Abstract
Heart failure, a prevalent and disabling co-morbidity of COPD, may impair cardiac output and muscle blood flow thereby contributing to exercise intolerance. To investigate the role of impaired central and peripheral hemodynamics in limiting exercise tolerance in COPD-heart failure overlap, cycle ergometer exercise tests at 20% and 80% peak work rate were performed by overlap (FEV1 = 56.9 ± 15.9% predicted, ejection fraction = 32.5 ± 6.9%; N = 16), FEV1-matched COPD (N = 16), ejection fraction-matched heart failure patients (N = 15) and controls (N = 12). Differences (Δ) in cardiac output (impedance cardiography) and vastus lateralis blood flow (indocyanine green) and deoxygenation (near-infrared spectroscopy) between work rates were expressed relative to concurrent changes in muscle metabolic demands (ΔO2 uptake). Overlap patients had approximately 30% lower endurance exercise tolerance than COPD and heart failure (p < 0.05). ΔBlood flow was closely proportional to Δcardiac output in all groups (r = 0.89–0.98; p < 0.01). Overlap showed the largest impairments in Δcardiac output/ΔO2 uptake and Δblood flow/ΔO2 uptake (p < 0.05). Systemic arterial oxygenation, however, was preserved in overlap compared to COPD. Blunted limb perfusion was related to greater muscle deoxygenation and lactate concentration in overlap (r = 0.78 and r = 0.73, respectively; p < 0.05). ΔBlood flow/ΔO2 uptake was related to time to exercise intolerance only in overlap and heart failure (p < 0.01). In conclusion, COPD and heart failure add to decrease exercising cardiac output and skeletal muscle perfusion to a greater extent than that expected by heart failure alone. Treatment strategies that increase muscle O2 delivery and/or decrease O2 demand may be particularly helpful to improve exercise tolerance in COPD patients presenting heart failure as co-morbidity.
Introduction
Exercise intolerance is a cardinal feature of chronic obstructive pulmonary disease (COPD) (Citation1). The underlying mechanisms, however, vary in patients showing similar resting ventilatory impairments (Citation2). There is also growing recognition that the phenotypic exteriorization of COPD and its pathophysiological consequences are influenced by co-morbidities (Citation1). Cardiocirculatory diseases, in particular, are highly prevalent in COPD and impact strongly on patients’ disability and quality of life (Citation3–5). Understanding how COPD and coexistent cardiocirculatory disease may interact to further compromise exercise tolerance remains an unmet clinical need in the care of these patients (Citation6,7).
In this context, heart failure with reduced left ventricular ejection fraction overlaps with COPD in nearly 1 in 3 elderly patients (Citation8). Blood flow and, consequently, oxygen (O2) delivery to working skeletal muscles may be impaired during exercise in both COPD (Citation9–11) and heart failure (Citation12,13). In COPD, peripheral muscle blood flow might be reduced due to the central hemodynamic consequences of acute-on-chronic lung hyperinflation (Citation14–16) and/or cardiac output redistribution towards the overloaded respiratory muscles (Citation17,18). In heart failure, low cardiac output, global sympathetically mediated vasoconstriction and microvascular abnormalities characteristically compromise appendicular muscle blood flow (as reviewed in ref. (Citation13)). It is thus conceivable that when COPD and heart failure overlap the aforementioned negative cardiopulmonary interactions and resulting deficits in muscle perfusion may be potentiated. This could lead to further decrements in muscle oxygenation and exercise tolerance. Despite these considerations, no previous study has investigated these important outcomes in patients with both COPD and heart failure (thereafter named as “overlap” group).
The current prospective study evaluated the role of central (cardiac output) and peripheral (leg muscle blood flow and deoxygenation) impairments in the O2 transport pathway in limiting exercise tolerance in patients with overlapping COPD-heart failure. To investigate potential additive effects of COPD and heart failure on those outcomes, overlap patients were matched carefully to COPD patients showing similar resting ventilatory impairment (i.e., post-bronchodilator FEV1) and heart failure patients showing similar left ventricular ejection fraction. We hypothesized that exercising limb muscle blood flow would be impaired at greater extent in overlap leading to elevated fractional O2 extraction (i.e., ↑de-oxyhemoglobin concentration by near-infrared spectroscopy) (Citation9,Citation19), increased reliance on nonoxidative metabolism (i.e., as reflected by ↑blood lactate concentration) and poorer exercise tolerance compared to isolated diseases. Confirmation of the study hypotheses would provide a strong rationale for novel treatment strategies that increase muscle O2 delivery and/or decrease O2 demand in COPD-heart failure overlap.
Methods
Subjects
Sixteen sedentary males with moderate-to-severe, smoking-related COPD and echocardiographic evidences of heart failure with reduced left ventricular ejection fraction (< Citation40%) comprised the COPD-heart failure group. Patients were seen prospectively by the same respirologists and cardiologists. Echocardiograms were performed before study entry (unless performed in the previous 6 months) and read by the same trained echocardiographists. Disease treatment was decided by consensus and optimized carefully before study entry (). Sixteen age- and gender-matched COPD patients with no clinical or echocardiographic evidence of heart failure and 15 patients with established heart failure but no airflow obstruction (FEV1/forced vital capacity (FVC) ratio >0.70) were also enrolled. Patients with COPD or heart failure were selected to match the overlap group by post-bronchodilator FEV1 and left ventricular ejection fraction, respectively. All participants were required to report chronic dyspnea (modified (Citation1-5) Medical Research Council (MRC) scale score 2-4 and intolerance to physical exertion according to the New York Heart Association (NYHA) scheme (class 2 or 3).
Table 1. Subjects’ main characteristics and pharmacological treatment.
Main exclusion criteria were: long-term O2 therapy, recent (within a year) rehabilitation, osteomuscular limitation to cycling, type I or non-controlled type II diabetes mellitus and peripheral arterial disease associated with claudication. Heart failure with preserved ejection fraction (diastolic heart failure) or heart failure due to valvar disease were also exclusion factors. Twelve sedentary males free of major cardio-respiratory and metabolic diseases (controls) were recruited by advertisement. All subjects gave written informed consent and the study was approved by the Medical Ethics committee of the Sao Paulo Hospital, Sao Paulo, Brazil and Kingston General Hospital, Kingston, ON, Canada.
Measurements
Lung function
Spirometry, lung diffusing capacity, and static lung volumes were evaluated (1085 ELITE D™, Medical Graphics) and test results were compared with predicted values from Quanjer et al. (Citation20). Resting blood gases were obtained from samples from the radial artery.
Cardiopulmonary exercise testing
Patients performed a two-stage cycle ergometer test starting at 20% (for 4 min) and, after a 4-min resting period, at 80% (to the limit of tolerance; Tlim, s) of the maximal power achieved on an incremental test performed on a previous day (10 W/min ramp protocol for patients and 15 W/min for controls). Recovery after the 20% bout lasted 4 min in order to allow ventilatory and pulmonary gas exchange responses to reach stability before the 80% trial. O2 uptake (L/min), carbon dioxide (CO2) output (L/min), respiratory exchange ratio, minute ventilation (L/min) and end-tidal partial pressure for CO2 (mmHg) were obtained breath-by-breath and averaged into 20 s bins (CardiO2 System, Medical Graphics). Arterial oxygen saturation was measured non-invasively by pulse oximetry (SpO2,%; Nonin 7500, Nonin). Breathlessness and leg effort were rated every minute according to the 10-point Borg category-ratio scale modified by Burdon et al. (Citation21). Ventilation was expressed relative to estimated maximal voluntary ventilation (MVV (L/min) = FEV1 × 35). Heart rate (HR, bpm) was determined automatically using the RR interval from a 12-lead electrocardiogram. Blood gases and lactate concentration were measured from arterialized (art) earlobe capillary samples near the end of each exercise bout.
Cardiac output and muscle blood flow and deoxygenation
Cardiac output (L/min) was assessed continuously by a calibrated signal-morphology impedance cardiography system (Physioflow PF-5™, Manatec). Principles of operation and algorithms have been described in detail elsewhere (Citation22). Near-infrared spectroscopy (NIRS; NIRO 200™ Hamamatsu Photonics KK) was used to evaluate skeletal muscle blood flow and deoxygenation. The optode was placed laterally over the mid-third of the right vastus lateralis. As described in detail by our group (Citation9) and others (Citation19), deoxy-hemoglobin (HHb,%) was selected as a proxy for muscle fractional O2 extraction and expressed relative to maximum values elicited by cuff-induced ischemia (Citation23). The NIRS system measured indocyanine green (ICG) concentration following a bolus injection of 5 mg of ICG into a forearm vein. ICG injections were performed during the third minute of each exercise stage. As described previously (Citation23,24), a muscle blood flow index was calculated as the maximal change in ICG divided by its rise time. Differences (Δ) in cardiac output and vastus lateralis blood flow and deoxygenation between 20 and 80% peak work rate were expressed relative to changes in metabolic demands, i.e., ΔO2 uptake.
Statistical analysis
Results are reported as means ± SD (including in graphs), unless stated otherwise. Statistical significance was set at p < 0.05. One-way analysis of variance (ANOVA) with Bonferroni's post-hoc test (for symmetrically distributed data) or the Kruskal–Wallis H-test (for asymmetrically distributed data) was performed to evaluate between-group differences. Pearson's correlation coefficient was used to test the strength of association between variables.
Results
Groups were well matched for anthropometric and demographic variables (). Smoking history did not differ between COPD and overlap. As expected, ischemic heart disease was the commonest cause of heart failure in both groups (10/15 in heart failure and 12/16 in overlap) followed by non-ischemic dilated cardiomyopathy and hypertensive cardiomyopathy. There were no differences in reported chronic dyspnoea, NYHA functional class and the main co-morbidities among patient groups ().
Resting functional variables
As intended by the study design, FEV1 in COPD and ejection fraction in heart failure were matched to those found in overlap (). Among patient groups, COPD had the highest static lung volumes and PaCO2 together with the lowest lung diffusing capacity, whereas overlap showed intermediate values. Higher PaO2 values were found in heart failure compared to COPD and overlap ().
Table 2. Resting lung function and responses to incremental exercise.
Peak exercise variables
Despite the lack of statistically significant differences in peak work rate among patient groups, peak O2 uptake was lower in overlap and heart failure, which is consistent with a lower ΔO2 uptake/Δwork rate relationship in the latter groups (). As expected from β-blocker therapy (), significantly lower peak heart rate was found in heart failure and overlap. On the other hand, higher dyspnea scores and ventilation/MVV were found in COPD and overlap. Peak leg effort scores were greater in heart failure and overlap compared with COPD ().
Physiological and sensorial responses at 80% peak work rate
Overlap patients had the lowest exercise tolerance among patient groups (∼30% lower compared to COPD and heart failure; ). As expected from similar peak work rates among patient groups (), there were no significant differences in absolute work rates at 20% and 80% (values ranging from 10-32 W and 38-68 W, respectively). Controls, however, exercised at greater work rates and O2 uptake levels compared to all patient groups. Despite similar O2 uptake, respiratory exchange ratio and CO2 output were greater in heart failure and overlap patients. Greater systemic oxygen desaturation and decrements in arterialized PO2 were found in COPD patients compared to the other groups (). COPD had the highest dyspnea scores while heart failure and overlap had the highest leg effort scores. Consequently, leg effort–dyspnea differences at exercise cessation were larger in overlap and heart failure than COPD ().
Table 3. Physiological and sensorial responses to constant work rate exercise test at 80% peak.
Central and peripheral hemodynamics
Overlap had lower cardiac output and muscle blood flow at 80% peak work rate compared to other groups. Heart failure had lower values than COPD and controls with the highest values being found in controls (p < 0.05) ( and ). Overlap also showed the smallest variation (Δ) in cardiac output and muscle blood flow as a function of ΔO2 uptake from 20%–80% peak work rate (p < 0.05). Heart failure showed intermediate values of Δcardiac output/ΔO2 uptake and Δmuscle blood flow/ΔO2 uptake among patient groups whereas no differences were found between COPD and controls (p > 0.05) ( and ). ΔMuscle blood flow was closely proportional to Δcardiac output in all groups ( and ).
Figure 1. Central (panels a and b) and peripheral (panels c and d) hemodynamic responses as a function of exercise intensity and their relationship (panels e and f) in healthy controls and patients with chronic obstructive pulmonary disease (COPD), chronic heart failure (CHF) and COPD-CHF (overlap). “Δ“ is the difference between 20% and 80% peak work rate.
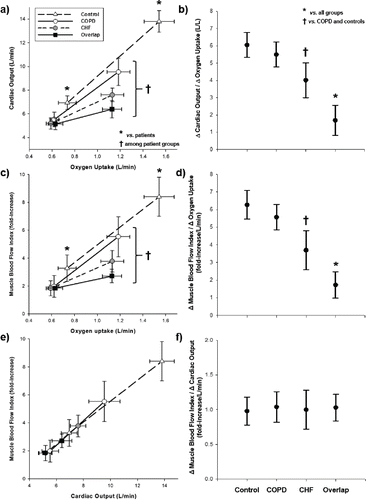
Blood lactate and muscle deoxygenation
Overlap had higher lactate and deoxygenation at 80% peak work rate compared to other groups. Heart failure had higher values than COPD and controls (p < 0.05). There were, however, no significant differences between COPD and controls ( and ). Overlap also showed the greatest increase (Δ) in lactate and muscle deoxygenation as a function of ΔO2 uptake from 20%–80% peak work rate. Heart failure had intermediate values of Δlactate/ΔO2 uptake and Δmuscle deoxygenation/ΔO2 uptake among patient groups, while no differences were found between COPD and controls (p > 0.05) ( and ). ΔLactate was proportionally related to Δmuscle deoxygenation in all groups ( and ). Of note, Δmuscle blood flow/ΔO2 uptake was inversely related to Δlactate and Δmuscle deoxygenation only in heart failure and overlap (r = −0.64 and r = −0.73, respectively; p < 0.01).
Muscle blood flow and exercise impairment
As shown in , Δmuscle blood flow/ΔO2 uptake was significantly related to time to exercise intolerance (tlim) in overlap and heart failure but not in COPD and controls.
Figure 3. Relationship between time to exercise intolerance (Tlim) and changes in muscle blood flow and oxygen uptake () in healthy controls and patients with chronic obstructive pulmonary disease (COPD), chronic heart failure (CHF) and COPD-CHF (overlap). “Δ“ is the difference between 20% and 80% peak work rate.
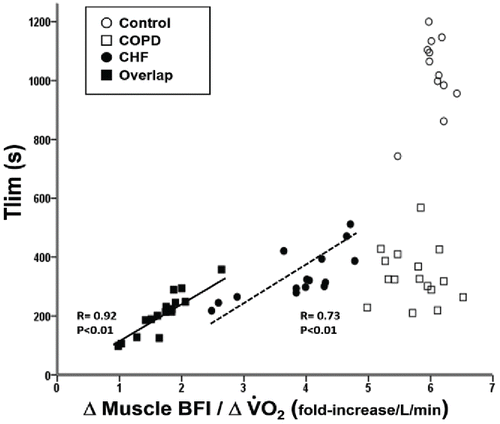
Discussion
This is the first study to assess the role of impaired central and peripheral hemodynamics on exercise intolerance in patients with coexistent COPD-heart failure (“overlap”). Consistent with our hypotheses, overlap patients had lower endurance exercise tolerance compared to FEV1-matched COPD and left ventricular ejection fraction-matched heart failure patients. Leg muscle blood flow, but not arterial oxygenation, was impaired to a greater extent in overlap being a powerful predictor of exercise intolerance in those patients. Reduced convective O2 delivery in overlap was accompanied by relatively greater fractional O2 extraction (i.e., increased muscle deoxygenation by NIRS)(Citation9,Citation19) and blood lactate concentration. Moreover, there was a shift on the locus of symptom limitation from dyspnoea to leg discomfort when COPD was accompanied by heart failure. In this context, therapies aimed at increasing muscle O2 delivery and/or decreasing O2 needs are expected to enhance exercise tolerance in this fast-growing patient population.
In the present study, overlap patients were matched by post-bronchodilator FEV1 to those with COPD. Although constituting the criterion used to classify severity in isolated COPD, FEV1 may not reflect faithfully respiratory impairments in overlap due to the compound effects of obstruction and restriction (Citation25). Patients were thus also matched by chronic dyspnoea and functional limitation () and their pharmacological treatments were standardized by the same physicians (). Inclusion of a heart failure group was instrumental to examine whether abnormalities in cardiac output and exercising muscle blood flow in overlap patients would be greater than those expected by heart failure alone. Moreover, an age-matched healthy group allowed us to control for potential abnormalities in central and peripheral hemodynamics brought on by senescence and/or detraining secondary to a sedentary lifestyle.
A key finding of the present study was the greater muscle blood flow impairment during exercise in overlap compared to not only COPD but also heart failure patients (c and ). Importantly, heart failure per se could not explain these findings given the similar ejection fraction and cardiac pharmacological treatment in overlap (). Putative mediators of this phenomenon include the effects of negative cardiopulmonary interactions on cardiac output (Citation2). In overlap patients, it is conceivable that relatively higher mean intra-thoracic pressures and larger pleural pressure swings induced by COPD further decrease right ventricular preload and left ventricular filling pressures (Citation2,Citation14,Citation16,Citation26). Larger increases in pulmonary arterial pressures may not only increase right ventricular afterload but also leftward-shift the interventricular septum, thereby lowering left ventricular volume (Citation15,Citation17,Citation26).
Due to the lack of inspiratory capacity maneuvers (to track operating lung volumes) and oesophageal pressure measurements we were unable to properly test the hypothesis that impaired cardiac performance was related to increased intra-thoracic pressure swings. Another possibility involves redistribution of cardiac output from the exercising limb muscles to the overloaded respiratory muscles (Citation17,18,Citation27). As shown in e and , however, the slope of the muscle blood flow-cardiac output relationship was remarkably similar across patient and control groups. Blunted chronotropic response secondary to β-blocker therapy may have also contributed to decrease exercise cardiac output in heart failure and overlap patients. Moreover, β-blockers (at least acutely) are known to change the glucose metabolism with a consequent increase in lactate levels (Citation28).
It should be noted, however, that lower cardiac output (a) and greater lactate levels (a) were found in overlap compared to heart failure despite similar β-blocker doses. These findings are consistent to the notion that COPD further deteriorated the hemodynamic responses to exertion in patients with heart failure. Collectively, our results suggest that, under the current circumstances, blunted muscle blood flow in overlap was likely related to deficits in cardiac output adjustments to exertion rather than blood flow redistribution. Direct hemodynamic measurements are warranted to confirm these non-invasive findings. Due to the lack of inspiratory capacity maneuvers (to track operating lung volumes) and oesophageal pressure measurements we could not provide mechanistic evidence that impaired cardiac performance was related to increased intra-thoracic pressure swings.
As illustrated in , relatively greater muscle fractional O2 extraction (i.e., elevated muscle deoxygenation) and lactate concentration are consistent with blunted blood flow responses ( and ) during exercise in overlap. Impaired muscle O2 delivery leads to greater reliance on O2-independent metabolism and accumulation of by-products (e.g., inorganic phosphate and H+) that contribute to muscle fatigue (Citation29) and enhanced ventilatory drive (Citation30). In fact, the locus of symptom limitation shifted from the respiratory system to the working peripheral muscles in overlap compared to COPD (). Moreover, the overlap group had higher ventilation-CO2 output ratios despite less hypoxemia (and, conceivably, less hypoxic drive) than COPD (). Altogether, these data support the notion that impaired peripheral muscle O2 delivery assumes a more prominent role in limiting exercise tolerance when heart failure adds to COPD (Citation6,Citation31–34).
A rather interesting finding was the relative preservation of systemic arterial oxygenation in overlap compared to COPD () in the face of greater exercising muscle fractional O2 extraction ( and ) and, conceivably, lower venous O2 content. Mechanisms related to this finding may include a better preserved diffusing capacity () and/or greater ventilatory response to exercise (). In fact, a hyperventilatory response to early metabolic acidosis has been shown to be protective against exercise-induced O2 desaturation in COPD (Citation35). Interpretation of a high ventilatory equivalent for CO2 being translated into alveolar hyperventilation (and not only “wasted” in the physiological dead space) is strengthened by the finding of lower arterialized PCO2 in overlap and heart failure compared to COPD (). Although the exact mechanism(s) operating in overlap await elucidation, this behavior likely minimized further decrements in muscle O2 delivery in these patients.
Clinical implications
There is growing recognition that patients with chronic cardiopulmonary diseases have similar mechanisms of exercise intolerance vis-à-vis impaired O2 delivery (Citation9,Citation32,Citation33,Citation37). Our data suggest that overlap patients are particularly prone to respond to interventions that increase skeletal muscle O2 delivery and/or reduce O2 demand. Potential therapeutic options include: (a) improving cardiac output by addressing negative cardiopulmonary interactions (e.g., via non-invasive positive pressure ventilation and/or heliox) (Citation10,Citation31), (b) adopting physical training strategies suitable for patients with severe central cardiorespiratory constraints (e.g., small muscle mass training) (Citation36,37), and (c) enhancing directly O2 delivery-to-utilization matching via increased muscle blood flow and/or lowered O2 cost of muscle contraction (e.g., sildenafil, nitrate supplementation) (Citation38,39).
Experimental considerations and study limitations
A methodological aspect of the present study that merits specific consideration is the expression of changes in key cardiocirculatory variables (cardiac output, muscle blood flow) relative to simultaneous changes in O2 demands, i.e., ΔO2 uptake. This was particularly important to allow inter-subject comparisons considering the semi-quantitative nature of NIRS-derived BFI (Citation24) and the potential uncertainties about the accuracy of absolute cardiac output values by impedance cardiography (Citation40). Thus, exercise-induced variations in O2 demands (elicited by different absolute work rates) were appropriately taken into consideration when we analyzed changes in cardiocirculatory responses. i.e., those subserving muscle O2 delivery. Due to the non-invasive nature of our study, however, we refrained to over-interpret these ratios (e.g., Δcardiac output /ΔO2 uptake) as indirect indexes of O2 extraction.
Responses to constant work rate rather than incremental exercise were the focus of the current study given that endurance exercise tolerance is thought to be more relevant to patients’ daily functioning than maximal exercise performance. In fact, patients with chronic cardiopulmonary disease are rarely exposed to bursts of progressive exertion to the maximum in daily life. On the other hand, the ability to sustain exercise for a “reasonable” time frame is critical to task completion. Reduced endurance exercise tolerance at 80% peak in overlap () despite the lack of differences in peak work rate () suggest important group differences in the sub-maximal power-time relationship, an interesting finding that might hold implication for targeting a tolerable work rate (e.g., exercise training).
As a minimally invasive study, the present investigation has other inherent limitations. Impedance cardiography might underestimate cardiac output in hyperinflated patients (Citation40). However, overlap patients were less hyperinflated than COPD patients suggesting that, if anything, we might have underestimated differences in cardiac output between these groups. Although NIRS-based estimations of blood flow with intra-venous ICG injections (Citation23,24) have been validated in clinical populations (Citation31), this method does not allow continuous measurements over time. We therefore chose to evaluate changes (Δ) in blood flow to alterations in metabolic demand. The close relationship between Δmuscle blood flow and Δcardiac output in healthy subjects () further increased confidence on our methodological approach. We acknowledge that COPD patients were typically hyperinflated and some developed hypoxemia. Thus, our results should not be extrapolated to less severe, non-hypoxemic patients. Nevertheless, it is conceivable that the dominance of cardiocirculatory over respiratory factors in limiting exercise tolerance in overlap would be particularly true for less severe COPD patients.
Summary and conclusions
This is the first study to demonstrate that overlap patients have lower endurance exercise tolerance compared to their COPD and heart failure counterparts matched by FEV1 and left ventricular ejection fraction, respectively. Our findings indicate that COPD and heart failure add to decrease exercising cardiac output and skeletal muscle perfusion to a greater extent than that expected by heart failure alone. Given that these abnormalities are related closely to poor exercise tolerance, increasing skeletal muscle O2 delivery and/or decreasing O2 demand might be of particular therapeutic relevance in this patient population.
Acknowledgments
The authors are indebted to all colleagues from the Pulmonary Function and Clinical Exercise Physiology Unit (SEFICE), Respiratory Division, Federal University of São Paulo (UNIFESP), São Paulo, Brazil for their collegial assistance during patients’ recruitment and data collection.
Funding
This work was supported by research grants from FAPESP (São Paulo State Research Agency, Brazil) and Canadian Foundation for Innovation-Leaders Operating Fund.
Declaration of interest statement
The authors declare that they have no conflicting interests. The authors alone are responsible for the content and writing of the article.
References
- Vestbo J, Hurd SS, Agustí AG, Jones PW, Vogelmeier C, Anzueto A, et al. Global strategy for the diagnosis, management, and prevention of chronic obstructive pulmonary disease: GOLD executive summary. Am J Respir Crit Care Med 2013; 187(4):347–365.
- O’Donnell DE, Laveneziana P, Webb K, Neder JA. Chronic obstructive pulmonary disease: clinical integrative physiology. Clin Chest Med 2014; 35(1):51–69.
- Smith MC, Wrobel JP. Epidemiology and clinical impact of major comorbidities in patients with COPD. Int J Chron Obstruct Pulmon Dis 2014; 9:871–888.
- Choudhury G, Rabinovich R, MacNee W. Comorbidities and systemic effects of chronic obstructive pulmonary disease. Clin Chest Med 2014 Mar;35(1):101–130.
- Güder G, Rutten FH. Comorbidity of heart failure and chronic obstructive pulmonary disease: more than coincidence. Curr Heart Fail Rep 2014; 11(3):337–346.
- Vogiatzis I, Zakynthinos S. The physiological basis of rehabilitation in chronic heart and lung disease. J Appl Physiol 2013; 115(1):16–21.
- Franssen FME, Rochester CL. Comorbidities in patients with COPD and pulmonary rehabilitation: do they matter? Eur Respir Rev Off J Eur Respir Soc 2014; 23(131):131–141.
- Rutten FH, Cramer M-JM, Lammers J-WJ, Grobbee DE, Hoes AW. Heart failure and chronic obstructive pulmonary disease: An ignored combination? Eur J Heart Fail 2006; 8(7):706–711.
- Chiappa GR, Borghi-Silva A, Ferreira LF, Carrascosa C, Oliveira CC, Maia J, et al. Kinetics of muscle deoxygenation are accelerated at the onset of heavy-intensity exercise in patients with COPD: relationship to central cardiovascular dynamics. J Appl Physiol 2008; 104(5):1341–1350.
- Borghi-Silva A, Oliveira CC, Carrascosa C, Maia J, Berton DC, Queiroga F, et al. Respiratory muscle unloading improves leg muscle oxygenation during exercise in patients with COPD. Thorax 2008; 63(10):910–915.
- Berton DC, Barbosa PB, Takara LS, Chiappa GR, Siqueira ACB, Bravo DM, et al. Bronchodilators accelerate the dynamics of muscle O2 delivery and utilisation during exercise in COPD. Thorax 2010; 65(7):588–593.
- Sperandio PA, Borghi-Silva A, Barroco A, Nery LE, Almeida DR, Neder JA. Microvascular oxygen delivery-to-utilization mismatch at the onset of heavy-intensity exercise in optimally treated patients with CHF. Am J Physiol Heart Circ Physiol 2009; 297(5):H1720–1728.
- Poole DC, Hirai DM, Copp SW, Musch TI. Muscle oxygen transport and utilization in heart failure: implications for exercise (in)tolerance. Am J Physiol Heart Circ Physiol 2012; 302(5):H1050–1063.
- Jörgensen K, Houltz E, Westfelt U, Nilsson F, Scherstén H, Ricksten S-E. Effects of lung volume reduction surgery on left ventricular diastolic filling and dimensions in patients with severe emphysema. Chest 2003; 124(5):1863–1870.
- Jörgensen K, Müller MF, Nel J, Upton RN, Houltz E, Ricksten S-E. Reduced intrathoracic blood volume and left and right ventricular dimensions in patients with severe emphysema: an MRI study. Chest 2007; 131(4):1050–1057.
- Aliverti A, Dellacà RL, Lotti P, Bertini S, Duranti R, Scano G, et al. Influence of expiratory flow-limitation during exercise on systemic oxygen delivery in humans. Eur J Appl Physiol 2005; 95(2–3):229–242.
- Aliverti A, Macklem PT. The major limitation to exercise performance in COPD is inadequate energy supply to the respiratory and locomotor muscles. J Appl Physiol 2008; 105(2):749–751; discussion 755–757.
- Amann M, Regan MS, Kobitary M, Eldridge MW, Boutellier U, Pegelow DF, et al. Impact of pulmonary system limitations on locomotor muscle fatigue in patients with COPD. Am J Physiol Regul Integr Comp Physiol 2010; 299(1):R314–324.
- Grassi B, Pogliaghi S, Rampichini S, Quaresima V, Ferrari M, Marconi C, et al. Muscle oxygenation and pulmonary gas exchange kinetics during cycling exercise on-transitions in humans. J Appl Physiol 2003; 95(1):149–158.
- Quanjer PH, Tammeling GJ, Cotes JE, Pedersen OF, Peslin R, Yernault JC. Lung volumes and forced ventilatory flows. Report Working Party Standardization of Lung Function Tests, European Community for Steel and Coal. Official Statement of the European Respiratory Society. Eur Respir J Suppl 1993; 16:5–40.
- Burdon JG, Juniper EF, Killian KJ, Hargreave FE, Campbell EJ. The perception of breathlessness in asthma. Am Rev Respir Dis 1982; 126(5):825–828.
- Charloux A, Lonsdorfer-Wolf E, Richard R, Lampert E, Oswald-Mammosser M, Mettauer B, et al. A new impedance cardiograph device for the non-invasive evaluation of cardiac output at rest and during exercise: comparison with the “direct” Fick method. Eur J Appl Physiol 2000; 82(4):313–320.
- Kuebler WM, Sckell A, Habler O, Kleen M, Kuhnle GE, Welte M, et al. Noninvasive measurement of regional cerebral blood flow by near-infrared spectroscopy and indocyanine green. J Cereb Blood Flow Metab Off J Int Soc Cereb Blood Flow Metab 1998; 18(4):445–456.
- Habazettl H, Athanasopoulos D, Kuebler WM, Wagner H, Roussos C, Wagner PD, et al. Near-infrared spectroscopy and indocyanine green derived blood flow index for noninvasive measurement of muscle perfusion during exercise. J Appl Physiol 2010; 108(4):962–967.
- Güder G, Rutten FH, Brenner S, Angermann CE, Berliner D, Ertl G, et al. The impact of heart failure on the classification of COPD severity. J Card Fail 2012; 18(8):637–644.
- Lalande S, Luoma CE, Miller AD, Johnson BD. Effect of changes in intrathoracic pressure on cardiac function at rest and during moderate exercise in health and heart failure. Exp Physiol 2012; 97(2):248–256.
- Olson TP, Joyner MJ, Dietz NM, Eisenach JH, Curry TB, Johnson BD. Effects of respiratory muscle work on blood flow distribution during exercise in heart failure. J Physiol 2010; 588(Pt 13):2487–2501.
- Van Baak MA. Beta-adrenoceptor blockade and exercise. An update. Sports Med Auckl NZ 1988; 5(4):209–225.
- Hepple RT. The role of O2 supply in muscle fatigue. Can J Appl Physiol Rev Can Physiol Appliquée 2002; 27(1):56–69.
- Gagnon P, Bussières JS, Ribeiro F, Gagnon SL, Saey D, Gagné N, et al. Influences of spinal anesthesia on exercise tolerance in patients with chronic obstructive pulmonary disease. Am J Respir Crit Care Med 2012; 186(7):606–615.
- Vogiatzis I, Habazettl H, Aliverti A, Athanasopoulos D, Louvaris Z, LoMauro A, et al. Effect of helium breathing on intercostal and quadriceps muscle blood flow during exercise in COPD patients. Am J Physiol Regul Integr Comp Physiol 2011; 300(6):R1549–1559.
- Gosker HR, Lencer NHMK, Franssen FME, van der Vusse GJ, Wouters EFM, Schols AMWJ. Striking similarities in systemic factors contributing to decreased exercise capacity in patients with severe chronic heart failure or COPD. Chest 2003; 123(5):1416–1424.
- Burtscher M. Exercise limitations by the oxygen delivery and utilization systems in aging and disease: coordinated adaptation and deadaptation of the lung-heart muscle axis - a mini-review. Gerontology 2013; 59(4):289–296.
- Dumitru L, Iliescu A, Dinu H, Badea R, Savulescu S, Huidu S, et al. Disability in COPD and chronic heart failure is the skeletal muscle the final common pathway? Mædica 2013; 8(2):206–213.
- Ciavaglia CE, Guenette JA, Ora J, Webb KA, Neder JA, O’Donnell DE. Does exercise test modality influence dyspnoea perception in obese patients with COPD? Eur Respir J 2014; 43(6):1621–1630.
- Esposito F, Reese V, Shabetai R, Wagner PD, Richardson RS. Isolated quadriceps training increases maximal exercise capacity in chronic heart failure: the role of skeletal muscle convective and diffusive oxygen transport. J Am Coll Cardiol 2011; 58(13):1353–1362.
- Dolmage TE, Goldstein RS. Effects of one-legged exercise training of patients with COPD. Chest 2008; 133(2):370–376.
- Sperandio PA, Oliveira MF, Rodrigues MK, Berton DC, Treptow E, Nery LE, et al. Sildenafil improves microvascular O2 delivery-to-utilization matching and accelerates exercise O2 uptake kinetics in chronic heart failure. Am J Physiol Heart Circ Physiol 2012; 303(12):H1474–1480.
- Bailey SJ, Fulford J, Vanhatalo A, Winyard PG, Blackwell JR, DiMenna FJ, et al. Dietary nitrate supplementation enhances muscle contractile efficiency during knee-extensor exercise in humans. J Appl Physiol 2010 Jul;109(1):135–148.
- Bougault V, Lonsdorfer-Wolf E, Charloux A, Richard R, Geny B, Oswald-Mammosser M. Does thoracic bioimpedance accurately determine cardiac output in COPD patients during maximal or intermittent exercise? Chest 2005; 127(4):1122–1131.