Abstract
Trefoil peptides (TFF) are constitutively expressed in the gastrointestinal tract and are involved in gastrointestinal defence and repair by promoting epithelial restitution. Although there is a general consensus regarding the pro-motogenic activity of trefoil peptides, the cellular mechanisms through which they mediate these processes are not completely understood. Pertubation of the E-cadherin/catenin complex at intercellular junctions appears to be a functional pathway through which TFF2 and TFF3 promote cell migration. Tight junction complexes seal the paracellular spaces between cells and contribute to epithelial barrier function. TFF3 peptide stimulation stabilises these junctions through upregulation of the tightening protein claudin-1 and redistribution of ZO-1 from the cytoplasm to the intercellular membrane with an increase in binding to occludin. Modulation of the functional activity and subcellular localisation of epithelial junctional adhesion molecules represent important mechanisms by which trefoil peptides may promote migration of intestinal epithelial cells in vitro and healing of mucosal damage in vivo.
INTRODUCTION
The trefoil peptides are a highly conserved family of small stable, secreted molecules that take their title from the “trefoil motif”, which is a (three-leafed-clover-shaped structure) secured by disulphide bonds. In humans to date three trefoil peptides have been identified: the single-trefoil domain containing pS2 (or TFF-1), the human spasmolytic polypeptide (TFF2) and the intestinal trefoil factor (TFF3) (CitationTaupin & Podolsky, 2003).
Although the function of trefoil peptides in humans in vivo still remains uncertain, data from several studies in vitro and in animal models have provided good evidence for their involvement in gastrointestinal defence and repair (CitationMarchbank et al., 2001; CitationRio et al., 1991; CitationPlayford et al., 1995). A role for TFF in mucosal healing and regeneration is supported by their overexpression within the gastrointestinal mucosa affected by chronic ulcerative and inflammatory lesions as in patients with inflammatory bowel diseases and gastrointestinal ulceration (CitationWright et al., 1992; CitationHoffmann, 2004). Both recombinant TTF3 and TTF2 have been shown to provide protection and they reduce the extent of ethanol- and NSAID-induced damage in vivo and in vitro (CitationBabyatsky et al., 1996). Repair of the gastrointestinal mucosa after injury occurs through a process called restitution which involves rapid migration of viable cells to cover the denuded region. Cell motility requires a dynamic interaction between cells, their extracellular matrix and the cytoskeletal network.
In epithelial cells the intercellular junctional complex includes well-defined structures such as the adherens junctions, the tight junctions and the desmosomes. This article will summarise the regulatory effects of trefoil peptides on the expression and function of the intercellular adhesion complexes and the potential implications in the prevention and healing of mucosal damage.
The E-cadherin/catenin complex, cell migration and epithelial restitution induced by the trefoil peptides
E-cadherin and its associated proteins catenins are localised mainly in the zonula adherens junctions and serve as the primary mediators of intercellular adhesion. Soluble motogenic factors such as epidermal growth factor (EGF), transforming growth factors alpha (TGF-α) and beta (TGF-β) and hepatocyte growth factor (HGF) provide the first signals inducing cell movement. Initiation and progression of cell migration and scattering on various extracellular substrates require the release of adhesive interactions between epithelial cells. This biological process has been shown to occur through disruption of the E-cadherin/catenin complex and modulation of expression, functional activity and binding specificity of the cell-matrix adhesion molecules integrins (CitationBorghi et al., 2010). Both integrins and E-cadherin are transmembrane receptors linked to the scaffolding cytoskeleton and function within a broader integrated adhesive network (CitationWeber et al., 2011).
Recombinant TFF2 and TFF3 have been shown to promote wound healing in intestinal epithelial cells through modulation of E-cadherin expression and localisation. Downregulation of membranous E-cadherin is observed in cells at the margins of mucosal ulceration during restitution in active Crohn's disease and ulcerative colitis (CitationKarayiannakis et al., 1998) regions where expression of TFF is rapidly increased (CitationWong et al., 1999, Citation2000). In the last decade different molecular mechanisms have been proposed to explain how trefoil peptides modulate the E-cadherin/catenin complex.
Early studies demonstrated that TFF3 stimulates migration through perturbation of the E-cadherin/catenin complex (CitationLiu et al., 1997). In vitro experiments showed that TFF3 induces rapid tyrosine phosphorylation of β catenin, γ catenin and the EGF receptor (EGFR) in association with a reduced membranous E-cadherin expression. This effect was reversed by the administration of tyrphostin, a competitive inhibitor of protein tyrosine kinases, with a re-establishment of normal intercellular adhesion, confirming that tyrosine phosphorylation is a key event in the changes induced by TFF3. Tyrosine phosphorylation of β catenin also modulates its role in Wnt signalling, increasing TCF-mediated transcription of genes involved in cell proliferation and migration. Thus, by up-regulating β catenin phosphorylation, TTF3 may potentially increase cell migration and proliferation in addition to reducing cell adhesion.
Recent studies provide new insight into the molecular mechanisms contributing to the modulation of the E-cadherin/catenin complex. In transfected cells, TFF3 induces downregulation of E-cadherin at transcriptional and post-transcriptional levels (CitationMeyer zum Büschenfelde et al., 2004) reflected by a reduced half-life of this molecule. Using an in vitro restitution model Dürer et al. demonstrated that TFF3 treatment triggers E-cadherin internalisation in cells behind the wound margins and in migrating cells (CitationDürer et al., 2007). Although internalisation of E-cadherin is also seen with another epithelial repairing factor, EGF, detailed analysis of the cell migration pattern revealed that unlike EGF treatment TFF3 induced formation of a continuous sheet of migrating cells which more efficiently covered the damaged area (CitationDürer et al., 2007).
TFF and cell-matrix focal adhesions
Spatial and temporal changes in cell-cell and cell-matrix adhesion molecules have been characterised during cell migration (CitationLauffenburger & Horwitz, 1996). For cell movement to take place, a co-ordinated response to soluble factors, activated signal transduction pathways and integration of responses from different surface receptors is mandatory. Extracellular matrix (ECM) represents a universal biological glue that not only provides a mechanical support to cells and tissues but also has a dynamic function controlling cell shape, proliferation, migration and differentiation. Integrins comprise a large family of surface receptors that mediate binding with ECM proteins. In addition to their adhesive functions, integrin signalling modulates cytoskeleton organisation and induces a migratory phenotype. Interactions between integrins and the ECM proteins are accompanied by the recruitment of multiple cytoskeletal and regulatory proteins to focal adhesion sites, conferring mechanical stability to the cell. Interaction between integrins and signalling proteins is also required for their role in cell migration and proliferation.. The focal adhesion kinase (FAK) is a non-receptor kinase linked to the cytoplasmic tail of the β integrin subunit at focal adhesions. Upon activation by integrins FAK undergoes autophosphorylation and initiates a cascade of signals through Ras to ERK/MAPK, which link integrins to mitogenic signalling (CitationTaupin & Podolsky, 2003).
Unlike other growth factors or intestinal luminal products (CitationBuda et al., 2003), trefoil peptides do not affect integrin expression. Although stimulation with TFF2 and TFF3 was associated with no changes in the expression of the main collagen receptor α2β1 integrin, in vitro (CitationEfstathiou & Pignatelli, 1998), TFF treatment of adherent non-transformed and colonic cancer cells has been reported to induce FAK phosphorylation and kinase activation (CitationTaupin & Podolsky, 2003) with important implications in the regeneration phase of mucosal healing after restitution. The pro-survival signals generated could prevent detachment-induced apoptosis (anoikis) encountered in migrating cells, enabling an efficient repair and cell renewal in the wounded area. Tetraspanins, a family of four-transmembrane domain proteins, are associated with integrin receptors and they regulate various aspects of integrin functions such as adhesion and motility (CitationHemler, 2005) and are thus potential mediators of TFF effects. Indeed, Vangl1, a member of the tetraspanin protein family, has been reported to mediate the TFF3-induced migratory response during wound healing promoting cell motility in normal human colon and intestinal cell lines (CitationKalabis et al., 2006).
Trefoil peptides, tight junction complexes and integrity of the mucosal barrier
The establishment of transgenic mouse models that chronically overexpress TFF3 or are TFF3-deficient (Tff3−/−) provide further insight into the function and the effect of this trefoil peptide under both basal conditions and after chemical-induced intestinal damage (CitationMarchbank et al., 2001). Mice overexpressing TFF3 in the intestinal mucosa were more resistant to epithelial damage induced by indomethacin and this protective effect was not mediated by differences in mucosal cell proliferation or the rate of migration. Ussing chamber measurements revealed an increased baseline electrical resistance in the jejunal region expressing TFF3, suggesting a possible effect on tight junction complexes (CitationMarchbank et al., 2001).
The molecular mechanisms underlying this TFF-induced increase in electrical resistance have not been extensively studied, but one possible contributory factor has been suggested from studies on epithelial cell-lines transfected with TFF3 which revealed changes in claudin expression (CitationMeyer zum Büschenfelde et al., 2006). Claudins are tetraspanin membrane proteins which play a critical role in the regulation of tight junction permeability (CitationTurksen & Troy, 2004). The cytoplasmic domains of claudins and occludin bind together and interact with the cytoplasmic ZO-1, ZO-2 and ZO-3 at the inner surface of the plasma membrane. In both HT29/B6 colon carcinoma cells and MDCK kidney cells CitationMeyer zum Büschenfelde et al. (2006) found that TFF-3 expression increased the protein level of the tightening claudin-1 and decreased the channel forming claudin-2, effects which coincided with increased transepithelial resistance (TER), a commonly used measure of the ability of tight junctions to block paracellular ion flow. The TFF3-induced changes in claudin expression were not accompanied by changes in either ZO-1, ZO-2 or occludin levels.
We have extended these studies by examining the consequences of recombinant TFF3 stimulation on epithelial barrier function and the structural organisation of the tight junction molecules occludin and ZO-1 using intestinal epithelial cell monolayers. We have used the Caco-2 cell line, derived from a human colon adenocarcinoma, that exhibits several morphological and functional characteristics of mature enterocytes and has been extensively utilised to study intestinal epithelial permeability. We observed increased TER values in Caco-2 monolayers after 24 hours stimulation with rTFF3 (330 ± 23 vs 450 ± 14 ohms.cm2, control and TFF3 treated, respectively p < 0.01), suggesting enhanced epithelial barrier properties. In agreement with studies on TFF3-transfected MDCK and HT29/B6 cells (CitationMeyer zum Büschenfelde et al., 2006), Western blotting revealed no significant changes in ZO-1 and occludin total protein levels in TFF3-treated Caco-2 cells (). The interaction between occludin and ZO-1 plays a crucial role in maintaining the structure of tight junctions and epithelial barrier functions. In order to evaluate the effect of rTFF3 on this interaction, we analysed the ZO-1/occludin complex by immunoprecipitation. After stimulation with rTFF3 a significant increase in the ZO-1 level was detected in anti-occludin immunoprecipitates (b). Compared to control (a–c), in TFFR3-treated monolayers, immunofluorescence staining and confocal microscopy showed a redistribution of ZO-1 from the cytoplasmic compartment to the intercellular junctions (a) with an increased intensity of the level of co-localisation when double-labelling experiments for ZO-1 and occludin were performed (c). These results suggest a regulatory effect of TFF3 on tight junction function through a selective modulation of ZO-1 cellular localisation.
Figure 1. (a) ZO-1 and occludin protein expression after TFF3 stimulation. Lysate from control (A) and TFF3-treated (B) monolayers were resolved by sodium dodecyl sulphate polyacrylamide gel electrophoresis. No changes in total protein levels were observed after TFF3 treatment. (b) Association of ZO-1 with occludin after TFF3 stimulation. Immunoprecipitation was performed with anti occludin antibody (Zymed) and precipitated proteins were examined by Western analysis. Compared to control (C), an increase in the total proportion of ZO-1 precipitated with occludin was observed after stimulation with TFF3 (10−9 M) (D). A: ZO-1 lysate only; B: beads only.
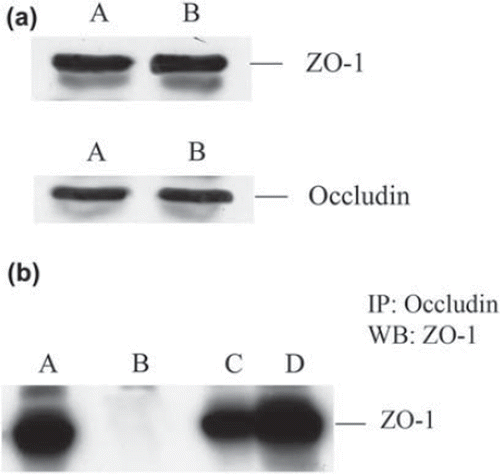
Figure 2. Expression and subcellular localisation of ZO-1 and occludin in unstimulated and TFF3-treated Caco-2 monolayers by confocal microscopy. (A) and (B) show ZO-1 (red) and occludin (green) immunolabelling. (C) shows an overlay of the two channels revealing co-localisation (yellow) of ZO-1 and occludin proteins at the cell membrane and cytoplasmic ZO-1 staining (magnification × 500). Compared to control, treated cells showed a greater degree of co-localisation (yellow) and a relocation of ZO-1 from the cytoplasmic to the cell membrane compartment.
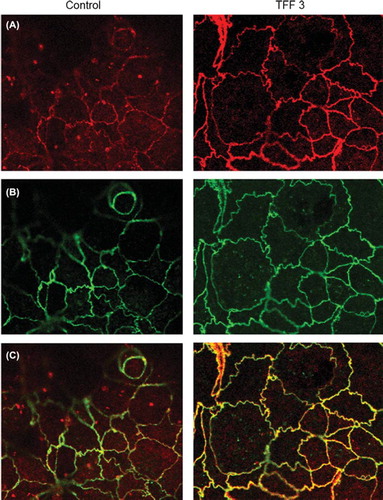
It has been shown that the inhibition of E-cadherin function prevents the assembly of tight junctions and desmosomes in vitro (CitationWatabe et al., 1994). At first glance our findings of an increased extent of tight junction functions after TFF3 stimulation may appear in contradiction with the disruption of the E-cadherin/catenin complex and the reduced E-cadherin membranous expression described previously (CitationLiu et al., 1997; Meyer zum Buschenfelde et al., 2004; CitationDürer et al., 2007). However, two models for how E-cadherin adhesion can control tight junction formation have been proposed. The first model suggests that the E-cadherin/catenin complex physically holds two neighbouring cells together so that tight junctions can be formed (CitationWatabe et al., 1994). The second model instead hypothesised that E-cadherin can act from afar, triggering intracellular signalling pathways which in turn activate tight junction assembly (CitationBalda et al., 1993). Inhibition of E-cadherin function by overexpression of a mutant form of the protein has been shown to increase tight junction assembly (CitationTroxell et al., 2000) and functional tight junctions have been shown to develop in the absence of E-cadherin-mediated cell–cell adhesions in hepatic cells (CitationThéard et al., 2007). This evidence suggests that the hierarchy of events controlling tight junction formation and functions are not absolute or linear and the presence of E-cadherin at the cell membrane is dispensable for TJ formation. Disruption of adherens junctions could release signalling molecules or activate pathways stimulating tight junction assembly (CitationTroxell et al., 2000).
Conclusions
It is now well established that trefoil peptides are cytoprotective and promote healing after gastrointestinal damage. However, there is no general agreement about their mode of action on epithelial cells and both extracellular and intracellular actions have been hypothesised. In vitro evidence has shown that trefoil peptides promote cell migration and are therefore thought to promote restitution after damage. Cell–cell junctional proteins and cell–matrix adhesive interactions are common targets for motogenic factors and in both these families of adhesion molecules control of cell migration is thought to involve intimate interactions between the transmembrane receptors and the cytoskeletal motility apparatus. TFF3 induces rapid phosphorylation of the cytoskeletal molecule β catenin leading to the dissociation of the E-cadherin/catenin complex from the underlying actin cytoskeleton, destabilisation of adherens junctions and promotion of cell migration. It can be speculated that this interaction may activate or influence integrin signal transduction pathways and trigger the cascade of events involved in cell migration.
Several lines of evidence from animal studies, including transgenic models, have provided important information on the protective effects of trefoil peptides against mucosal damage. Tight junctions are highly dynamic structures which maintain the barrier function of the intestinal epithelium. In the presence of trefoil peptides mucosal integrity is enhanced and we have shown that stimulation with TFF3 increases tight junction function and assembly in vitro. This effect could contribute to mucosal protection from injury by the many potentially damaging macromolecules and agents present in the intestinal lumen.
METHODS
Cell culture
The colonic epithelial cell line Caco-2 purchased from the European tissue culture collection (ETCC) was used for the study. Caco-2 cells were grown in Dulbecco's MEM (DMEM) (Gibco, UK) supplemented with 10% foetal calf serum. They were incubated in flasks at 37°C in a humidified atmosphere of 5% CO2 and split with trypsin-EDTA solution (Gibco, UK).
Transepithelial resistance (TER) analysis
The cells were seeded on polycarbonate filters (pore diameter of 3 μm) (Costar, The Netherlands) at a concentration of 1.1–1.4 × 105 cells/ml. Fourteen-day-old Caco-2 monolayers were pre-incubated in serum-free medium for 12 hours and then incubated in the presence or absence of TFF3 10‐9 M for 6 hours. TER of the Caco-2 cell monolayers was measured using an EVOM epithelial volt-ohm metre and STX2 electrode (World Precision Instruments, FL, USA). Experiments were carried out in triplicate.
Immunoprecipitation and Western blotting
Cell lysis was performed on ice in the presence of a cocktail of protease inhibitors (Aprotinin 0.1–2 μg/ml, Leupeptin 0.5–2 μg/ml, PMSF 20–100 μg/ml, Trypsin-Chymotrypsin inhibitor 10 μg/ml, TPCK 100 μg/ml, all from Sigma). Pre-clearing of cell extract was performed using mouse and rabbit IgG to reduce non-specific binding. Protein G sepharose beads (Pharmacia Biotech, St. Albans UK) were pre-washed in lysis buffer and incubated overnight with cell extract in the presence of the immunoprecipitating antibody at 4°C. The precipitated proteins were eluted from beads under reducing conditions by boiling in Laemmli sample buffer for 5 minutes. Composition of eluted protein complexes was analysed by SDS-PAGE and Western blotting as previously described (Buda, 2003). Sample protein concentration was determined by BioRad protein assay (BioRad, Hemel Hempstead, UK).
Immunofluorescence and confocal microscopy analysis
Treated and control cells were fixed in 4% (w/v) paraformaldehyde and permeabilised with Triton X 100 (0.05%) (Sigma). ZO-1 was immunofluorescently labelled with rabbit polyclonal antibody (Zymed, USA) followed by Alexa Fluor 568 goat anti rabbit F(Ab)2 fragments (Molecular Probes, USA). For detection of occludin, cells were incubated with mouse monoclonal antibody (Zymed, USA) followed by Alexa Fluor 488 goat anti mouse F(Ab)2 fragments (Molecular Probes, USA). Cell monolayers on Transwell filters were mounted onto glass slides with Vectashield (Vector, Burlington, USA) before viewing on a Leica TCS-NT confocal scanning microscope attached to a Leica DM IRBE inverted epifluorescence microscope (Leica Microsystems, Mannheim, Germany) using a 63X oil-immersion lens and imaging parameters to optimise resolution.
Declaration of interest: The authors report no conflicts of interest. The authors alone are responsible for the content and writing of the article.
REFERENCES
- Babyatsky MW, deBeaumont M, Thim L, Podolsky DK (1996). Oral trefoil peptides protect against ethanol- and indomethacin-induced gastric injury in rats. Gastroenterology. 110:489–497.
- Balda MS, Gonzalez-Mariscal L, Matter K, Cereijido M, Anderson JM (1993). Assembly of the tight junction: the role of diacylglycerol. J Cell Biol. 123:293–302.
- Borghi N, Lowndes M, Maruthamuthu V, Gardel ML, Nelson WJ (2010). Regulation of cell motile behavior by crosstalk between cadherin- and integrin-mediated adhesions. Proc Natl Acad Sci U S A. 107:13324–13329.
- Buda A, Qualtrough D, Jepson MA, Martines D, Paraskeva C, Pignatelli M (2003). Butyrate downregulates alpha2beta1 integrin: a possible role in the induction of apoptosis in colorectal cancer cell lines. Gut. 52:729–734.
- Dürer U, Hartig R, Bang S, Thim L, Hoffmann W (2007). TFF3 and EGF induce different migration patterns of intestinal epithelial cells in vitro and trigger increased internalization of E-cadherin. Cell Physiol Biochem. 20:329–346.
- Efstathiou JA, Pignatelli M (1998). Modulation of epithelial cell adhesion in gastrointestinal homeostasis. Am J Pathol. 153:341–347.
- Hemler ME (2005). Tetraspanin functions and associated microdomains. Nat Rev Mol Cell Biol. 6:801–811.
- Hoffmann W (2004). Trefoil factor family (TFF) peptides: regulators of mucosal regeneration and more. Peptides 25:727–730.
- Kalabis J, Rosenberg I, Podolsky DK (2006). Vangl1 protein acts as a downstream effector of intestinal trefoil factor (ITF)/TFF3 signaling and regulates wound healing of intestinal epithelium. J Biol Chem. 281:6434–6441.
- Karayiannakis AJ, Syrigos KN, Efstathiou J, Valizadeh A, Noda M, Playford RJ, Kmiot W, Pignatelli M (1998). Expression of catenins and E-cadherin during epithelial restitution in inflammatory bowel disease. J. Pathol. 185:413–418.
- Lauffenburger DA, Horwitz AF (1996). Cell migration: a physically integrated molecular process. Cell. 84:359–369.
- Liu D, el-Hariry I, Karayiannakis AJ, Wilding J, Chinery R, Kmiot W, McCrea PD, Gullick WJ, Pignatelli M (1997). Phosphorylation of beta-catenin and epidermal growth factor receptor by intestinal trefoil factor. Lab Invest. 77:557–563.
- Marchbank T, Cox HM, Goodlad RA, Giraud AS, Moss SF, Poulsom R, Wright NA, Jankowski J, Playford RJ (2001). Effect of ectopic expression of rat trefoil factor family 3 (intestinal trefoil factor) in the jejunum of transgenic mice. J Biol Chem. 276:24088–24096.
- Meyer zum Büschenfelde D, Hoschützky H, Tauber R, Huber O (2004). Molecular mechanisms involved in TFF3 peptide-mediated modulation of the E-cadherin/catenin cell adhesion complex. Peptides. 25:873–883.
- Meyer zum Büschenfelde D, Tauber R, Huber O (2006). TFF3-peptide increases transepithelial resistance in epithelial cells by modulating claudin-1 and -2 expression. Peptides. 27:3383–3390.
- Playford RJ, Marchbank T, Chinery R, Evison R, Pignatelli M, Boulton RA, Thim L, Hanby AM (1995). Human spasmolytic polypeptide is a cytoprotective agent that stimulates cell migration. Gastroenterology. 108:108–116.
- Rio MC, Chenard MP, Wolf C, Marcellin L, Tomasetto C, Lathe R, Bellocq JP, Chambon P (1991). Induction of pS2 and hSP genes as markers of mucosal ulceration of the digestive tract. Gastroenterology. 100:375–379.
- Taupin D, Podolsky DK (2003). Trefoil factors: initiators of mucosal healing. Nat Rev Mol Cell Biol. 4:721–732.
- Théard D, Steiner M, Kalicharan D, Hoekstra D, van Ijzendoorn SC (2007). Cell polarity development and protein trafficking in hepatocytes lacking E-cadherin/beta-catenin-based adherens junctions. Mol Biol Cell. 18:2313–2321.
- Troxell ML, Gopalakrishnan S, McCormack J, Poteat BA, Pennington J, Garringer SM, Schneeberger EE, Nelson WJ, Marrs JA (2000). Inhibiting cadherin function by dominant mutant E-cadherin expression increases the extent of tight junction assembly. J Cell Sci. 113:985–996.
- Turksen K, Troy TC (2004). Barriers built on claudins. J Cell Sci. 117:2435–2447.
- Watabe M, Nagafuchi A, Tsukita S, Takeichi M (1994). Induction of polarized cell-cell association and retardation of growth by activation of the E-cadherin-catenin adhesion system in a dispersed carcinoma line. J Cell Biol. 127:247–256.
- Weber GF, Bjerke MA, DeSimone DW (2011). Integrins and cadherins join forces to form adhesive networks. J Cell Sci. 124:1183–1193.
- Wong WM, Playford RJ, Wright NA (2000). Peptide gene expression in gastrointestinal mucosal ulceration: ordered sequence or redundancy? Gut. 46:286–292.
- Wong WM, Poulsom R, Wright NA (1999). Trefoil peptides. Gut. 44:890–895.
- Wright NA, Poulsom R, Stamp G, Van Norden S, Sarraf C, Elia G, Ahnen D, Jeffery R, Longcroft J, Pike C, Rio MC, Chambon P. (1992). Trefoil peptide gene expression in gastrointestinal epithelial cells in inflammatory bowel disease. Scand J Gastroenterol Suppl. 193:76–82.