6 Suppl):39S–51S.
- Holguin F, Folch E, Redd SC, Mannino DM. Comorbidity and mortality in COPD-related hospitalizations in the United States, 1979 to 2001. Chest 2005; 128(4):2005–2011.
- Farkhooy A, Janson C, Arnardottir RH, Malinovschi A, Emtner M, Hedenstrom H. Impaired carbon monoxide diffusing capacity is the strongest predictor of exercise intolerance in COPD. COPD 2013; 10(2):180–185.
- Cockayne DA, Cheng DT, Waschki B, Sridhar S, Ravindran P, Hilton H, et al. Systemic biomarkers of neutrophilic inflammation, tissue injury and repair in COPD patients with differing levels of disease severity. PLoS One 2012; 7(6):e38629.
- Owens GR, Rogers RM, Pennock BE, Levin D. The diffusing capacity as a predictor of arterial oxygen desaturation during exercise in patients with chronic obstructive pulmonary disease. N Engl J Med 1984; 310(19):1218–1221.
- Boutou AK, Shrikrishna D, Tanner RJ, Smith C, Kelly JL, Ward SP, et al. Lung function indices for predicting mortality in chronic obstructive pulmonary disease. Eur Respir J. 2013; 42(3):616–625.
- Arnardottir RH, Sorensen S, Ringqvist I, Larsson K. Two different training programmes for patients with COPD: a randomised study with 1-year follow-up. Respir Med 2006; 100(1):130–139.
- Arnardottir RH, Boman G, Larsson K, Hedenstrom H, Emtner M. Interval training compared with continuous training in patients with COPD. Respir Med. 2007; 101(6):1196–1204.
- Hedenstrom H, Malmberg P, Agarwal K. Reference values for lung function tests in females. Regression equations with smoking variables. Bull Eur Physiopathol Respir 1985; 21(6):551–557.
- Hedenstrom H, Malmberg P, Fridriksson HV. Reference values for lung function tests in men: regression equations with smoking variables. Ups J Med Sci 1986; 91(3):299–310.
- Macintyre N, Crapo RO, Viegi G, Johnson DC, van der Grinten CP, Brusasco V, et al. Standardisation of the single-breath determination of carbon monoxide uptake in the lung. Eur Respir J 2005; 26(4):720–735.
- Miller MR, Hankinson J, Brusasco V, Burgos F, Casaburi R, Coates A, et al. Standardisation of spirometry. Eur Respir J 2005; 26(2):319–338.
- Wanger J, Clausen JL, Coates A, Pedersen OF, Brusasco V, Burgos F, et al. Standardisation of the measurement of lung volumes. Eur Respir J 2005; 26(3):511–522.
- Carter R, Holiday DB, Nwasuruba C, Stocks J, Grothues C, Tiep B. 6-minute walk work for assessment of functional capacity in patients with COPD. Chest 2003; 123(5):1408–1415.
- O’Donnell DE, Revill SM, Webb KA. Dynamic hyperinflation and exercise intolerance in chronic obstructive pulmonary disease. Am J Respir Crit Care Med 2001; 164(5):770–777.
- Oga T, Nishimura K, Tsukino M, Sato S, Hajiro T, Mishima M. Exercise capacity deterioration in patients with COPD: longitudinal evaluation over 5 years. Chest 2005; 128(1):62–69.
- Cooper CB, Celli BR, Jardim JR, Wise RA, Legg D, Guo J, Kesten S. Treadmill endurance during 2-year treatment with tiotropium in patients with COPD: a randomized trial. Chest 2013; 144(2):490–497.
- Burge PS, Calverley PM, Jones PW, Spencer S, Anderson JA, Maslen TK. Randomised, double blind, placebo controlled study of fluticasone propionate in patients with moderate to severe chronic obstructive pulmonary disease: the ISOLDE trial. Br Med J 2000; 320(7245):1297–1303.
- Kiakouama L, Cottin V, Glerant JC, Bayle JY, Mornex JF, Cordier JF. Conditions associated with severe carbon monoxide diffusion coefficient reduction. Respir Med 2011; 105(8):1248–1256.
- Dransfield MT, Washko GR, Foreman MG, Estepar RS, Reilly J, Bailey WC. Gender differences in the severity of CT emphysema in COPD. Chest 2007; 132(2):464–470.
- Shaker SB, Stavngaard T, Hestad M, Bach KS, Tonnesen P, Dirksen A. The extent of emphysema in patients with COPD. Clin Respir J 2009; 3(1):15–21.
- Kirby M, Owrangi A, Svenningsen S, Wheatley A, Coxson HO, Paterson NA, McCormack DG, Parraga G. On the role of abnormal DLCO in ex-smokers without airflow limitation: symptoms, exercise capacity and hyperpolarised helium-3 MRI. Thorax 2013; 68(8):752–759.
- Iversen KK, Kjaergaard J, Akkan D, Kober L, Torp-Pedersen C, Hassager C, Vestbo J, Kjoller E, and the ECHOS-Lung Function Study Group. Chronic obstructive pulmonary disease in patients admitted with heart failure. J Intern Med 2008; 264(4):361–369.
- Vestbo J, Edwards LD, Scanlon PD, Yates JC, Agusti A, Bakke P, Calverley PM, Celli B, Coxson HO, Crim C, Lomas DA, MacNee W, Miller BE, Silverman EK, Tal-Singer R, Wouters E, Rennard SI, ECLIPSE Investigators. Changes in forced expiratory volume in 1 second over time in COPD. N Engl J Med. 2011;365(13):1184–92.
- Teixeira PJ, Costa CC, Berton DC, Versa G, Bertoletti O, Canterle DB. [Six-minute walk work is not correlated to the degree of airflow obstruction in patients with Chronic Obstructive Pulmonary Disease (COPD)]. Rev Port Pneumol 2006; 12(3):241–254.
- Fujimoto H, Asai K, Watanabe T, Kanazawa H, Hirata K. Association of six-minute walk distance (6MWD) with resting pulmonary function in patients with chronic obstructive pulmonary disease (COPD). Osaka City Med J 2011; 57(1):21–29.
- Mohsenifar Z, Lee SM, Diaz P, Criner G, Sciurba F, Ginsburg M, Wise RA. Single-breath diffusing capacity of the lung for carbon monoxide: a predictor of PaO2, maximum work rate, and walking distance in patients with emphysema. Chest 2003; 123(5):1394–1400.
- Oga T, Nishimura K, Tsukino M, Sato S, Hajiro T. Analysis of the factors related to mortality in chronic obstructive pulmonary disease: role of exercise capacity and health status. Am J Respir Crit Care Med 2003; 167(4):544–549.
- Hill K, Dolmage TE, Woon L, Coutts D, Goldstein R, Brooks D. Comparing peak and submaximal cardiorespiratory responses during field walking tests with incremental cycle ergometry in COPD. Respirology 2012; 17(2):278–284.
- Pinto-Plata VM, Cote C, Cabral H, Taylor J, Celli BR. The 6-min walk distance: change over time and value as a predictor of survival in severe COPD. Eur Respir J 2004; 23(1):28–33.
- Martinez FJ, Foster G, Curtis JL, Criner G, Weinmann G, Fishman A, DeCamp MM, Benditt J, Sciurba F, Make B, Mohsenifar Z, Diaz P, Hoffman E, Wise R; NETT Research Group. Predictors of mortality in patients with emphysema and severe airflow obstruction. Am J Respir Crit Care Med 2006; 173(12):1326–1334.
- Kosak M, Smith T. Comparison of the 2-, 6-, and 12-minute walk tests in patients with stroke. J Rehabil Res Dev 2005; 42(1):103–107.
- Motegi T, Jones RC, Ishii T, Hattori K, Kusunoki Y, Furutate R, Yamada K, Gemma A, Kida K. A comparison of three multidimensional indices of COPD severity as predictors of future exacerbations. Int J Chron Obstruct Pulmon Dis 2013; 8:259–271.
- de Miguel Diez J, Chancafe Morgan J, Jimenez Garcia R. The association between COPD and heart failure risk: a review. Int J Chron Obstruct Pulmon Dis. 2013; 8:305–312.
- Buess C, Lehnigk B, Magnussen H. Comparison of two systems for measuring the carbon monoxide diffusing capacity of the lung. Am J Respir Crit Care Med. 2010; 181(1_MeetingAbstracts):A6489.