Abstract
In the next decade, Chronic Obstructive Pulmonary Disease (COPD) will be a major leading cause of death worldwide. Impaired muscle function and mass are common systemic manifestations in COPD patients and negatively influence survival. Respiratory and limb muscles are usually affected in these patients, thus contributing to poor exercise tolerance and reduced quality of life (QoL). Muscles from the lower limbs are more severely affected than those of the upper limbs and the respiratory muscles. Several epidemiological features of COPD muscle dysfunction are being reviewed. Moreover, the most relevant etiologic factors and biological mechanisms contributing to impaired muscle function and mass loss in respiratory and limb muscles of COPD patients are also being discussed. Currently available therapeutic strategies such as different modalities of exercise training, neuromuscular electrical and magnetic stimulation, respiratory muscle training, pharmacological interventions, nutritional support, and lung volume reduction surgery are also being reviewed, all applied to COPD patients. We claim that body composition and quadriceps muscle strength should be routinely explored in COPD patients in clinical settings, even at early stages of their disease. Despite the progress achieved over the last decade in the description of this relevant systemic manifestation in COPD, much remains to be investigated. Further elucidation of the molecular mechanisms involved in muscle dysfunction, muscle mass loss and poor anabolism will help design novel therapeutic targets. Exercise and muscle training, alone or in combination with nutritional support, is undoubtedly the best treatment option to improve muscle mass and function and QoL in COPD patients.
Introduction
In the next decade, Chronic Obstructive Pulmonary Disease (COPD) will be a major leading cause of death worldwide (Citation1–4). Muscle dysfunction, defined as the loss of either strength or endurance properties of muscles, is a major co-morbidity in COPD that results in the impairment of the patients’ exercise capacity and quality of life (Citation5). Other co-morbidities such as chronic heart failure, malnutrition, muscle wasting, exacerbations, and reduced physical activity or immobilization may further deteriorate muscle strength and endurance in those patients.
In COPD, although skeletal muscle dysfunction may affect both respiratory and limb muscles, the latter are in general more severely affected. In this regard, quadriceps muscle dysfunction and reduced muscle mass as measured by mid-thigh cross-sectional area were, indeed, shown to negatively influence survival and COPD mortality (Citation6,7). On the other hand, respiratory muscle dysfunction may contribute to hypercapnic respiratory failure, exercise limitation, increased risk for acute exacerbations, and eventual death (Citation8). On these grounds, muscle dysfunction appears to be a major systemic manifestation in COPD, whose potential identification should be included in the routine assessment of these patients in the clinic.
In an attempt to make health-care professionals become more aware of the magnitude of the problem, the current article provides the clinician with a general overview on the existing evidence of this co-morbidity, while describing the most relevant etiologic factors and biological mechanisms clearly involved in the pathophysiology of this co-morbidity. Additionally, currently available therapeutic options for both respiratory and limb muscle dysfunction in COPD are briefly described in the present review. Finally, it should also be mentioned that specific guidance on how to evaluate or classify muscle dysfunction in clinical settings is not being provided herein, since these aspects were extensively discussed in the latest version of the American Thoracic Society (ATS)/European Respiratory Society (ERS) statement on limb muscle dysfunction in COPD by expert authors (Citation9).
Key definitions in COPD muscle dysfunction
The function of a muscle is largely dependent on the physiological properties of its components, especially the fibers. Their speed of contraction and predominant type of metabolism, which in turn, determines their resistance to fatigue, are the most relevant physiologic features. Type I fibers are slow-twitch, have a predominantly oxidative metabolism, and are very resistant to fatigue. Type IIx are fast-twitch fibers, have an anaerobic metabolism, and are low fatigue-resistant. Type IIa fibers exhibit intermediate physiologic and metabolic profiles. Moreover, the relationships among the different physiologic variables of a muscle, namely those described between force and contraction velocity, stimulation frequency, or muscle length, also contribute to the correct muscle performance.
Nonetheless, in clinical settings, muscle function is evaluated through the “in vivo” measurements of the muscle properties. In this regard, strength, defined as the ability of the muscle to develop maximal force, and endurance or the ability to sustain a submaximal force over time, are the two properties commonly assessed in actual patients. In COPD, muscle dysfunction is characterized by a decline in either strength or endurance of the affected muscles (Citation5). The strength of a muscle is mainly determined by its size, while nutritional availability (glycogen stored in the muscle) is the main contributor to endurance. Other factors such as capillary density, oxygen delivery, and the oxidative capacity of the myofibers may also play a role in muscle performance in patients.
COPD patients may experience weakness of the lower limb muscles (Citation10;Citation11), which is commonly defined as a decline in the ability to generate force. In clinical and experimental settings, the force of a muscle group is typically quantified using a dynamometer, thus reductions in muscle quadriceps force may help predict weakness (Citation7,Citation11). Prolonged and strong contractions may lead to muscle fatigue, which increases in direct proportions to the rate of nutritional depletion. Muscle fatigue, defined as the temporary failure of the muscle to perform a task, may result from inability of the metabolic and contractile processes to continue supplying the same work output.
Therefore, fatigue may also be defined as a transient failure to generate force following loaded muscle contractions (Citation12,13). In fatigue, specific neurophysiological and mechanical parameters are altered, while they return to normality by rest (Citation14). Importantly, weakness and fatigue may also coexist within the same COPD patient as a weak muscle will become more rapidly fatigued (Citation14). Moreover, loss of muscle force and susceptibility to fatigue may improve in response to interventions such as strength exercise training and specific nutritional support administered to patients with COPD (Citation15) (see below for further details).
Epidemics of muscle dysfunction in COPD
Poor muscle function and mass loss are common systemic manifestations in COPD patients. Respiratory and limb muscles are usually affected in these patients, thus contributing to ventilatory constraints, poor exercise tolerance, and reduced quality of life (Citation16). As such, in a European-based study, it was estimated that in one third of the COPD patients, even at very early stages of their disease, quadriceps muscle dysfunction was an apparent manifestation (Citation11). Specifically, the force generated by the quadriceps of the patients was 25% lower in average than that produced by the age-matched healthy control subjects in the study (Citation11).
A multiple linear regression model was estimated to calculate the number of patients that exhibited quadriceps weakness (reduced quadriceps force) on the basis of a coefficient of reduction in muscle strength (Citation11). Importantly, the proportion of patients with muscle weakness was identical in the different participating countries regardless of the patients’ airway obstruction (Citation11). In line with this, the prevalence of muscle weakness among the patients did not significantly correlate with disease severity as measured by Global Initiative for COPD (GOLD) stages, while that prevalence was significantly greater in patients showing higher BODE (body mass index, airflow obstruction, dyspnea, exercise capacity) and dyspnea scores (Citation11).
In another investigation (Citation17), quadriceps strength and rectus femoris cross-sectional area were also significantly reduced (19%–25%) in a cohort of 161 COPD patients that were evenly distributed from GOLD stage I to stage IV compared to a population of age-matched healthy controls. Peripheral muscle endurance was also shown to be impaired in COPD patients, even in those with relatively normal physical activity and mild-to-moderate airflow obstruction (Citation18). Importantly, endurance decrease was associated with an early onset of muscle fatigue in the patients, which could not be predicted from their airflow limitation or quadriceps muscle weakness (reduced strength) (Citation18).
On the other hand, the prevalence of respiratory and diaphragm muscle dysfunction, although not fully elucidated in large-cohort studies, has also been shown to be of relevance in different groups of patients with advanced COPD (20%–30% reduction) compared to age-matched healthy controls (Citation19–23). Importantly, respiratory and limb muscle dysfunction may be further impaired as a result of repeated acute exacerbations as these negatively influence muscle mass and function in COPD (Citation24). Finally, poor muscle mass and lower limb muscle dysfunction, as assessed by mid-thigh cross-sectional area and quadriceps weakness, respectively, were also shown to strongly influence exercise capacity and quality of life (Citation16,Citation25,Citation26), to increase health care use (Citation27), and to predict survival and mortality in patients with COPD (Citation6,7).
Evidence of COPD muscle dysfunction
Limb muscle dysfunction
Peripheral muscles of COPD patients exhibit greater susceptibility to fatigue than age-matched healthy subjects (Citation18,Citation28), as well as in response to high-intensity cycling exercise (Citation29,30). Interestingly, the gastrocnemius and tibialis anterior muscles were also shown to be more prone to fatigue during normal walking in COPD patients (Citation31,32). As abovementioned, strength and endurance properties are impaired in the lower limb muscles of patients with COPD. Despite that strength is not the most sensitive measure to evaluate muscle function, its usefulness in clinical settings is justified by its accessibility that enables the clinician to easily diagnose skeletal muscle dysfunction in COPD. Importantly, the outcome of muscle strength measurements varies widely depending on the type of tests employed (Citation9): voluntary maneuvers, in which the patient's cooperation is of crucial importance, as opposed to non-voluntary maneuvers in which the patient's collaboration is not relevant.
Non-volitional assessment of muscle strength is characterized by the application of a single electrical or magnetic stimulus of a peripheral nerve of the given muscle (twitch force) in the patients (Citation9). Interestingly, this modality of muscle strength evaluation also enables the clinicians to determine in vivo force-frequency curves, in which force is recorded at different stimulation frequencies (high and low), by electrical or magnetic stimulation of the motor nerve in the patients (Citation9), thus enabling to define high- and low-frequency fatigue, respectively. Despite that the assessment of muscle endurance may be more sensitive to facilitate the design of tailored interventions in COPD patients, its use in clinical settings is limited, probably as a result of a lack of standardized protocols or reference values. Compared to healthy controls, peripheral muscle endurance was reduced in COPD patients, even in those with mild-to-moderate airway obstruction and was independent of lung function or physical activity parameters (Citation18).
Nevertheless, endurance of the upper limb muscles was well preserved (Citation33,34), suggesting that limb muscle abnormalities seem to be heterogeneous in COPD patients, and probably related to the activity of the muscle groups. Finally, lower limb muscle function as measured by quadriceps strength may be further impaired by exacerbations in COPD patients (Citation35–37), which negatively influence muscle mass and exercise tolerance even one month after hospital discharge (Citation35).
Respiratory muscle dysfunction
In COPD, despite that the respiratory muscles undergo a positive adaptation (training-like effect) that renders them more fatigue-resistant (Citation14,Citation21,Citation38), maximal inspiratory and expiratory pressures (strength) and endurance of these muscles are frequently reduced in the patients (Citation14,Citation19,Citation21–23,Citation39,40) (See following section for specific details on the etiology and mechanisms). Respiratory muscle dysfunction imposes ventilatory constraints to the patients that may worsen the underlying chronic respiratory failure frequently present in COPD patients, especially in advanced stages. Furthermore, respiratory muscle dysfunction constitutes an important factor accounting for an increased risk of hospital readmissions (Citation8).
On the other hand, acute exacerbations also impair respiratory muscle function as they severely affect whole body muscle mass (Citation24,Citation35). Indeed, exacerbations could be considered as one of the most relevant systemic contributors that seem to equally affect both limb and respiratory muscles in patients with COPD (Citation24). Additionally, other factors such as the need of the respiratory muscles to overcome the inspiratory loads imposed by the increased ventilatory demands occurring during COPD acute exacerbations further impair respiratory muscle function in these patients. Also, changes in lung volume during exacerbations poses the diaphragm and the rib cage in a mechanical disadvantage, which will further deteriorate the function of other respiratory muscles such as the intercostal muscles (Citation24).
Etiologic factors and biological mechanisms of muscle dysfunction in COPD
Several factors and biological mechanisms have been shown to participate in the multifactorial etiology of respiratory and limb muscle dysfunction in COPD. Cigarette smoke, hypoxia, hypercapnia and acidosis, metabolic alterations of several types, malnutrition, genetics, systemic inflammation, aging, co-morbidities, concomitant treatments, exacerbations, and inactivity are counted among the most relevant etiologic factors that contribute to muscle dysfunction in COPD patients through the action of several biological mechanisms.
The specific contribution of biological events and pathways to both respiratory and limb muscle dysfunction has been extensively discussed in different comprehensive reports (Citation9,Citation14,Citation21,Citation41) as well as in more specific reviews that were mainly focused on the discussion of structural abnormalities (Citation42), muscle remodeling (Citation21), oxidative stress and muscle wasting (Citation43), epigenetic regulation of muscle mass and function (Citation44,45), autophagy (Citation46), and metabolic derangements (Citation47) together with the influence of exercise training (Citation48) and exacerbations (Citation24). A brief outline of the specific role played by the most relevant etiologic factors and biological mechanisms involved in COPD muscle dysfunction follows.
Limb muscle dysfunction
As aforementioned, muscles from the lower limbs are usually more severely affected than those of the upper limbs and the respiratory muscles. In patients with COPD, most of the studies have focused so far on the analyses of the vastus lateralis of the quadriceps. Whether the same findings could be observed in other muscles of the lower limbs remains unknown. However, as the quadriceps is a major locomotor muscle and is easily accessible, it has been chosen as the best option for the studies aimed at exploring the mechanisms involved in the limb muscle dysfunction and exercise intolerance of COPD patients. Hitherto several etiologic factors that lead to a deconditioned phenotype of the lower limb muscles in COPD have been identified. In Figure , the contributing factors shown to negatively influence muscle mass and function are being represented.
Figure 1. (A) Several etiologic factors involved in the multifactorial etiology of muscle dysfunction exert deleterious effects on the function and mass (deconditioning) of lower limb muscles in patients with COPD. Hence, the balance in the scale is completely skewed towards the negative side (left-hand side tray in the scale). (B) In lower limb muscles, several biological events that mediate the actions of the etiologic factors exert direct deleterious effects on muscle function, structure, and mass. Hence, the balance in the scale is completely skewed towards the negative side (left-hand side tray in the scale). (C) Schematic representation on how the different reported etiologic factors contribute to lower muscle dysfunction in COPD through the action of the reported biological events that directly modify muscle phenotype and function in the patients.
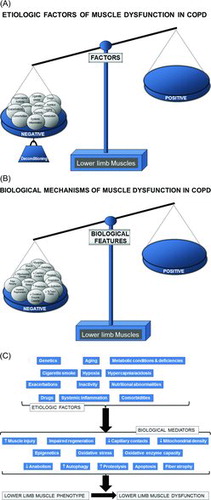
Cigarette smoke, genetics, hypoxia, hypercapnia and acidosis, metabolic derangements including vitamin D and testosterone deficiencies, drugs (systemic corticosteroids), other co-morbidities, exacerbations, systemic inflammation, nutritional abnormalities, reduced physical activity, and aging are all etiologic factors that clearly contribute to muscle dysfunction of the lower limbs in COPD (Citation9,14) (Figure ). Nevertheless, the predominance of physical inactivity (Citation17), nutritional abnormalities (Citation49), repeated exacerbations (Citation24,Citation36,Citation37,Citation50), and corticosteroids (Citation24,Citation51) should be underscored above the other factors as the main contributors to muscle function and mass impairment, especially in advanced COPD.
As illustrated in Figures and 1C, several molecular and cellular events take place in the lower limb muscles, which mediate the effects of the different etiologic factors in the patients, leading to modifications in the muscle phenotype and function. Studies demonstrating the contribution of each biological event in the lower limb muscle dysfunction of COPD patients are summarized as follows. A fiber-type switch towards a less fatigue-resistant (phenotype from slow-twitch to fast-twitch fibers) is a major feature in the vastus lateralis of patients with advanced COPD (Citation10;Citation52;Citation53). Furthermore, fast-twitch fibers are also of smaller size in patients with advanced COPD and poor muscle mass (Citation10), thus contributing to muscle weakness.
Additionally, factors such as aging (Citation54), malnutrition (Citation55), and systemic corticosteroid treatment (Citation56) may worsen the fast-twitch fiber atrophy observed in COPD. Besides, a reduction in the capillary numbers and contacts (Citation57,58) that could eventually impair oxygen delivery to the myofibers (Citation59–61) is another contributing biological mechanism. Other structural abnormalities such as muscle cell membrane and sarcomere damage may also negatively influence muscle function (Citation10,Citation42,Citation62). Mitochondrial derangements (Citation47) of several types including decreased density as a result of fewer proportions of slow-twitch fibers (Citation10,Citation47,Citation63), were also demonstrated to alter muscle function in COPD (Citation64–66) (Figures and 1C).
Oxidative stress, defined as the imbalance between oxidants and antioxidants, and as measured by several markers such as protein oxidation and nitration and lipid peroxidation has been consistently shown in blood and limb muscles of patients with severe COPD both at rest and after exercise (Citation10,Citation19,Citation22,Citation67–74). Importantly, oxidative stress markers were also shown to inversely correlate with several clinical and physiological parameters such as exercise capacity, body composition, and quadriceps strength of the patients (Citation10,Citation68) (Figures and 1C). Although oxidative stress and inflammation have been previously associated, evidence shows that local inflammatory events do not seem to occur in muscles of COPD patients (Citation10,Citation67,Citation69,Citation75), even in atrophying muscles or during exacerbations (Citation24,Citation50).
Chronic hypoxia induce a reduction in muscle mass probably as a result of the interaction of several molecular mediators such as inflammation (Citation76), hypoxia inducible factor-1 signaling pathway (Citation77), oxidative stress (Citation73,Citation79), and reduced oxidative enzyme capacity and capillary numbers (Citation79). Hypercapnia, which may worsen during exacerbations, may also play a role in COPD muscle dysfunction through acidosis as it enhances ubiquitin-proteasome proteolytic system activity (Citation80) and/or through a reduction in protein anabolism (Citation80,81) (Figures and 1C).
Cigarette smoke may also contribute to limb muscle dysfunction in COPD through several biological mechanisms such as decreased type I fiber sizes and proportions and reduced mitochondrial activity, while concomitantly causing an increase in oxidative stress levels in healthy smokers and experimental animal models of chronic exposure to cigarette smoke (Citation69,Citation82) (Figures and 1C). Other relevant molecular and cellular events that have been shown to mediate muscle mass loss and dysfunction in COPD from different etiologic factors are increased proteolysis (Citation10), apoptosis (Citation75), epigenetic mechanisms (Citation44,45,Citation83–85), and increased autophagy (Citation86) (Figures and 1C).
Respiratory muscle dysfunction
In COPD, since the respiratory muscles are chronically exposed to the inspiratory loads and must remain active throughout the existence of the patients, they are less severely affected than the lower limb muscles. In the last years, respiratory muscles have been studied through the analyses of the costal diaphragm, with very restricted access, and only via thoracotomy performed for clinical reasons (mainly lung cancer and lung volume reduction surgeries).
In COPD patients, modifications in ventilatory mechanics as a result of static pulmonary hyperinflation, which modifies thorax geometry and shortens the diaphragm length, displace the muscle away from its optimal length to generate the required forces (Citation14). Additionally, their respiratory muscles need to overcome the increased work of breathing resulting from the greater elastic, resistive, and threshold inspiratory loads imposed by airflow limitation (Citation14). These factors, which are inherent to the respiratory condition (ventilatory mechanics alterations), constitute the main contributors to respiratory muscle dysfunction in COPD (Figure ). However, it has also been shown that in COPD patients, the respiratory muscles somehow undergo a positive adaptation (training-like effect) that renders them, especially the diaphragm, more fatigue-resistant compared to diaphragm forces developed by healthy subjects when exposed to identical lung volumes (Citation14,Citation38) (Figure ).
Figure 2. A) In the respiratory muscles, etiologic local factors such as alterations in ventilatory mechanics: changes in thorax geometry and inspiratory overloads may somehow counterbalance (training-like effect, right-hand side tray in the scale) the deleterious effects of other factors of a rather systemic nature (left-hand side tray in the scale), as they also contribute to lower limb muscle dysfunction. B) In the respiratory muscles, several cellular and molecular mechanisms exert beneficial effects (adaptive mechanisms, right-hand side tray), which partly counterbalance the deleterious actions of other biological events (left-hand side tray). C) Schematic representation on how the different reported etiologic factors contribute to respiratory muscle dysfunction in COPD through the action of several biological mechanisms that modify muscle phenotype and function in the patients. Notice that in the COPD respiratory muscle dysfunction, as opposed to dysfunction of the lower limb muscles, several etiologic factors may exert beneficial effects (training-like effect, right-hand side panels) on muscle mass and performance through the action of different biological mediators (adaptive mechanisms) that lead to adaptation of the inspiratory muscles in COPD (all depicted in the right-hand side of the figure). These adaptive mechanisms partly counterbalance the deleterious effects of other factors and mechanisms of a rather systemic nature (depicted in the left-hand side of the figure).
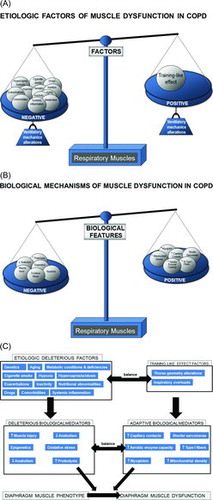
Finally, the same abovementioned etiologic factors for the lower limb muscles, may also affect, to different degrees, the respiratory muscles in COPD: Cigarette smoke, hypoxia, hypercapnia and acidosis, metabolic derangements, malnutrition, genetics, systemic inflammation, aging, co-morbidities, concomitant treatments, exacerbations, and reduced physical activity (Citation9,Citation14) (Figure ). However, in COPD respiratory muscle dysfunction, exacerbations, nutritional abnormalities, and aging are likely to play a key role above the other contributing factors (Citation14,Citation21,Citation49).
As shown in Figures and 2C, several molecular and cellular events that take place in the lower limb muscles also seem to mediate the effects of the different etiologic factors that negatively influence phenotype and function in the respiratory muscles. Nonetheless, specific cellular and molecular events also mediate beneficial effects (adaptive biological mediators) on the diaphragm of COPD patients (Citation14): shorter sarcomere length (Citation87), higher proportions of slow-twitch fibers and myoglobin content (Citation20,Citation88), increased capillary contacts per fiber (Citation88), increased mitochondrial density (Citation87), and enhanced aerobic enzyme capacity (Citation89,90) (Figures and 2C).
On this basis, the adaptive mechanisms may offset to certain extent the adverse phenotypic features in the diaphragm of patients with COPD. In these patients, the final respiratory muscle outcome will rely on the balance between deleterious and adaptive biological mediators (Figures -2C), which may also differ between stable and acute conditions within the same patient. Interestingly, in the external intercostal muscle of COPD patients, similar adaptive cellular events may also take place (Citation91–93). Nevertheless, in advanced COPD, biological mechanisms concomitantly affecting the lower limbs such as injury (Citation62), oxidative stress (Citation19,Citation22), enhanced proteolysis (Citation94) and reduced anabolism (Citation95), apoptosis (Citation75), and epigenetic events (Citation45,Citation85) will prevail over the adaptive mechanisms (Figures –2C).
Conclusively, in stable COPD, a fragile balance between adaptive and deleterious biological mechanisms exists in the respiratory muscles. However, the predominance of factors such as exacerbations, nutritional abnormalities, and aging would skew the balance towards a rather negative phenotype of these muscles, thus leading to ventilatory failure and eventual death of the patients (Citation14,Citation21,Citation24).
Interventions in COPD muscle dysfunction
Pulmonary rehabilitation is currently defined as a comprehensive intervention based on a thorough assessment of the patient using different therapies that include exercise training, education, and behavior change designed to improve the physical and psychosocial condition of people with chronic respiratory diseases and to promote the long-term adherence to health-enhancing behaviors (for review see reference (Citation96)). As aforementioned, exercise capacity is reduced in patients with COPD and it may be limited by dyspnea. Moreover, skeletal muscle dysfunction, age-related decline in function (Citation97), and physical deconditioning further impair exercise capacity in COPD patients (Citation96). Exercise training is nowadays considered to be the basis of pulmonary rehabilitation. Interestingly, it has been shown that even patients with advanced COPD can sustain the required training intensity and duration to achieve improvements in skeletal muscle function and performance (Citation58,Citation98).
Despite the absence of modifications in lung function parameters, exercise training leads to improvements in exercise tolerance in COPD (Citation99,100). Additionally, ventilatory requirements for a given submaximal work rate are also reduced in response to exercise training, as a result of increased oxidative capacity and efficiency of the skeletal muscle (Citation100,101). Exercise training may also induce positive effects on other areas (psychosocial), while reducing symptom burden and improving the cardiovascular system (Citation96). Needless to say, that the tailored exercise prescription will rely on the specific patient's baseline exercise capacity as well as on the absence of contraindications such as cardiovascular disease. Additionally, effectiveness of the training can be ensured by optimizing the patient's medical treatment, including long-term oxygen therapy and the control of co-morbidities (Citation96).
Finally, it should be underscored that for exercise training to be effective the training load should be higher than those of daily life activities to improve aerobic capacity and muscle strength and must progress as improvement takes place (Citation96). Depending on the target end-points to ameliorate, different modalities of training have been specifically designed so far in COPD patients: endurance exercise training, interval training, resistance training, neuromuscular stimulation, and respiratory muscle training [for extensive review, see reference (Citation96)]. In the current review, different training modalities with special attention to endurance exercise training, neuromuscular stimulation, and respiratory muscle training are briefly discussed below. Moreover, the different therapeutic interventions have been reviewed on the basis of the two groups of muscles, limb and respiratory, respectively.
Limb muscle dysfunction
Training modalities
Endurance exercise training is a major component of the pulmonary rehabilitation programs. It aims at improving cardiorespiratory fitness that is associated with a reduction in breathlessness and fatigue and at increasing the function of the muscles involved in ambulation (Citation96). Despite that high-intensity endurance exercise training programs are commonly used in pulmonary rehabilitation, low-intensity or interval training modalities are alternatives when the patients experience difficulty achieving the target intensity or duration (Citation96). In endurance exercise training programs, cycling or walking are the commonest applied exercise modalities. In order to improve exercise tolerance, muscle function, and bioenergetics, exercise training is applied at a frequency of three to five times a week, at an intensity that is greater than 60% maximal work rate, for 20 to 60 minutes per session in patients with COPD (Citation96). Moreover, a Rating of Perceived Exertion of 12 to 14 and a Borg dyspnea or fatigue score of 4 to 6 are considered to be a target training intensity (Citation102).
Interval training is an alternative to standard endurance exercise training in patients who experience symptoms (dyspnea or fatigue) or difficulties achieving the target intensity and/or duration. In interval training, high-intensity exercise periods are combined with others of lower-intensity exercise (Citation96). Interestingly, whole-body training is a modality that aims to improve cardiorespiratory endurance, muscle strength, and flexibility. It includes several types of exercise such as standard endurance exercise training, treadmill walking, weight lifting, and gymnastics. Importantly, whole-body training was shown to induce an anabolic response in COPD patients with preserved body composition, but the improvements in exercise tolerance and muscle performance were superior to those observed in muscle mass (Citation99). Resistance or strength training consisting of the repetitive lifting of heavy loads by local muscle groups was shown to improve muscle mass and strength of the peripheral muscles in patients with COPD (Citation25,Citation103). Improvements in muscle mass and strength are more largely attained in response to resistance training than to endurance exercise training (Citation33). Furthermore, patients experience less dyspnea during the exercise period than in programs of endurance exercise training (Citation96).
Nowadays, despite that small proportions of COPD patients may not respond to endurance exercise training, arguments against its benefits for the treatment of limb muscle dysfunction in COPD are scarce (Citation9,Citation48,Citation58,59,Citation96,Citation98,Citation104–108). Besides, evidence also shows that the size of all fiber types increases in the vastus lateralis in response to endurance exercise training of long duration (8–10 weeks) (Citation58,Citation105–108). Moreover, endurance exercise training also induced a fiber-type shift towards a more fatigue-resistant phenotype (increased slow-twitch fibers) (Citation58,Citation105–108) and an improvement in the oxidative capacity (Citation109) of the limb muscles in trained patients. Improvements in quadriceps muscle mass and strength were also achieved in response to exercise training in COPD (Citation110).
Importantly, the intensity and duration of the exercise training are key factors that may predict outcomes such as muscle oxidative capacity adaptations and prevent the potential development of oxidative stress. In fact, it was demonstrated that while the intensity of the exercise program results in changes in muscle fiber structure, the duration of the exercise training influences adaptation to muscle oxidative capacity and capillary growth (Citation68,Citation98,Citation105,Citation107,Citation108). In this regard, in COPD patients, it has been estimated that a minimum duration of 8-12 weeks of the endurance training program is required to achieve the beneficial effects in terms of exercise performance and muscle function of the lower limbs (Citation59,Citation98,Citation104,Citation111).
Furthermore, the magnitude of the response to exercise training (improvements in exercise capacity and muscle function) varies widely in patients with COPD (Citation112). In this regard, patients who developed contractile fatigue as measured by reductions in magnetic twitch quadriceps force during the exercise sessions were those exhibiting greater training effects in terms of quality of life and functional exercise capacity after the program (Citation28). These findings reinforce the concept that intensity is a major element of the exercise training outcome. Intensity is also a relevant parameter for the improvements seen in muscle function (strength) of the lower limbs to translate into maximal and submaximal exercise capacity and general physiologic adaptation in COPD patients (Citation96,Citation98,Citation111).
Finally, it has also been reported that training may prevent or reduce the impairment in muscle function and mass observed during the course of exacerbations (Citation24). On this basis, resistance training initiated during the second day of hospitalization was shown to counteract limb muscle dysfunction and exercise capacity of the patients at discharge (Citation113). Additionally, in the group of trained patients, the beneficial effects remained even one month after discharge (Citation113). In line with this, it has also been demonstrated that additional benefits can be attained by adding resistance training to a program of endurance exercise training in patients with COPD (Citation110,Citation112).
Other training modalities such as upper limb and flexibility training have also been the focus of research in the last decade. A systematic review (Citation114) on all forms of upper limb training was inconclusive about whether improvements in upper limb performance were satisfactory and to what extent they could entrain additional benefits in the patients’ quality of life or dyspnea involving daily living activities. Despite that flexibility training is a component of pulmonary rehabilitation programs its effectiveness has not yet been demonstrated in specific clinical trials conducted on COPD patients (Citation96).
Neuromuscular stimulation
Severe COPD patients with limited exercise capacity may experience difficulty participating in exercise training, especially in high-intensity endurance training programs. Transcutaneous electrical stimulation of skeletal muscles appears as an alternative rehabilitation modality in which muscles are trained without the exercise component. Electrical stimulation is applied following specific protocols in which the intensity (amplitude), frequency, and duration of the stimulus are selected to achieve the desired muscle contraction.
Importantly, many of the most significant drawbacks related to exercise training (dyspnea, minimal cardiocirculatory demands, and psychosocial aspects) are absent in electrical stimulation (Citation96). Therefore, this training modality is of interest in patients with severe exercise limitation and/or during exacerbations or respiratory failure (Citation115). Several studies have shown that dyspnea, exercise capacity, and limb muscle strength improved in response to neuromuscular electrical stimulation training in stable COPD outpatients with baseline severely reduced exercise tolerance (Citation115–117), as well as during exacerbations (Citation115,Citation118).
Interestingly, neuromuscular stimulation of the quadriceps muscle for six weeks was shown to improve muscle strength, and the size and anabolism of the muscle fibers in the vastus lateralis (Citation119). More recently, in a very elegant prospective study (DICES trial) (Citation120), it was demonstrated that strength training and high-frequency neuromuscular electrical stimulation were equally effective in improving quadriceps muscle strength and exercise capacity in COPD patients with severe airflow limitation, dyspnea, and muscle weakness.
Despite that neuromuscular electrical stimulation is in general well tolerated for training purposes it may also have several limitations, namely skin pain and reproducibility. On this basis, alternatives such as magnetic stimulation have recently emerged and may also be of interest and very useful in clinical settings (Citation121,122). In this regard, quadriceps magnetic stimulation training was shown to be well tolerated by the severe patients while improving muscle strength, exercise capacity, and the size of slow-twitch fibers (Citation121,Citation123,Citation124). Nonetheless, in order to better define the duration and intensity of the magnetic stimulation training as well as the target populations, studies including larger groups of COPD patients should still be conducted.
Anabolic stimulation
Patients with COPD are prone to nutritional depletion especially during exacerbations. Adequate nutritional support is absolutely required in these patients. Moreover, in order to ensure the effects on muscle mass and function, nutritional supplementation has also been the focus of research in COPD patients (Citation125,126). For instance, an intervention based on dietary food counseling and advice on food fortification for 6 months induced a gain in body weight, dyspnea scores, and quality of life parameters, but not in lung or skeletal muscle functions in outpatients with COPD and malnutrition (Citation126).
Moreover, increases in body weight, muscle mass, respiratory and limb muscle strength, exercise capacity, and quality of life were also seen in COPD inpatients with altered body composition in response to three oral liquid nutritional supplements administered together with an eight-week pulmonary rehabilitation program (Citation125). Nutritional supplementation seems effective especially in combination with an anabolic stimulus such as exercise training in patients with advanced COPD and poor body composition.
The line has also been put forward that several anabolic drugs and bioactive nutrients such as testosterone (Citation127), growth hormone and its analogues (Citation128), megestrol acetate (Citation129), L-carnitine (Citation130), creatine (Citation131), antioxidants (Citation132,133), and vitamin D supplements (Citation134) may help prevent muscle mass loss and dysfunction of limb muscles in COPD. Nonetheless, results are not yet conclusive and concerns regarding safety and potential adverse effects require evidence from larger clinical trials before any of these drugs can be actually implemented in clinical settings.
Respiratory muscle dysfunction
Respiratory muscle training
As abovementioned respiratoy muscle strength is commonly reduced in patients with COPD (Citation19,Citation22,Citation23,Citation25,Citation38). Both the perception of dyspnea and exercise tolerance are negatively influenced by the decreased pressure-generating capacity of the inspiratory muscles observed in these patients (Citation16,Citation38,Citation135). Despite the recognized beneficial effects of endurance exercise training, it does not seem to improve respiratory muscle strength in the patients probably because the ventilatory loads are not of sufficient magnitude to induce a training adaptation (Citation96,Citation100,Citation136). On this basis, in patients with severe respiratory muscle weakness, inspiratory muscle training in which specific inspiratory loads are applied to the patients appears as a good modality to reduce dyspnea and improve exercise capacity.
In general special devices that impose a threshold or resistive load are used in respiratory muscle training. In inspiratory muscle training programs for patients with COPD, a minimum load of 30% of their maximal inspiratory pressure is required in order to improve both respiratory muscle function and exercise tolerance (Citation40,Citation137,Citation138). Indeed, in all the studies, COPD patients reached a training intensity equal to 60% of their maximal inspiratory pressure to ensure a training effect (Citation40,Citation137,Citation138). In general, inspiratory muscle strength and endurance (Citation137,Citation139) and dyspnea (Citation139) were shown to improve in response to inspiratory muscle training as demonstrated in these meta-analyses reviews. Interestingly, inspiratory muscle training of high intensity improved the strength and endurance properties of the inspiratory muscles, while it also induced a significant increase in the proportions of slow-twitch fibers and in the size of the fast-twitch fibers in the external intercostal muscles of the trained patients (Citation40). Moreover, a specific program characterized by the training of the expiratory muscles of COPD patients significantly improved expiratory muscle function, their exercise capacity and quality of life (Citation140).
Despite that many different modalities of respiratory muscle training are commonly used, interval-training programs characterized by loaded breathing combined with resting periods seem to be adequately tolerated by the COPD patients, while allowing for the achievement of the desired improvements in maximal inspiratory pressure (Citation139,Citation141). Eventually, it is conceivable that respiratory muscle training added to a general exercise training program may benefit those patients with advanced COPD and significant inspiratory muscle weakness (Citation137,Citation139,Citation142). Nevertheless, more evidence based on the design of prospective future studies is absolutely required.
Lung volume reduction surgery (LVRS)
The national emphysema treatment trial (NETT) recently showed (Citation143) that the use of LVRS for the treatment of hyperinflation in emphysema improved lung function, exercise tolerance, and even survival in those patients with upper lobe-predominance disease. Importantly, inspiratory muscle force generation of the respiratory muscles, which was evaluated using several parameters of global respiratory muscle and diaphragm functions, was significantly improved in patients with severe emphysema one month after LVRS compared to baseline values (Citation144). The restoration of the chest geometry to a more normal function capacity certainly played a role in improving the ability of the inspiratory muscles to generate force (Citation144).
Pharmacological interventions
The calcium sensitizer levosimendan improves myocardial contractility in clinical settings. Interestingly, in vitro contractility studies of muscle fibers obtained from the diaphragm of patients with COPD and control subjects showed that levosimendan induced andimprovement in force generation by both slow- and fast-twitch fibers (Citation145). Moreover, in a randomized trial, treatment with levosimendan was demonstrated to induce several beneficial effects on diaphragm muscle function in healthy individuals exposed to inspiratory loading (Citation146).
Specifically, levosimendan treatment attenuated the loss on twitch diaphragm force observed after loading in the non-treated subjects, and improved neuro-mechanical efficiency and electrical activity of the diaphragm during loading in healthy individuals (Citation146). Other compounds such as treatment with the antioxidant N-acetylcysteine (NAC) was also shown to attenuate low-frequency diaphragm fatigue in healthy humans exposed to resistive breathing (Citation147). In keeping with, NAC also induced a decrease in oxidative stress levels in the diaphragm of dogs exposed to resistive breathing of a wide range of intensities, especially in those breathing against the highest loads (Citation132). Taken together, the findings suggest that these drugs could be used in clinical settings to treat patients with COPD and respiratory failure.
Concluding Remarks
In view of currently available knowledge, we claim that body composition and both respiratory and limb muscle strength should be routinely explored in COPD patients in clinical settings, even at early stages of their disease. In spite of the progress achieved over the last decade in the assessment of this relevant systemic manifestation of COPD patients, much remains to be investigated. Further elucidation of the molecular mechanisms involved in muscle dysfunction and mass loss and poor anabolism will shed light into new avenues of research that will help design novel therapeutic targets. Until effective and safer pharmacological therapies emerge, exercise and muscle training modalities, alone or in combination with nutritional support, are undoubtedly the best treatment options to improve muscle mass and function, and quality of life in COPD patients.
Declaration of Interest Statement
The authors declare that they have no conflict of interest, financial or otherwise in relation to this manuscript. The authors alone are responsible for the content and writing of the paper.
Acknowledgments
The authors gratefully acknowledge Ester Puig-Vilanova for her help with the reference list. CIBERES, FIS 11/02029, FIS 12/02534, SAF-2011-26908, 2009-SGR-393; SEPAR 2010, SEPAR 2014, FUCAP 2009, FUCAP 2011, FUCAP 2012, and Marató TV3 (MTV3-07-1010) (Spain) have contributed to support part of the research described in this review. Dr. Esther Barreiro was a recipient of the ERS COPD Research Award 2008.
References
- Miravitlles M, Soler-Cataluna JJ, Calle M, Molina J, Almagro P, Quintano JA, Riesco JA, Trigueros JA, Pinera P, Simon A, Lopez-Campos JL, Soriano JB, et al. Spanish COPD Guidelines (GesEPOC): pharmacological treatment of stable COPD. Spanish Society of Pulmonology and Thoracic Surgery. Arch Bronconeumol 2012; 48(7):247–257.
- Miravitlles M, Calle M, Soler-Cataluna JJ. Clinical phenotypes of COPD: identification, definition and implications for guidelines. Arch Bronconeumol 2012; 48(3):86–98.
- Miravitlles M, Soler-Cataluna JJ, Calle M, Molina J, Almagro P, Antonio QJ, Antonio RJ, Antonio TJ, Pinera P, Simon A, Rodriguez-Hermosa JL, Marco E, et al. Spanish Guideline for COPD (GesEPOC). Update 2014. Arch Bronconeumol 2014; 50 Suppl 1:1–16.
- Vestbo J, Hurd SS, Agustí AG, Jones PW, Vogelmeier C, Anzueto A, Barnes PJ, Fabbri LM, Martinez FJ, Nishimura M, Stockley RA, Sin DD, Rodriguez-Roisin R. Global strategy for the diagnosis, management, and prevention of chronic obstructive pulmonary disease: GOLD executive summary. Am J Respir Crit Care Med 2013; 187(4):347–365.
- Martínez-Llorens J, Ausín P, Roig A, Balañá A, Admetlló M, Muñoz L, Gea J. Nasal inspiratory pressure: an alternative for the assessment of inspiratory muscle strength? Arch Bronconeumol 2011; 47(4):169–175.
- Marquis K, Debigare R, Lacasse Y, LeBlanc P, Jobin J, Carrier G, Maltais F. Midthigh muscle cross-sectional area is a better predictor of mortality than body mass index in patients with chronic obstructive pulmonary disease. Am J Respir Crit Care Med 2002; 166(6):809–813.
- Swallow EB, Reyes D, Hopkinson NS, Man WD, Porcher R, Cetti EJ, Moore AJ, Moxham J, Polkey MI. Quadriceps strength predicts mortality in patients with moderate to severe chronic obstructive pulmonary disease. Thorax 2007; 62(2):115–120.
- Vilaro J, Ramirez-Sarmiento A, Martinez-Llorens JM, Mendoza T, Alvarez M, Sanchez-Cayado N, Vega A, Gimeno E, Coronell C, Gea J, Roca J, Orozco-Levi M. Global muscle dysfunction as a risk factor of readmission to hospital due to COPD exacerbations. Respir Med 2010; 104(12):1896-902.
- Maltais F, Decramer M, Casaburi R, Barreiro E, Burelle Y, Debigaré R, Dekhuijzen PN, Franssen F, Gayan-Ramirez G, Gea J, Gosker HR, Gosselink R, Hayot M, Hussain SN, Janssens W, Polkey MI, Roca J, Saey D, Schols AM, Spruit MA, Steiner M, Taivassalo T, Troosters T, Vogiatzis I, Wagner PD; ATS/ERS Ad Hoc Committee on Limb Muscle Dysfunction in COPD. An official American Thoracic Society/European Respiratory Society statement: update on limb muscle dysfunction in chronic obstructive pulmonary disease. Am J Respir Crit Care Med 2014; 189(9):e15-e62.
- Fermoselle C, Rabinovich R, Ausin P, Puig-Vilanova E, Coronell C, Sanchez F, Roca J, Gea J, Barreiro E. Does oxidative stress modulate limb muscle atrophy in severe COPD patients? Eur Respir J 2012; 40(4):851–862.
- Seymour JM, Spruit MA, Hopkinson NS, Natanek SA, Man WD, Jackson A, Gosker HR, Schols AM, Moxham J, Polkey MI, Wouters EF. The prevalence of quadriceps weakness in COPD and the relationship with disease severity. Eur Respir J 2010; 36(1):81–88.
- Janaudis-Ferreira T, Wadell K, Sundelin G, Lindstrom B. Thigh muscle strength and endurance in patients with COPD compared with healthy controls. Respir Med 2006; 100(8):1451–1457.
- Luo YM, Hart N, Mustfa N, Lyall RA, Polkey MI, Moxham J. Effect of diaphragm fatigue on neural respiratory drive. J Appl Physiol 2001; 90(5):1691–1699.
- Gea J, Agusti A, Roca J. Pathophysiology of muscle dysfunction in COPD. J Appl Physiol 2013; 114(9):1222-34.
- Mador MJ, Kufel TJ, Pineda LA, Steinwald A, Aggarwal A, Upadhyay AM, Khan MA. Effect of pulmonary rehabilitation on quadriceps fatiguability during exercise. Am J Respir Crit Care Med 2001; 163(4):930–935.
- Gosselink R, Troosters T, Decramer M. Peripheral muscle weakness contributes to exercise limitation in COPD. Am J Respir Crit Care Med 1996; 153(3):976–980.
- Shrikrishna D, Patel M, Tanner RJ, Seymour JM, Connolly BA, Puthucheary ZA, Walsh SL, Bloch SA, Sidhu PS, Hart N, Kemp PR, Moxham J, et al. Quadriceps wasting and physical inactivity in patients with COPD. Eur Respir J 2012; 40(5):1115–1122.
- Coronell C, Orozco-Levi M, Mendez R, Ramirez-Sarmiento A, Galdiz JB, Gea J. Relevance of assessing quadriceps endurance in patients with COPD. Eur Respir J 2004; 24(1):129–136.
- Barreiro E, de la Puente B, Minguella J, Corominas JM, Serrano S, Hussain SN, Gea J. Oxidative stress and respiratory muscle dysfunction in severe chronic obstructive pulmonary disease. Am J Respir Crit Care Med 2005; 171(10):1116–1124.
- Levine S, Kaiser L, Leferovich J, Tikunov B. Cellular adaptations in the diaphragm in chronic obstructive pulmonary disease. N Engl J Med 1997; 337(25):1799–1806.
- Levine S, Bashir MH, Clanton TL, Powers SK, Singhal S. COPD elicits remodeling of the diaphragm and vastus lateralis muscles in humans. J Appl Physiol 2013; 114(9):1235–1245.
- Marin-Corral J, Minguella J, Ramirez-Sarmiento AL, Hussain SN, Gea J, Barreiro E. Oxidised proteins and superoxide anion production in the diaphragm of severe COPD patients. Eur Respir J 2009; 33(6):1309–1319.
- Polkey MI, Kyroussis D, Hamnegard CH, Mills GH, Green M, Moxham J. Diaphragm strength in chronic obstructive pulmonary disease. Am J Respir Crit Care Med 1996; 154(5):1310–1317.
- Gayan-Ramirez G, Decramer M. Mechanisms of striated muscle dysfunction during acute exacerbations of COPD. J Appl Physiol 2013; 114(9):1291–1299.
- Gosselink R, Troosters T, Decramer M. Distribution of muscle weakness in patients with stable chronic obstructive pulmonary disease. J Cardiopulm Rehabil 2000; 20(6):353–360.
- Simpson K, Killian K, McCartney N, Stubbing DG, Jones NL. Randomised controlled trial of weightlifting exercise in patients with chronic airflow limitation. Thorax 1992; 47(2):70–75.
- Decramer M, Gosselink R, Troosters T, Verschueren M, Evers G. Muscle weakness is related to utilization of health care resources in COPD patients. Eur Respir J 1997; 10(2):417–423.
- Burtin C, Saey D, Saglam M, Langer D, Gosselink R, Janssens W, Decramer M, Maltais F, Troosters T. Effectiveness of exercise training in patients with COPD: the role of muscle fatigue. Eur Respir J 2012; 40(2):338–344.
- Bachasson D, Wuyam B, Pepin JL, Tamisier R, Levy P, Verges S. Quadriceps and respiratory muscle fatigue following high-intensity cycling in COPD patients. PLoS One 2013; 8(12):e83432.
- Mador MJ, Bozkanat E, Kufel TJ. Quadriceps fatigue after cycle exercise in patients with COPD compared with healthy control subjects. Chest 2003; 123(4):1104–1111.
- Gagnon P, Maltais F, Bouyer L, Ribeiro F, Coats V, Brouillard C, Noel M, Rousseau-Gagnon M, Saey D. Distal leg muscle function in patients with COPD. COPD 2013; 10(2):235–242.
- Marquis N, Debigare R, Bouyer L, Saey D, Laviolette L, Brouillard C, Maltais F. Physiology of walking in patients with moderate to severe chronic obstructive pulmonary disease. Med Sci Sports Exerc 2009; 41(8):1540–1548.
- Clark CJ, Cochrane LM, Mackay E, Paton B. Skeletal muscle strength and endurance in patients with mild COPD and the effects of weight training. Eur Respir J 2000; 15(1):92–97.
- Newell SZ, McKenzie DK, Gandevia SC. Inspiratory and skeletal muscle strength and endurance and diaphragmatic activation in patients with chronic airflow limitation. Thorax 1989; 44(11):903–912.
- Crul T, Spruit MA, Gayan-Ramirez G, Quarck R, Gosselink R, Troosters T, Pitta F, Decramer M. Markers of inflammation and disuse in vastus lateralis of chronic obstructive pulmonary disease patients. Eur J Clin Invest 2007; 37(11):897–904.
- Pitta F, Troosters T, Probst VS, Spruit MA, Decramer M, Gosselink R. Physical activity and hospitalization for exacerbation of COPD. Chest 2006; 129(3):536–544.
- Spruit MA, Gosselink R, Troosters T, Kasran A, Gayan-Ramirez G, Bogaerts P, Bouillon R, Decramer M. Muscle force during an acute exacerbation in hospitalised patients with COPD and its relationship with CXCL8 and IGF-I. Thorax 2003; 58(9):752–756.
- Similowski T, Yan S, Gauthier AP, Macklem PT, Bellemare F. Contractile properties of the human diaphragm during chronic hyperinflation. N Engl J Med 1991; 325(13):917–923.
- Ramirez-Sarmiento A, Orozco-Levi M, Barreiro E, Mendez R, Ferrer A, Broquetas J, Gea J. Expiratory muscle endurance in chronic obstructive pulmonary disease. Thorax 2002; 57(2):132–136.
- Ramirez-Sarmiento A, Orozco-Levi M, Guell R, Barreiro E, Hernandez N, Mota S, Sangenis M, Broquetas JM, Casan P, Gea J. Inspiratory muscle training in patients with chronic obstructive pulmonary disease: structural adaptation and physiologic outcomes. Am J Respir Crit Care Med 2002; 166(11):1491–1497.
- Barreiro E, Sieck G. Muscle dysfunction in COPD. J Appl Physiol 2013; 114(9):1220–1221.
- Mantilla CB, Sieck GC. Neuromotor control in chronic obstructive pulmonary disease. J Appl Physiol 2013; 114(9):1246–1252.
- Remels AH, Gosker HR, Langen RC, Schols AM. The mechanisms of cachexia underlying muscle dysfunction in COPD. J Appl Physiol 2013; 114(9):1253–1262.
- Barreiro E, Sznajder JI. Epigenetic regulation of muscle phenotype and adaptation: a potential role in COPD muscle dysfunction. J Appl Physiol 2013; 114(9):1263–1272.
- Barreiro E, Gea J. Epigenetics and muscle dysfunction in chronic obstructive pulmonary disease. Transl Res 2014; Apr 15. pii: S1931-5244(14)00130-3. doi: 10.1016/j.trsl.2014.04.006. [Epub ahead of print] Review. PMID: 24794953 [PubMed - as supplied by publisher]
- Hussain SN, Sandri M. Role of autophagy in COPD skeletal muscle dysfunction. J Appl Physiol 2013; 114(9):1273–1281.
- Puente-Maestu L, Lazaro A, Humanes B. Metabolic derangements in COPD muscle dysfunction. J Appl Physiol 2013; 114(9):1282–1290.
- Ribeiro F, Theriault ME, Debigare R, Maltais F. Should all patients with COPD be exercise trained? J Appl Physiol 2013; 114(9):1300–1308.
- Gea J, Martinez-Llorens J, Barreiro E. [Nutritional abnormalities in chronic obstructive pulmonary disease.]. Med Clin (Barc) 2014; 143(2):78–84.
- Crul T, Testelmans D, Spruit MA, Troosters T, Gosselink R, Geeraerts I, Decramer M, Gayan-Ramirez G. Gene expression profiling in vastus lateralis muscle during an acute exacerbation of COPD. Cell Physiol Biochem 2010; 25(4–5):491–500.
- Decramer M, de B, V, Dom R. Functional and histologic picture of steroid-induced myopathy in chronic obstructive pulmonary disease. Am J Respir Crit Care Med 1996; 153(6 Pt 1):1958–1964.
- Gosker HR, Zeegers MP, Wouters EF, Schols AM. Muscle fibre type shifting in the vastus lateralis of patients with COPD is associated with disease severity: a systematic review and meta-analysis. Thorax 2007; 62(11):944–949.
- Natanek SA, Riddoch-Contreras J, Marsh GS, Hopkinson NS, Man WD, Moxham J, Polkey MI, Kemp PR. Yin Yang 1 expression and localisation in quadriceps muscle in COPD. Arch Bronconeumol 2011; 47(6):296–302.
- Lexell J, Downham D. What is the effect of ageing on type 2 muscle fibres? J Neurol Sci 1992; 107(2):250–251.
- Kelsen SG, Ference M, Kapoor S. Effects of prolonged undernutrition on structure and function of the diaphragm. J Appl Physiol 1985; 58(4):1354–1359.
- Dekhuijzen PN, Decramer M. Steroid-induced myopathy and its significance to respiratory disease: a known disease rediscovered. Eur Respir J 1992; 5(8):997–1003.
- Jobin J, Maltais F, Doyon JF, LeBlanc P, Simard PM, Simard AA, Simard C. Chronic obstructive pulmonary disease: capillarity and fiber-type characteristics of skeletal muscle. J Cardiopulm Rehabil 1998; 18(6):432–437.
- Whittom F, Jobin J, Simard PM, LeBlanc P, Simard C, Bernard S, Belleau R, Maltais F. Histochemical and morphological characteristics of the vastus lateralis muscle in patients with chronic obstructive pulmonary disease. Med Sci Sports Exerc 1998; 30(10):1467–1474.
- Maltais F, Simard AA, Simard C, Jobin J, Desgagnes P, LeBlanc P. Oxidative capacity of the skeletal muscle and lactic acid kinetics during exercise in normal subjects and in patients with COPD. Am J Respir Crit Care Med 1996; 153(1):288–293.
- Saey D, Michaud A, Couillard A, Cote CH, Mador MJ, LeBlanc P, Jobin J, Maltais F. Contractile fatigue, muscle morphometry, and blood lactate in chronic obstructive pulmonary disease. Am J Respir Crit Care Med 2005; 171(10):1109–1115.
- Saey D, Lemire BB, Gagnon P, Bombardier E, Tupling AR, Debigare R, Cote CH, Maltais F. Quadriceps metabolism during constant workrate cycling exercise in chronic obstructive pulmonary disease. J Appl Physiol 2011; 110(1):116–124.
- Orozco-Levi M, Coronell C, Ramirez-Sarmiento A, Lloreta J, Martinez-Llorens J, Galdiz JB, Gea J. Injury of peripheral muscles in smokers with chronic obstructive pulmonary disease. Ultrastruct Pathol 2012; 36(4):228–238.
- Heijdra YF, Pinto-Plata V, Frants R, Rassulo J, Kenney L, Celli BR. Muscle strength and exercise kinetics in COPD patients with a normal fat-free mass index are comparable to control subjects. Chest 2003; 124(1):75–82.
- Naimi AI, Bourbeau J, Perrault H, Baril J, Wright-Paradis C, Rossi A, Taivassalo T, Sheel AW, Rabol R, Dela F, Boushel R. Altered mitochondrial regulation in quadriceps muscles of patients with COPD. Clin Physiol Funct Imag 2011; 31(2):124–131.
- Puente-Maestu L, Perez-Parra J, Godoy R, Moreno N, Tejedor A, Torres A, Lazaro A, Ferreira A, Agusti A. Abnormal transition pore kinetics and cytochrome C release in muscle mitochondria of patients with chronic obstructive pulmonary disease. Am J Respir Cell Mol Biol 2009; 40(6):746–750.
- Puente-Maestu L, Lazaro A, Tejedor A, Camano S, Fuentes M, Cuervo M, Navarro BO, Agusti A. Effects of exercise on mitochondrial DNA content in skeletal muscle of patients with COPD. Thorax 2011; 66(2):121–127.
- Barreiro E, Schols AM, Polkey MI, Galdiz JB, Gosker HR, Swallow EB, Coronell C, Gea J. Cytokine profile in quadriceps muscles of patients with severe COPD. Thorax 2008; 63(2):100–107.
- Barreiro E, Rabinovich R, Marin-Corral J, Barbera JA, Gea J, Roca J. Chronic endurance exercise induces quadriceps nitrosative stress in patients with severe COPD. Thorax 2009; 64(1):13–19.
- Barreiro E, Peinado VI, Galdiz JB, Ferrer E, Marin-Corral J, Sanchez F, Gea J, Barbera JA. Cigarette smoke-induced oxidative stress: A role in chronic obstructive pulmonary disease skeletal muscle dysfunction. Am J Respir Crit Care Med 2010; 182(4):477–488.
- Couillard A, Maltais F, Saey D, Debigare R, Michaud A, Koechlin C, LeBlanc P, Prefaut C. Exercise-induced quadriceps oxidative stress and peripheral muscle dysfunction in patients with chronic obstructive pulmonary disease. Am J Respir Crit Care Med 2003; 167(12):1664–1669.
- Fermoselle C, Sanchez F, Barreiro E. [Reduction of muscle mass mediated by myostatin in an experimental model of pulmonary emphysema]. Arch Bronconeumol 2011; 47(12):590–598.
- Koechlin C, Couillard A, Simar D, Cristol JP, Bellet H, Hayot M, Prefaut C. Does oxidative stress alter quadriceps endurance in chronic obstructive pulmonary disease? Am J Respir Crit Care Med 2004; 169(9):1022–1027.
- Koechlin C, Maltais F, Saey D, Michaud A, LeBlanc P, Hayot M, Prefaut C. Hypoxaemia enhances peripheral muscle oxidative stress in chronic obstructive pulmonary disease. Thorax 2005; 60(10):834–841.
- Rodriguez DA, Kalko S, Puig-Vilanova E, Perez-Olabarria M, Falciani F, Gea J, Cascante M, Barreiro E, Roca J. Muscle and blood redox status after exercise training in severe COPD patients. Free Radic Biol Med 2012; 52(1):88–94.
- Barreiro E, Ferrer D, Sanchez F, Minguella J, Marin-Corral J, Martinez-Llorens J, Lloreta J, Gea J. Inflammatory cells and apoptosis in respiratory and limb muscles of patients with COPD. J Appl Physiol 2011; 111(3):808–817.
- Takabatake N, Nakamura H, Abe S, Inoue S, Hino T, Saito H, Yuki H, Kato S, Tomoike H. The relationship between chronic hypoxemia and activation of the tumor necrosis factor-alpha system in patients with chronic obstructive pulmonary disease. Am J Respir Crit Care Med 2000; 161(4 Pt 1):1179–1184.
- Caron MA, Theriault ME, Pare ME, Maltais F, Debigare R. Hypoxia alters contractile protein homeostasis in L6 myotubes. FEBS Lett 2009; 583(9):1528—1534.
- Chandel NS, McClintock DS, Feliciano CE, Wood TM, Melendez JA, Rodriguez AM, Schumacker PT. Reactive oxygen species generated at mitochondrial complex III stabilize hypoxia-inducible factor-1alpha during hypoxia: a mechanism of O2 sensing. J Biol Chem 2000; 275(33):25130–25138.
- de TC, Costes F, Langen RC, Pison C, Gosker HR. Hypoxia and muscle maintenance regulation: implications for chronic respiratory disease. Curr Opin Clin Nutr Metab Care 2011; 14(6):548–553.
- Bailey JL, Wang X, England BK, Price SR, Ding X, Mitch WE. The acidosis of chronic renal failure activates muscle proteolysis in rats by augmenting transcription of genes encoding proteins of the ATP-dependent ubiquitin-proteasome pathway. J Clin Invest 1996; 97(6):1447–1453.
- Franch HA, Raissi S, Wang X, Zheng B, Bailey JL, Price SR. Acidosis impairs insulin receptor substrate-1-associated phosphoinositide 3-kinase signaling in muscle cells: consequences on proteolysis. Am J Physiol Renal Physiol 2004; 287(4):F700–F706.
- Barreiro E, del Puerto-Nevado L, Puig-Vilanova E, Perez-Rial S, Sanchez F, Martinez-Galan L, Rivera S, Gea J, Gonzalez-Mangado N, Peces-Barba G. Cigarette smoke-induced oxidative stress in skeletal muscles of mice. Respir Physiol Neurobiol 2012; 182(1):9–17.
- Donaldson A, Natanek SA, Lewis A, Man WD, Hopkinson NS, Polkey MI, Kemp PR. Increased skeletal muscle-specific microRNA in the blood of patients with COPD. Thorax 2013; 68(12):1140–1149.
- Lewis A, Riddoch-Contreras J, Natanek SA, Donaldson A, Man WD, Moxham J, Hopkinson NS, Polkey MI, Kemp PR. Downregulation of the serum response factor/miR-1 axis in the quadriceps of patients with COPD. Thorax 2012; 67(1):26–34.
- Puig-Vilanova E, Ausin P, Martinez-Llorens J, Gea J, Barreiro E. Do epigenetic events take place in the vastus lateralis of patients with mild chronic obstructive pulmonary disease? PLoS One 2014; 9(7):e102296.
- Guo Y, Gosker HR, Schols AM, Kapchinsky S, Bourbeau J, Sandri M, Jagoe RT, Debigare R, Maltais F, Taivassalo T, Hussain SN. Autophagy in locomotor muscles of patients with chronic obstructive pulmonary disease. Am J Respir Crit Care Med 2013; 188(11):1313–1320.
- Orozco-Levi M, Gea J, Lloreta JL, Felez M, Minguella J, Serrano S, Broquetas JM. Subcellular adaptation of the human diaphragm in chronic obstructive pulmonary disease. Eur Respir J 1999; 13(2):371–378.
- Doucet M, Debigare R, Joanisse DR, Cote C, LeBlanc P, Gregoire J, Deslauriers J, Vaillancourt R, Maltais F. Adaptation of the diaphragm and the vastus lateralis in mild-to-moderate COPD. Eur Respir J 2004; 24(6):971–979.
- Ribera F, N'Guessan B, Zoll J, Fortin D, Serrurier B, Mettauer B, Bigard X, Ventura-Clapier R, Lampert E. Mitochondrial electron transport chain function is enhanced in inspiratory muscles of patients with chronic obstructive pulmonary disease. Am J Respir Crit Care Med 2003; 167(6):873–879.
- Wijnhoven JH, Janssen AJ, van Kuppevelt TH, Rodenburg RJ, Dekhuijzen PN. Metabolic capacity of the diaphragm in patients with COPD. Respir Med 2006; 100(6):1064–1071.
- Jimenez-Fuentes MA, Gea J, Aguar MC, Minguella J, Lloreta J, Felez M, Broquetas J. [Capillary density and respiratory function in the external intercostal muscle]. Arch Bronconeumol 1999; 35(10):471–476.
- Rivero JL, Ruz A, Marti-Korff S, Estepa JC, Aguilera-Tejero E, Werkman J, Sobotta M, Lindner A. Effects of intensity and duration of exercise on muscular responses to training of thoroughbred racehorses. J Appl Physiol 2007; 102(5):1871–1882.
- Schakman O, Gilson H, Kalista S, Thissen JP. Mechanisms of muscle atrophy induced by glucocorticoids. Horm Res 2009; 72 Suppl 1:36–41.
- Ottenheijm CA, Heunks LM, Li YP, Jin B, Minnaard R, van Hees HW, Dekhuijzen PN. Activation of the ubiquitin-proteasome pathway in the diaphragm in chronic obstructive pulmonary disease. Am J Respir Crit Care Med 2006; 174(9):997–1002.
- Schols AM, Soeters PB, Mostert R, Saris WH, Wouters EF. Energy balance in chronic obstructive pulmonary disease. Am Rev Respir Dis 1991; 143(6):1248–1252.
- Spruit MA, Singh SJ, Garvey C, ZuWallack R, Nici L, Rochester C, Hill K, Holland AE, Lareau SC, Man WD, Pitta F, Sewell L, et al. An official American Thoracic Society/European Respiratory Society statement: key concepts and advances in pulmonary rehabilitation. Am J Respir Crit Care Med 2013; 188(8):e13–e64.
- Spruit MA, Franssen FM, Rutten EP, Wagers SS, Wouters EF. Age-graded reductions in quadriceps muscle strength and peak aerobic capacity in COPD. Rev Bras Fisioter 2012; 16(2):148-56.
- Maltais F, LeBlanc P, Simard C, Jobin J, Berube C, Bruneau J, Carrier L, Belleau R. Skeletal muscle adaptation to endurance training in patients with chronic obstructive pulmonary disease. Am J Respir Crit Care Med 1996; 154(2 Pt 1):442–447.
- Franssen FM, Broekhuizen R, Janssen PP, Wouters EF, Schols AM. Effects of whole-body exercise training on body composition and functional capacity in normal-weight patients with COPD. Chest 2004; 125(6):2021–2028.
- Spruit MA, Gosselink R, Troosters T, De PK, Decramer M. Resistance versus endurance training in patients with COPD and peripheral muscle weakness. Eur Respir J 2002; 19(6):1072–1078.
- Porszasz J, Emtner M, Goto S, Somfay A, Whipp BJ, Casaburi R. Exercise training decreases ventilatory requirements and exercise-induced hyperinflation at submaximal intensities in patients with COPD. Chest 2005; 128(4):2025–2034.
- Horowitz MB, Littenberg B, Mahler DA. Dyspnea ratings for prescribing exercise intensity in patients with COPD. Chest 1996; 109(5):1169–1175.
- Bernard S, LeBlanc P, Whittom F, Carrier G, Jobin J, Belleau R, Maltais F. Peripheral muscle weakness in patients with chronic obstructive pulmonary disease. Am J Respir Crit Care Med 1998; 158(2):629–634.
- Maltais F, LeBlanc P, Whittom F, Simard C, Marquis K, Belanger M, Breton MJ, Jobin J. Oxidative enzyme activities of the vastus lateralis muscle and the functional status in patients with COPD. Thorax 2000; 55(10):848–853.
- Vogiatzis I, Terzis G, Nanas S, Stratakos G, Simoes DC, Georgiadou O, Zakynthinos S, Roussos C. Skeletal muscle adaptations to interval training in patients with advanced COPD. Chest 2005; 128(6):3838—3845.
- Vogiatzis I, Stratakos G, Simoes DC, Terzis G, Georgiadou O, Roussos C, Zakynthinos S. Effects of rehabilitative exercise on peripheral muscle TNFalpha, IL-6, IGF-I and MyoD expression in patients with COPD. Thorax 2007; 62(11):950–956.
- Vogiatzis I, Simoes DC, Stratakos G, Kourepini E, Terzis G, Manta P, Athanasopoulos D, Roussos C, Wagner PD, Zakynthinos S. Effect of pulmonary rehabilitation on muscle remodelling in cachectic patients with COPD. Eur Respir J 2010; 36(2):301–310.
- Vogiatzis I, Terzis G, Stratakos G, Cherouveim E, Athanasopoulos D, Spetsioti S, Nasis I, Manta P, Roussos C, Zakynthinos S. Effect of pulmonary rehabilitation on peripheral muscle fiber remodeling in patients with COPD in GOLD stages II to IV. Chest 2011; 140(3):744–752.
- Sala E, Roca J, Marrades RM, Alonso J, Gonzalez De Suso JM, Moreno A, Barbera JA, Nadal J, de JL, Rodriguez-Roisin R, Wagner PD. Effects of endurance training on skeletal muscle bioenergetics in chronic obstructive pulmonary disease. Am J Respir Crit Care Med 1999; 159(6):1726–1734.
- Ortega F, Toral J, Cejudo P, Villagomez R, Sanchez H, Castillo J, Montemayor T. Comparison of effects of strength and endurance training in patients with chronic obstructive pulmonary disease. Am J Respir Crit Care Med 2002; 166(5):669–674.
- Maltais F, LeBlanc P, Jobin J, Berube C, Bruneau J, Carrier L, Breton MJ, Falardeau G, Belleau R. Intensity of training and physiologic adaptation in patients with chronic obstructive pulmonary disease. Am J Respir Crit Care Med 1997; 155(2):555–561.
- Mador MJ, Bozkanat E, Aggarwal A, Shaffer M, Kufel TJ. Endurance and strength training in patients with COPD. Chest 2004; 125(6):2036–2045.
- Troosters T, Probst VS, Crul T, Pitta F, Gayan-Ramirez G, Decramer M, Gosselink R. Resistance training prevents deterioration in quadriceps muscle function during acute exacerbations of chronic obstructive pulmonary disease. Am J Respir Crit Care Med 2010; 181(10):1072–1077.
- Janaudis-Ferreira T, Hill K, Goldstein R, Wadell K, Brooks D. Arm exercise training in patients with chronic obstructive pulmonary disease: a systematic review. J Cardiopulm Rehabil Prev 2009; 29(5):277–283.
- Neder JA, Sword D, Ward SA, Mackay E, Cochrane LM, Clark CJ. Home based neuromuscular electrical stimulation as a new rehabilitative strategy for severely disabled patients with chronic obstructive pulmonary disease (COPD). Thorax 2002; 57(4):333–337.
- Bourjeily-Habr G, Rochester CL, Palermo F, Snyder P, Mohsenin V. Randomised controlled trial of transcutaneous electrical muscle stimulation of the lower extremities in patients with chronic obstructive pulmonary disease. Thorax 2002; 57(12):1045–1049.
- Sillen MJ, Speksnijder CM, Eterman RM, Janssen PP, Wagers SS, Wouters EF, Uszko-Lencer NH, Spruit MA. Effects of neuromuscular electrical stimulation of muscles of ambulation in patients with chronic heart failure or COPD: a systematic review of the English-language literature. Chest 2009; 136(1):44–61.
- Abdellaoui A, Prefaut C, Gouzi F, Couillard A, Coisy-Quivy M, Hugon G, Molinari N, Lafontaine T, Jonquet O, Laoudj-Chenivesse D, Hayot M. Skeletal muscle effects of electrostimulation after COPD exacerbation: a pilot study. Eur Respir J 2011; 38(4):781–788.
- Vivodtzev I, Debigare R, Gagnon P, Mainguy V, Saey D, Dube A, Pare ME, Belanger M, Maltais F. Functional and muscular effects of neuromuscular electrical stimulation in patients with severe COPD: a randomized clinical trial. Chest 2012; 141(3):716–725.
- Sillen MJ, Franssen FM, Delbressine JM, Vaes AW, Wouters EF, Spruit MA. Efficacy of lower-limb muscle training modalities in severely dyspnoeic individuals with COPD and quadriceps muscle weakness: results from the DICES trial. Thorax 2014; 69(6):525–531.
- Madariaga VB, Manterola AG, Miro EL, Iturri JB. [Magnetic stimulation of the quadriceps: analysis of 2 stimulators used for diagnostic and therapeutic applications]. Arch Bronconeumol 2007; 43(7):411–417.
- Man WD, Moxham J, Polkey MI. Magnetic stimulation for the measurement of respiratory and skeletal muscle function. Eur Respir J 2004; 24(5):846–860.
- Bustamante V, Casanova J, Lopez de SE, Mas S, Sellares J, Gea J, Galdiz JB, Barreiro E. Redox balance following magnetic stimulation training in the quadriceps of patients with severe COPD. Free Radic Res 2008; 42(11–12):939–948.
- Bustamante V, Lopez de Santa ME, Gorostiza A, Jimenez U, Galdiz JB. Muscle training with repetitive magnetic stimulation of the quadriceps in severe COPD patients. Respir Med 2010; 104(2):237–245.
- Creutzberg EC, Wouters EF, Mostert R, Weling-Scheepers CA, Schols AM. Efficacy of nutritional supplementation therapy in depleted patients with chronic obstructive pulmonary disease. Nutrition 2003; 19(2):120–127.
- Weekes CE, Emery PW, Elia M. Dietary counselling and food fortification in stable COPD: a randomised trial. Thorax 2009; 64(4):326–331.
- Casaburi R, Bhasin S, Cosentino L, Porszasz J, Somfay A, Lewis MI, Fournier M, Storer TW. Effects of testosterone and resistance training in men with chronic obstructive pulmonary disease. Am J Respir Crit Care Med 2004; 170(8):870–878.
- Gullett NP, Hebbar G, Ziegler TR. Update on clinical trials of growth factors and anabolic steroids in cachexia and wasting. Am J Clin Nutr 2010; 91(4):1143S–1147S.
- Weisberg J, Wanger J, Olson J, Streit B, Fogarty C, Martin T, Casaburi R. Megestrol acetate stimulates weight gain and ventilation in underweight COPD patients. Chest 2002;121(4):1070–1078.
- Borghi-Silva A, Baldissera V, Sampaio LM, Pires-DiLorenzo VA, Jamami M, Demonte A, Marchini JS, Costa D. L-carnitine as an ergogenic aid for patients with chronic obstructive pulmonary disease submitted to whole-body and respiratory muscle training programs. Braz J Med Biol Res 2006; 39(4):465–474.
- Deacon SJ, Vincent EE, Greenhaff PL, Fox J, Steiner MC, Singh SJ, Morgan MD. Randomized controlled trial of dietary creatine as an adjunct therapy to physical training in chronic obstructive pulmonary disease. Am J Respir Crit Care Med 2008; 178(3):233-239.
- Barreiro E, Galdiz JB, Marinan M, Alvarez FJ, Hussain SN, Gea J. Respiratory loading intensity and diaphragm oxidative stress: N-acetyl-cysteine effects. J Appl Physiol 2006; 100(2):555–563.
- Laviolette L, Lands LC, Dauletbaev N, Saey D, Milot J, Provencher S, LeBlanc P, Maltais F. Combined effect of dietary supplementation with pressurized whey and exercise training in chronic obstructive pulmonary disease: a randomized, controlled, double-blind pilot study. J Med Food 2010; 13(3):589–598.
- Lehouck A, Mathieu C, Carremans C, Baeke F, Verhaegen J, Van EJ, Decallonne B, Bouillon R, Decramer M, Janssens W. High doses of vitamin D to reduce exacerbations in chronic obstructive pulmonary disease: a randomized trial. Ann Intern Med 2012; 156(2):105–114.
- Hamilton AL, Killian KJ, Summers E, Jones NL. Muscle strength, symptom intensity, and exercise capacity in patients with cardiorespiratory disorders. Am J Respir Crit Care Med 1995; 152(6 Pt 1):2021–2031.
- Wanke T, Formanek D, Lahrmann H, Brath H, Wild M, Wagner C, Zwick H. Effects of combined inspiratory muscle and cycle ergometer training on exercise performance in patients with COPD. Eur Respir J 1994; 7(12):2205–2211.
- Gosselink R, De VJ, van den Heuvel SP, Segers J, Decramer M, Kwakkel G. Impact of inspiratory muscle training in patients with COPD: what is the evidence? Eur Respir J 2011; 37(2):416–425.
- Larson JL, Kim MJ, Sharp JT, Larson DA. Inspiratory muscle training with a pressure threshold breathing device in patients with chronic obstructive pulmonary disease. Am Rev Respir Dis 1988; 138(3):689–696.
- Geddes EL, O'Brien K, Reid WD, Brooks D, Crowe J. Inspiratory muscle training in adults with chronic obstructive pulmonary disease: an update of a systematic review. Respir Med 2008; 102(12):1715–1729.
- Mota S, Guell R, Barreiro E, Solanes I, Ramirez-Sarmiento A, Orozco-Levi M, Casan P, Gea J, Sanchis J. Clinical outcomes of expiratory muscle training in severe COPD patients. Respir Med 2007; 101(3):516–524.
- Hill K, Jenkins SC, Philippe DL, Cecins N, Shepherd KL, Green DJ, Hillman DR, Eastwood PR. High-intensity inspiratory muscle training in COPD. Eur Respir J 2006; 27(6):1119–1128.
- Lotters F, van TB, Kwakkel G, Gosselink R. Effects of controlled inspiratory muscle training in patients with COPD: a meta-analysis. Eur Respir J 2002; 20(3):570–576.
- Criner GJ, Cordova F, Sternberg AL, Martinez FJ. The National Emphysema Treatment Trial (NETT) Part II: Lessons learned about lung volume reduction surgery. Am J Respir Crit Care Med 2011; 184(8):881–893.
- Teschler H, Stamatis G, el-Raouf Farhat AA, Meyer FJ, Costabel U, Konietzko N. Effect of surgical lung volume reduction on respiratory muscle function in pulmonary emphysema. Eur Respir J 1996; 9(9):1779–1784.
- van Hees HW, Dekhuijzen PN, Heunks LM. Levosimendan enhances force generation of diaphragm muscle from patients with chronic obstructive pulmonary disease. Am J Respir Crit Care Med 2009; 179(1):41–47.
- Doorduin J, Sinderby CA, Beck J, Stegeman DF, van Hees HW, van der Hoeven JG, Heunks LM. The calcium sensitizer levosimendan improves human diaphragm function. Am J Respir Crit Care Med 2012; 185(1):90–95.
- Travaline JM, Sudarshan S, Criner GJ. Recovery of PdiTwitch following the induction of diaphragm fatigue in normal subjects. Am J Respir Crit Care Med 1997; 156(5):1562–1566.