Abstract
Gap junctions are a specialized group of cell-to-cell junctions that mediate direct intercellular communication between cells. They arise from the interaction of two hemichannels of adjacent cells, which in turn are composed of six connexin proteins. In liver, gap junctions are predominantly found in hepatocytes and play critical roles in virtually all phases of the hepatic life cycle, including cell growth, differentiation, liver-specific functionality and cell death. Liver gap junctions are directed through a broad variety of mechanisms ranging from epigenetic control of connexin expression to post-translational regulation of gap junction activity. This paper reviews established and novel aspects regarding the architecture, control and functional relevance of liver gap junctions.
Keywords:
INTRODUCTION
As holds for all multicellular systems, homeostasis in liver is controlled by the entangled network of extracellular, intracellular and intercellular communication mechanisms. Typically, extracellular signals trigger intracellular signal transduction cascades. Several of these intracellular signals are subsequently propagated to adjacent cells. Such intercellular connections are formed by arrays of gap junctions (Vinken et al., Citation2006b, Citation2008,Citation2009b). The liver was among the first organs in which gap junctions were studied (Loewenstein & Kanno, Citation1967; Revel & Karnovsky, Citation1967). In 1974, Goodenough isolated two gap junction proteins from mouse liver, which were designated connexins (Goodenough, Citation1974). Today, more than 20 different connexin species have been identified. They are all named based upon their molecular weight as predicted by cDNA sequencing and are expressed in a cell type-specific way (Bai & Wang, Citation2014). Nevertheless, they all share a similar structure consisting of four transmembrane domains, two extracellular loops, one cytosolic loop, one cytosolic carboxyterminal tail and one cytosolic aminotail. Following their synthesis, six connexins form a hemichannel or connexon at the plasma membrane surface, which then docks with another hemichannel from a neighboring cell to generate a gap junction () (Vinken et al., Citation2006b, Citation2008, Citation2009b). Gap junctions mediate the passive diffusion of small, i.e. below 1.5 kDa, and hydrophilic molecules, such as glucose, glutamate, glutathione, adenosine triphosphate, cyclic adenosine monophosphate, inositol triphosphate and ions, including calcium, sodium and potassium (Alexander & Goldberg, Citation2003; Decrock et al., Citation2009). Numerous physiological processes are regulated by substances that are intercellularly exchanged via gap junctions and hence gap junctional intercellular communication (GJIC) is considered as a key mechanism in the control of tissue homeostasis (Vinken et al., Citation2006b, Citation2008, Citation2009b). Because of this critical task, GJIC is strictly regulated at multiple levels by a myriad of mechanisms. In this paper, a state-of-the-art overview of the structure, regulation and function of gap junctions in liver is provided.
Figure 1. Structure of gap junctions. Gap junctions are formed by the docking of two hemichannels or connexons from neighboring cells, which in turn are built up by six connexins. Connexins share a similar structure consisting of four transmembrane domains (TM), two extracellular loops (EL), one cytosolic loop (CL), one cytosolic carboxyterminal tail (COOH) and one cytosolic aminotail (NH2).
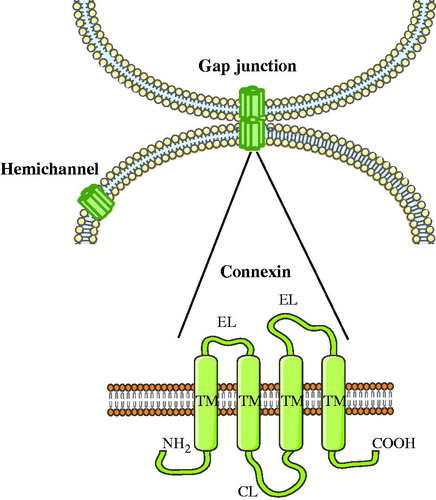
STRUCTURE OF LIVER GAP JUNCTIONS
The major connexin species in liver is Cx32, which represents 90% of the total hepatic connexin content (Cascio et al., Citation1995; Neveu et al., Citation1995). Cx32 is expressed by hepatocytes and to a lesser extent by sinusoidal endothelial cells. These cells as well as stellate cells and Kupffer cells also produce small quantities of Cx26 (Fischer et al., Citation2005), while Cx43 is detectable in Kupffer cells, stellate cells, sinusoidal endothelial cells, cells of Glisson’s capsule and cholangiocytes (Berthoud et al., Citation1992; Bode et al., Citation2002; Fischer et al., Citation2005; Greenwel et al., Citation1993; Sáez, Citation1997). Furthermore, Cx40 and Cx37 are present in liver vascular cells () (Shiojiri et al., Citation2006). Despite the wide repertoire of connexins expressed in liver, the presence of functional gap junctions has only been demonstrated in hepatocytes and stellate cells (Fischer et al., Citation2005). Unlike Cx32, which is uniformly distributed in liver tissue, Cx26 is preferentially expressed in the periportal area (Spray et al., Citation1994). Hence, most gap junctions in the pericentral and periportal regions are Cx32 homotypic and Cx32–Cx26 heterotypic channels, respectively (Iwai et al., Citation2000). Gap junctions occupy about 3% of the hepatocyte membrane surface (Spray et al., Citation1994) and are organized in plaques of 0.2–1 μm in diameter (Kojima et al., Citation1996) that contains 10–10,000 channels (Musil et al., Citation2000). Connexin proteins usually display rapid turnover rates in comparison with other plasma membrane proteins. Both in vitro, i.e. primary cultured hepatocytes (Traub et al., Citation1987), and in vivo, i.e. regenerating liver (Traub et al., Citation1983), the half-lives of Cx26 and Cx32 have been found to be 2 and 3 h, respectively, whereas the turnover times of other integral membrane proteins in primary hepatocyte cultures generally range from 17 to 100 h (Chu & Doyle, Citation1985). Upon degradation, gap junctional channels are internalized by one of the two opposing cells, resulting in the formation of so-called annular gap junction. These structures are further degraded by both lysosomes and proteasomes, although the precise degradation pathway depends on the identity of the connexin and cell type (Laird, Citation2005, Citation2006). Degradation of Cx32-based gap junctions in rat liver mainly occurs via the lysosomal pathway (Rahman et al., Citation1993).
Table 1. Expression of connexins in liver.
REGULATION OF LIVER GAP JUNCTIONS
Short-term regulation
Short-term control of GJIC, so-called gating, is driven by a number of factors, including pH, transmembrane voltage and calcium concentration (Cottrell & Burt, Citation2005). Post-translational modifications, such as S-nitrosylation, sumoylation and phosphorylation, also directly regulate gap junction opening (Johnstone et al., Citation2012). Phosphorylation mainly occurs at the cytoplasmic carboxyterminal connexin tail. In fact, all connexins are phosphoproteins with the notable exception of Cx26. The regulation of gap junction opening by phosphorylation is complex, as the outcome of this post-translational modification depends on the nature of the kinase and connexin as well as on the cellular context (Laird, Citation2005; Solan & Lampe, Citation2005). Cx43 has been most extensively studied in this regard. Cx43 is a substrate for many kinases, including protein kinases A and C, members of the mitogen-activated protein kinase family, casein kinase 1, the cyclin-dependent kinase 1/cyclin B complex and v-Src (Solan & Lampe, Citation2005, Citation2009). Different from other connexins, shifts in electrophoretic mobility occur upon phosphorylation of Cx43. Usually, three bands appear during sodium dodecylsulfate–polyacrylamide gel electrophoresis analysis, representing the fast-migrating nonphosphorylated Cx43 isoform, referred to as NP-Cx43, and two slow-migrating phosphorylated Cx43 isoforms, namely P1-Cx43 and P2-Cx43 (Cooper et al., Citation2000; Solan & Lampe, Citation2005, Citation2009). In liver, Cx43 is predominantly presented in its NP-Cx43 isoform (Vinken et al., Citation2006a, Citation2012b). Cx32 can be phosphorylated by protein kinases A and C, the epidermal growth factor receptor and calcium-calmodulin-dependent protein kinase II (Lampe & Lau, Citation2004). While phosphorylation by protein kinase A promotes hepatocellular GJIC (Saez et al., Citation1986), phosphorylation by protein kinase C prevents calpain-mediated proteolysis (Elvira et al., Citation1993). Gap junction activity can also be affected by connexin binding partners. Connexins indeed interact with a plethora of proteins, referred to as the interactome. For instance, assembly of adherens junctions composed of E-cadherin and α-catenin at the hepatocyte cell plasma membrane surface is a prerequisite for the formation of Cx32-based gap junctions (Fujimoto et al., Citation1997).
Long-term regulation
Long-term control of GJIC involves regulation at the transcriptional level of connexin expression (Oyamada et al., Citation2005). The architecture of most connexin genes is simple, consisting of a first exon (E1) that harbors the 5′-untranslated region (UTR), which is separated by an intron of varying length from a second exon (E2), bearing the complete coding sequence and the 3′-UTR. The Cx32 gene is exceptional because of differential splicing of the 5′-UTR (Oyamada et al., Citation2005; Söhl & Willecke, Citation2004). The human and rat Cx32 genes comprise three exons, i.e. E1, E1B and E2, whereas their mouse and bovine counterparts contain four exons, i.e. E1, E1A, E1B and E2 (Duga et al., Citation1999; Neuhaus et al., Citation1996; Söhl et al., Citation2001). Cx32 gene transcription can be initiated through two tissue-specific promoters. The P1 promoter, located upstream of E1, is active in hepatocytes and pancreatic secretory acinar cells, generating the E1–E2 transcript, whereas the P2 promoter, which is located upstream of E1B, is active in peripheral nerves and yields the E1B–E2 transcript (Neuhaus et al., Citation1996; Söhl et al., Citation1996). In mice and cows, a third transcript, i.e. E1A–E2, is found in embryonic cells, oocytes and adult liver (Duga et al., Citation1999; Neuhaus et al., Citation1996). Such differential promoter usage and alternative splicing have also been reported for the murine and rat Cx43 genes (Pfeifer et al., Citation2004). Connexin gene promoters show binding affinity for several general transcription factors, including activator protein 1, yin yang 1 and specificity protein 1 (Oyamada et al., Citation2005). In parallel, a number of cell type-specific transcription factors control connexin gene transcription. Thus, liver-specific expression of Cx32 relies on the binding of hepatocyte nuclear factor 1α at the P1 promoter (Field et al., Citation2003; Koffler et al., Citation2002). In the last decade, epigenetic mechanisms, including histone acetylation and DNA methylation, have also joined in as master regulators of connexin expression (Oyamada et al., Citation2005; Vinken et al., Citation2009a). In this respect, the absence of Cx43 and Cx32 expression in rat MH1C1 hepatoma cells and rat WB-F344 liver epithelial cells, respectively, is causally linked to DNA methylation in the corresponding gene promoters (Piechocki et al., Citation1999). Furthermore, inhibition of histone deacetylation positively affects GJIC in primary hepatocyte cultures, which is associated with differential effects on the expression of Cx26, Cx32 and Cx43 (Vinken et al., Citation2006a, Citation2007).
FUNCTION OF LIVER GAP JUNCTIONS
Role in proliferation
The adult liver displays very low proliferative activity. However, upon partial hepatectomy, the remaining hepatic lobes start to grow and the original size becomes restored within 7–10 days (Taub, Citation2004). During this cell cycling event, GJIC transiently increases in the G1 phase, followed by a dramatic decrease upon initiation of the S phase (Dermietzel et al., Citation1987; Fladmark et al., Citation1997; Koenig et al., Citation2006; Kojima et al., Citation2003; Kren et al., Citation1993; Meyer et al., Citation1981; Miyashita et al., Citation1991; Sugiyama & Ohta, Citation1990; Temme et al., Citation2000; Traub et al., Citation1983; Yee & Revel, Citation1978). Similar alterations are seen in Cx32 expression, whereas Cx43 production remains unchanged (Kren et al., Citation1993; Temme et al., Citation2000; Traub et al., Citation1983). These findings can be reproduced in an in vitro model of hepatocyte proliferation, namely mitogen-stimulated primary hepatocytes (Fladmark et al., Citation1997; Kojima et al., Citation1997, Citation2004). In this system, mitogen-activated protein kinase phosphorylates Cx32, resulting in its decreased expression (Kojima et al., Citation2004). In fact, connexin phosphorylation seems to be a major mechanism underlying GJIC alterations in liver cell cycling. In serum-stimulated rat liver cells, progression from the G0 state to the S phase is related to protein kinase C-dependent phosphorylation of Cx43 and disruption of GJIC (Koo et al., Citation1997). The relevance of altered GJIC during cell cycling is unclear. In the regenerating liver of rats treated with an inhibitor of mitogen-activated protein kinase, the disappearance of Cx32 is inhibited without affecting hepatocyte proliferative activity (Kojima et al., Citation2003). This suggests that downregulation of gap junctions can occur independently of proliferation and hence may be considered as a fortuitous side effect of the growth response. On the other hand, in the regenerating liver of Cx32 knock-out mice, the G0/S transition of the cell cycle and thereby the proliferative activity of the hepatocytes are not promoted, but the extent of synchronous initiation and termination of DNA synthesis is decreased (Dagli et al., Citation2004; Sugiyama & Ohta, Citation1990). In this view, reduction of GJIC does not provide a direct signal for cells to divide per se, but rather permits cell cycle progression upon mitogenic stimulation. GJIC hereby seems to be coordinated with cell growth and serves a purpose other than triggering proliferation. Such a purpose may include the functional segregation of the metabolic pools in dividing cells from their quiescent neighbors in order to avoid homeostatic imbalance (Chipman et al., Citation2003; Dermietzel et al., Citation1987; Fladmark et al., Citation1997). Others strongly believe that gap junctions fulfill a determinate function in cell proliferation control rather than merely an assisting role in growth progression. Gap junctions indeed provide a pathway for the direct exchange of essential growth mediators, such as cyclic adenosine monophosphate (Vinken et al., Citation2012a). Interfering with connexin gene expression often reveals additional mechanisms involved in gap junction-mediated control of cell proliferation. In this context, transfection of liver-derived cell lines with connexin genes can directly alter gene expression patterns. Forced expression of Cx32 and Cx26 in rat liver epithelial cells and human hepatoma cells triggers the production of p27 and E-cadherin, respectively, which, in turn, negatively affect proliferation (Paul, Citation1986). Furthermore, connexins can physically interact with cell growth regulators. The scaffolding protein Discs large homolog 1 (Dlgh1) acts as a tumor suppressor protein and its presence at the cell plasma membrane surface, bound to Cx32, is associated with cell cycle arrest. Upon its release, occurring upon downregulation of Cx32 expression, Dlgh1 translocates to the cell nucleus, ultimately resulting in induction of proliferation. Therefore, maintaining Dlgh1 at the cell plasma membrane surface may be a regulatory mechanism by which Cx32 controls hepatocyte proliferation (Duffy et al., Citation2007).
Role in differentiation and liver-specific functionality
Early hepatic progenitor cells switch from Cx43 to Cx26 production, but especially to a Cx32 modus upon differentiation into hepatocytes (Naves et al., Citation2001; Neveu et al., Citation1995; Paku et al., Citation2004; Zhang & Thorgeirsson, Citation1994). A similar phenomenon has been observed in in vitro settings of liver differentiation, such as in liver epithelial cell line models (Rosenberg et al., Citation1996; Zhang & Thorgeirsson, Citation1994). The generation of the Cx26 zonated pattern during liver development parallels the establishment of metabolic heterogeneity in hepatocytes. In this respect, glucagon receptors are mainly found in the pericentral area, whereas the inverse is observed for their ligands (Berthoud et al., Citation1992). Glucagon is known to perform a much more pronounced inductive effect on the transcription of Cx26 genes compared with Cx32 genes (Kojima et al., Citation1995). It is therefore thought that the hepatic Cx26 zonation pattern is regulated at the transcriptional level and that glucagon is a master regulator of this event (Iwai et al., Citation2000; Kojima et al., Citation1995). In adult liver, the establishment of GJIC is critical for the maintenance of several liver-specific functions, including glycogenolysis (Stümpel et al., Citation1998), ammonia removal (Yang et al., Citation2003), albumin secretion (Yang et al., Citation2003), bile secretion (Temme et al., Citation2001) and xenobiotic biotransformation (Neveu et al., Citation1994; Shoda et al., Citation1999et al., Citation2000). Regarding the latter, both the constitutive and drug-induced expression of cytochrome P450 isoenzymes, in particular cytochrome P450 2B6 and 3A4, require the presence of Cx32-based gap junctions (Hamilton et al., Citation2001). Induction of cytochrome P450 1A1/2 and 2B1/2 simultaneously occurs with downregulation of pericentral Cx32 protein amounts in rat (Neveu et al., Citation1994; Shoda et al., Citation1999et al., Citation2000). These concomitant changes in the expression of Cx32 and cytochrome P450 isoenzymes could reflect a defense mechanism to restrict the intercellular diffusion of reactive intermediates produced through xenobiotic biotransformation (Neveu et al., Citation1994). The messengers that are conveyed through gap junctions and that affect liver functionality largely remain obscure, but an exception exists for glycogenolysis. This metabolic process is initiated by hormonal and neuronal stimuli and mainly takes place in the periportal region. Pericentral hepatocytes also show glycogenolytic activity, albeit to a much lesser extent (Saez et al., Citation2003; Stümpel et al., Citation1998). Cx32-based gap junctions hereby propagate the glycogenolytic response from the periportal to the pericentral areas. More specifically, they control the intercellular trafficking of inositol triphosphate, which activates calcium release from endoplasmic reticulum stores, in turn evoking calcium waves throughout the acinar tract (Gaspers & Thomas, Citation2005; Saez et al., Citation2003). In line with this observation is the finding that Cx32 knock-out mice show lowered blood glucose levels upon glycogenolytic stimulation (Stümpel et al., Citation1998). A similar scenario is seen in bile formation and flow, whereby ductular secretion from cholangiocytes depends on the spread of calcium waves through Cx43-containing gap junctions (Bode et al., Citation2002; Nathanson et al., Citation1999).
Role in cell death
During the early stages of apoptosis in serum-deprived cultured rat liver cells, GJIC is enhanced. This goes hand in hand with increased Cx43 expression and phosphorylation. The latter is likely to be mediated by the cyclin-dependent kinase 1/cyclin B complex, which also controls the G2/M transition of the cell cycle. Upon further progression of cell death, GJIC activity deteriorates, as evidenced by the absence of communication between apoptotic bodies (Contreras et al., Citation2004). The temporary induction of GJIC in the early phases of apoptosis could point to a role for gap junctions in the initial spread of a death wave from cell to cell. In this light, calcium ions are thought to be the killing messengers. The onset of apoptosis is generally associated with drastic alterations in the concentration of calcium, an ion that is intercellularly exchanged via gap junctions. The subsequent downregulation of GJIC could serve the reduction of the flux of toxic metabolites, such as nitric oxide and superoxide ions, and hence the protection of living cells (Contreras et al., Citation2004; Krutovskikh et al., Citation2002). Of note, compelling evidence shows that connexin hemichannels, rather than gap junctions, are involved in liver cell death. Following induction of apoptosis in primary hepatocytes, GJIC rapidly deteriorates, which is accompanied by a decay of the gap junctional Cx32 protein pool. Concomitantly, Cx32 is de novo synthesized and gathers in a hemichannel configuration. This becomes particularly evident toward the final stages of the cell death process (Vinken et al., Citation2010). Likewise, Cx43 signaling, also involving hemichannels, was found to facilitate the onset of spontaneous apoptosis in cultures of primary hepatocytes (Vinken et al., Citation2012b). Cx43 hereby interacts with mitochondrial proteins (Vinken et al., Citation2013), as equally holds true for hepatocellular Cx32 (Fowler et al., Citation2013). In fact, connexin hemichannels can be located at other subcellular compartments in addition to the plasma membrane, such as mitochondria, where they have been linked to apoptosis (Goubaeva et al., Citation2007). Furthermore, connexin signaling also plays a role in other cell death modes. Thus, gap junctions consisting of Cx26 and Cx32 have been found to synchronize chemical-induced necrosis in primary hepatocytes (Saito et al., Citation2014). Recently, GJIC was found to be involved in experimentally triggered autophagy in rat liver cells (Zou et al., Citation2015).
CONCLUSIONS AND PERSPECTIVES
The liver is a vital organ endowed with several critical tasks. In order to do so, communication between the different liver cell types is a prerequisite. Hepatocytes, the major work horses of the liver, directly communicate with each other through gap junctions. Hepatocellular gap junctions, consisting of Cx32 and Cx26, have been studied for more than half a century (Loewenstein & Kanno, Citation1967; Revel & Karnovsky, Citation1967). As a result, many roles have been attributed to these structures, including those related to liver cell growth, differentiation and cell death. In the past decade, it has become clear that the structural precursors of gap junctions, namely connexin hemichannels, may also contribute to the latter process by mediating paracrine signaling (Chandrasekhar & Bera, Citation2012; Decrock et al., Citation2009). Furthermore, a novel class of connexin-like proteins has been identified, namely the pannexins, which gather in a configuration identical to connexin hemichannels and that provide an additional pathway for communication between the cytosol of individual cells and their extracellular environment (Penuela et al., Citation2014). Of those, pannexin1 is expressed by hepatocytes (Csak et al., Citation2011; Ganz et al., Citation2011) and has been linked to apoptosis (Xiao et al., Citation2012). A major challenge now lies in the further exploration of the role of connexin and pannexin hemichannels in other aspects of the liver life cycle. The regulation of connexin and pannexin signaling also deserves further scrutiny. In particular, connexins are known to be subjected to several other post-translational modifications in addition to the currently studied ones, including glycosylation, N-acetylation, ubiquitination, lipidation, hydroxylation, methylation and deamidation (D’hondt et al., Citation2013), yet the functional relevance of these changes, in casu in the liver, is not clear. Similarly, microRNAs have been found to control connexin production at the post-transcriptional level in recent years (Vinken et al., Citation2009a), but specific information in relation to liver is currently lacking. Most importantly, the role of connexin signaling in liver pathology should be thoroughly investigated. Indeed, because of its key function as a gatekeeper of hepatic homeostasis, it is not surprising that connexin signaling is essentially involved in liver disease. Thus far, connexins and their channels have been found to underlie drug-induced acute liver failure, hepatitis, cholestasis, nonalcoholic fatty liver disease, fibrosis, cirrhosis and hepatocellular carcinoma (Maes et al., Citation2015; Vinken et al., Citation2013). Upon introduction of drugs that modulate connexin expression or channel activity, this may open new perspectives for the establishment of new therapies in the hepatology field in the upcoming years.
Disclosure statement
The authors report no declarations of interest. This work was financially supported by grants from the European Research Council (ERC Starting Grant 335476), the Fund for Scientific Research-Flanders (FWO grants G009514N and G010214N), the University Hospital of the Vrije Universiteit Brussel-Belgium (“Willy Gepts Fonds” UZ-VUB), the US National Institutes of Health (R01 DK102142), the University of São Paulo-Brazil and the Foundation for Research Support of the State of São Paulo-Brazil (FAPESP SPEC grant 2013/50420-6).
References
- Alexander DB and Goldberg GS (2003). Transfer of biologically important molecules between cells through gap junction channels. Curr Med Chem. 10: 2045–2058.
- Bai D and Wang AH (2014). Extracellular domains play different roles in gap junction formation and docking compatibility. Biochem J. 458: 1–10.
- Balasubramaniyan V, Dhar DK, Warner AE, Vivien Li WY, Amiri AF, Bright B, Mookerjee RP, Davies NA, Becker DL, and Jalan R (2013). Importance of Connexin-43 based gap junction in cirrhosis and acute-on-chronic liver failure. J Hepatol. 58: 1194–1200.
- Berthoud VM, Iwanij V, Garcia AM, and Sáez JC (1992). Connexins and glucagon receptors during development of rat hepatic acinus. Am J Physiol. 263: G650–G658.
- Bode HP, Wang L, Cassio D, Leite MF, St-Pierre MV, Hirata K, Okazaki K, Sears ML, Meda P, Nathanson MH, et al. (2002). Expression and regulation of gap junctions in rat cholangiocytes. Hepatology. 36: 631–640.
- Cascio M, Kumar NM, Safarik R, and Gilula NB (1995). Physical characterization of gap junction membrane connexons (hemi-channels) isolated from rat liver. J Biol Chem. 270: 18643–18648.
- Chandrasekhar A and Bera AK (2012). Hemichannels: Permeants and their effect on development, physiology and death. Cell Biochem Funct. 30: 89–100.
- Chaytor AT, Martin PE, Edwards DH, and Griffith TM (2001). Gap junctional communication underpins EDHF-type relaxations evoked by ACh in the rat hepatic artery. Am J Physiol Heart Circ Physiol. 280: H2441–H2450.
- Chipman JK, Mally A, and Edwards GO (2003). Disruption of gap junctions in toxicity and carcinogenicity. Toxicol Sci. 71: 146–153.
- Chu FF and Doyle D (1985). Turnover of plasma membrane proteins in rat hepatoma cells and primary cultures of rat hepatocytes. J Biol Chem. 260: 3097–3107.
- Cogliati B, Da Silva TC, Aloia TP, Chaible LM, Real-Lima MA, Sanches DS, Matsuzaki P, Hernandez-Blazquez FJ, and Dagli ML (2011). Morphological and molecular pathology of CCL4-induced hepatic fibrosis in connexin43-deficient mice. Microsc Res Technol. 74: 421–429.
- Contreras JE, Sánchez HA, Véliz LP, Bukauskas FF, Bennett MV, and Sáez JC (2004). Role of connexin-based gap junction channels and hemichannels in ischemia-induced cell death in nervous tissue. Brain Res Brain Res Rev. 47: 290–303.
- Cooper CD, Solan JL, Dolejsi MK, and Lampe PD (2000). Analysis of connexin phosphorylation sites. Methods. 20: 196–204.
- Cottrell GT and Burt JM (2005). Functional consequences of heterogeneous gap junction channel formation and its influence in health and disease. Biochim Biophys Acta. 1711: 126–141.
- Csak T, Ganz M, Pespisa J, Kodys K, Dolganiuc A, and Szabo G (2011). Fatty acid and endotoxin activate inflammasomes in mouse hepatocytes that release danger signals to stimulate immune cells. Hepatology. 54: 133–144.
- Dagli ML, Yamasaki H, Krutovskikh V, and Omori Y (2004). Delayed liver regeneration and increased susceptibility to chemical hepatocarcinogenesis in transgenic mice expressing a dominant-negative mutant of connexin32 only in the liver. Carcinogenesis. 25: 483–492.
- Decrock E, Vinken M, De Vuyst E, Krysko DV, D'Herde K, Vanhaecke T, Vandenabeele P, Rogiers V, and Leybaert L (2009). Connexin-related signaling in cell death: To live or let die? Cell Death Differ. 16: 524–536.
- D’hondt C, Iyyathurai J, Vinken M, Rogiers V, Leybaert L, Himpens B, and Bultynck G (2013). Regulation of connexin- and pannexin-based channels by post-translational modifications. Biol Cell. 105: 373–398.
- Dermietzel R, Yancey SB, Traub O, Willecke K, and Revel JP (1987). Major loss of the 28-kD protein of gap junction in proliferating hepatocytes. J Cell Biol. 105: 1925–1934.
- Duffy HS, Iacobas I, Hotchkiss K, Hirst-Jensen BJ, Bosco A, Dandachi N, Dermietzel R, Sorgen PL, and Spray DC (2007). The gap junction protein connexin32 interacts with the Src homology 3/hook domain of discs large homolog 1. J Biol Chem. 282: 9789–9796.
- Duga S, Asselta R, Del Giacco L, Malcovati M, Ronchi S, Tenchini ML, and Simonic T (1999). A new exon in the 5′ untranslated region of the connexin32 gene. Eur J Biochem. 259: 188–196.
- Elvira M, Díez JA, Wang KK, and Villalobo A (1993). Phosphorylation of connexin-32 by protein kinase C prevents its proteolysis by mu-calpain and m-calpain. J Biol Chem. 268: 14294–14300.
- Eugenin EA, Gonzalez HE, Sanchez HA, Branes MC, and Saez JC (2007). Inflammatory conditions induce gap junctional communication between rat Kupffer cells both in vivo and in vitro. Cell Immunol. 247: 103–110.
- Field JM, Tate LA, Chipman JK, and Minchin SD (2003). Identification of functional regulatory regions of the connexin32 gene promoter. Biochim Biophys Acta. 1628: 22–29.
- Fischer R, Reinehr R, Lu TP, Schonicke A, Warskulat U, Dienes HP, and Haussinger D (2005). Intercellular communication via gap junctions in activated rat hepatic stellate cells. Gastroenterology. 128: 433–448.
- Fladmark KE, Gjertsen BT, Molven A, Mellgren G, Vintermyr OK, and Døskeland SO (1997). Gap junctions and growth control in liver regeneration and in isolated rat hepatocytes. Hepatology. 25: 847–855.
- Fowler SL, Akins M, Zhou H, Figeys D, and Bennett SA (2013). The liver connexin32 interactome is a novel plasma membrane-mitochondrial signaling nexus. J Proteome Res. 12: 2597–2610.
- Fujimoto K, Nagafuchi A, Tsukita S, Kuraoka A, Ohokuma A, and Shibata Y (1997). Dynamics of connexins, E-cadherin and alpha-catenin on cell membranes during gap junction formation. J Cell Sci. 110: 311–322.
- Ganz M, Csak T, Nath B, and Szabo G (2011). Lipopolysaccharide induces and activates the Nalp3 inflammasome in the liver. World J Gastroenterol. 17: 4772–4778.
- Gaspers LD and Thomas AP (2005). Calcium signaling in liver. Cell Calcium. 38: 329–342.
- Gonzalez HE, Eugenin EA, Garces G, Solis N, Pizarro M, Accatino L, and Saez JC (2002). Regulation of hepatic connexins in cholestasis: Possible involvement of Kupffer cells and inflammatory mediators. Am J Physiol Gastrointest Liver Physiol. 282: G991–G1001.
- Goodenough DA (1974). Bulk isolation of mouse hepatocyte gap junctions. Characterization of the principal protein, connexin. J Cell Biol. 61: 557–563.
- Goubaeva F, Mikami M, Giardina S, Ding B, Abe J, and Yang J (2007). Cardiac mitochondrial connexin 43 regulates apoptosis. Biochem Biophys Res Commun. 352: 97–103.
- Greenwel P, Rubin J, Schwartz M, Hertzberg EL, and Rojkind M (1993). Liver fat-storing cell clones obtained from a CCl4-cirrhotic rat are heterogeneous with regard to proliferation, expression of extracellular matrix components, interleukin-6, and connexin 43. Lab Invest. 69: 210–216.
- Hamilton GA, Jolley SL, Gilbert D, Coon DJ, Barros S, and LeCluyse EL (2001). Regulation of cell morphology and cytochrome P450 expression in human hepatocytes by extracellular matrix and cell-cell interactions. Cell Tissue Res. 306: 85–99.
- Hernández-Guerra M, González-Méndez Y, de Ganzo ZA, Salido E, García-Pagán JC, Abrante B, Malagón AM, Bosch J, and Quintero E (2014). Role of gap junctions modulating hepatic vascular tone in cirrhosis. Liver Int. 34: 859–868.
- Iwai M, Harada Y, Muramatsu A, Tanaka S, Mori T, Okanoue T, Katoh F, Ohkusa T, and Kashima K (2000). Development of gap junctional channels and intercellular communication in rat liver during ontogenesis. J Hepatol. 32: 11–18.
- Johnstone SR, Billaud M, Lohman AW, Taddeo EP, and Isakson BE (2012). Posttranslational modifications in connexins and pannexins. J Membr Biol. 245: 319–332.
- Koenig S, Krause P, Drabent B, Schaeffner I, Christ B, Schwartz P, Unthan-Fechner K, and Probst I (2006). The expression of mesenchymal, neural and haematopoietic stem cell markers in adult hepatocytes proliferating in vitro. J Hepatol. 44: 1115–1124.
- Koffler LD, Fernstrom MJ, Akiyama TE, Gonzalez FJ, and Ruch RJ (2002). Positive regulation of connexin32 transcription by hepatocyte nuclear factor-1alpha. Arch Biochem Biophys. 407: 160–167.
- Kojima T, Mitaka T, Shibata Y, and Mochizuki Y (1995). Induction and regulation of connexin26 by glucagon in primary cultures of adult rat hepatocytes. J Cell Sci. 108: 2771–2780.
- Kojima T, Yamamoto T, Lan M, Murata M, Takano K, Go M, Ichimiya S, Chiba H, and Sawada N (2004). Inhibition of MAP kinase activity moderates changes in expression and function of Cx32 but not claudin-1 during DNA synthesis in primary cultures of rat hepatocytes. Med Electron Microsc. 37: 101–113.
- Kojima T, Yamamoto T, Murata M, Lan M, Takano K, Go M, Ichimiya S, Chiba H, and Sawada N (2003). Role of the p38 MAP-kinase signaling pathway for Cx32 and claudin-1 in the rat liver. Cell Commun Adhes. 10: 437–443.
- Kojima T, Yamamoto M, Mochizuki C, Mitaka T, Sawada N, and Mochizuki Y (1997). Different changes in expression and function of connexin 26 and connexin 32 during DNA synthesis and redifferentiation in primary rat hepatocytes using a DMSO culture system. Hepatology. 26: 585–597.
- Kojima T, Yamamoto M, Tobioka H, Mizuguchi T, Mitaka T, and Mochizuki Y (1996). Changes in cellular distribution of connexins 32 and 26 during formation of gap junctions in primary cultures of rat hepatocytes. Exp Cell Res. 223: 314–326.
- Koo SK, Kim DY, Park SD, Kang KW, and Joe CO (1997). PKC phosphorylation disrupts gap junctional communication at G0/S phase in clone 9 cells. Mol Cell Biochem. 167: 41–49.
- Kren BT, Kumar NM, Wang SQ, Gilula NB, and Steer CJ (1993). Differential regulation of multiple gap junction transcripts and proteins during rat liver regeneration. J Cell Biol. 123: 707–718.
- Krutovskikh VA, Piccoli C, and Yamasaki H (2002). Gap junction intercellular communication propagates cell death in cancerous cells. Oncogene. 21: 1989–1999.
- Kumar NM and Gilula NB (1986). Cloning and characterization of human and rat liver cDNAs coding for a gap junction protein. J Cell Biol. 103: 767–776.
- Kuraoka A, Iida H, Hatae T, Shibata Y, Itoh M, and Kurita T (1993). Localization of gap junction proteins, connexins 32 and 26, in rat and guinea pig liver as revealed by quick-freeze, deep-etch immunoelectron microscopy. J Histochem Cytochem. 41: 971–980.
- Laird DW (2005). Connexin phosphorylation as a regulatory event linked to gap junction internalization and degradation. Biochim Biophys Acta. 1711: 172–182.
- Laird DW (2006). Life cycle of connexins in health and disease. Biochem J. 394: 527–543.
- Lampe PD and Lau AF (2004). The effects of connexin phosphorylation on gap junctional communication. Int J Biochem Cell Biol. 36: 1171–1186.
- Loewenstein WR and Kanno Y (1967). Intercellular communication and tissue growth. I. Cancerous growth. J Cell Biol. 33: 225–234.
- Maes M, Crespo Yanguas S, Willebrords J, Cogliati B, and Vinken M (2015). Connexin and pannexin signaling in gastrointestinal and liver disease. Transl Res. 166: 332–343.
- Meyer DJ, Yancey SB, and Revel JP (1981). Intercellular communication in normal and regenerating rat liver: A quantitative analysis. J Cell Biol. 91: 505–523.
- Miyashita T, Takeda A, Iwai M, and Shimazu T (1991). Single administration of hepatotoxic chemicals transiently decreases the gap-junction-protein levels of connexin 32 in rat liver. Eur J Biochem. 196: 37–42.
- Musil LS, Le AC, VanSlyke JK, and Roberts LM (2000). Regulation of connexin degradation as a mechanism to increase gap junction assembly and function. J Biol Chem. 275: 25207–25215.
- Nakashima Y, Ono T, Yamanoi A, El-Assal ON, Kohno H, and Nagasue N (2004). Expression of gap junction protein connexin32 in chronic hepatitis, liver cirrhosis, and hepatocellular carcinoma. J Gastroenterol. 39: 763–768.
- Nathanson MH, Rios-Velez L, Burgstahler AD, and Mennone A (1999). Communication via gap junctions modulates bile secretion in the isolated perfused rat liver. Gastroenterology. 116: 1176–1183.
- Naves MM, Silveira ER, Dagli ML, and Moreno FS (2001). Effects of beta-carotene and vitamin A on oval cell proliferation and connexin 43 expression during hepatic differentiation in the rat. J Nutr Biochem. 12: 685–692.
- Neuhaus IM, Bone L, Wang S, Ionasescu V, and Werner R (1996). The human connexin32 gene is transcribed from two tissue-specific promoters. Biosci Rep. 16: 239–248.
- Neveu MJ, Hully JR, Babcock KL, Hertzberg EL, Nicholson BJ, Paul DL, and Pitot HC (1994). Multiple mechanisms are responsible for altered expression of gap junction genes during oncogenesis in rat liver. J Cell Sci. 107: 83–95.
- Neveu MJ, Hully JR, Babcock KL, Vaughan J, Hertzberg EL, Nicholson BJ, Paul DL, and Pitot HC (1995). Proliferation-associated differences in the spatial and temporal expression of gap junction genes in rat liver. Hepatology. 22: 202–212.
- Nicholson B, Dermietzel R, Teplow D, Traub O, Willecke K, and Revel JP (1987). Two homologous protein components of hepatic gap junctions. Nature. 329: 732–734.
- Oyamada M, Krutovskikh VA, Mesnil M, Partensky C, Berger F, and Yamasaki H (1990). Aberrant expression of gap junction gene in primary human hepatocellular carcinomas: Increased expression of cardiac-type gap junction gene connexin 43. Mol Carcinog. 3: 273–278.
- Oyamada M, Oyamada Y, and Takamatsu T (2005). Regulation of connexin expression. Biochim Biophys Acta. 1719: 6–23.
- Paku S, Nagy P, Kopper L, and Thorgeirsson SS (2004). 2-Acetylaminofluorene dose-dependent differentiation of rat oval cells into hepatocytes: Confocal and electron microscopic studies. Hepatology. 39: 1353–1361.
- Paul DL (1986). Molecular cloning of cDNA for rat liver gap junction protein. J Cell Biol. 10: 123–134.
- Penuela S, Harland L, Simek J, and Laird DW (2014). Pannexin channels and their links to human disease. Biochem J. 461: 371–381.
- Pfeifer I, Anderson C, Werner R, and Oltra E (2004). Redefining the structure of the mouse connexin43 gene: Selective promoter usage and alternative splicing mechanisms yield transcripts with different translational efficiencies. Nucleic Acids Res. 32: 4550–4562.
- Piechocki MP, Burk RD, and Ruch RJ (1999). Regulation of connexin32 and connexin43 gene expression by DNA methylation in rat liver cells. Carcinogenesis. 20: 401–406.
- Rahman S, Carlile G, and Evans WH (1993). Assembly of hepatic gap junctions. Topography and distribution of connexin 32 in intracellular and plasma membranes determined using sequence-specific antibodies. J Biol Chem. 268: 1260–1265.
- Revel JP and Karnovsky MJ (1967). Hexagonal array of subunits in intercellular junctions of the mouse heart and liver. J Cell Biol. 33: C7–C12.
- Rosenberg E, Faris RA, Spray DC, Monfils B, Abreu S, Danishefsky I and Reid LM (1996). Correlation of expression of connexin mRNA isoforms with degree of cellular differentiation. Cell Adhes Commun. 4: 223–235.
- Sáez JC (1997). Intercellular gap junctional communication is required for an optimal metabolic response of the functional units of liver. Hepatology. 25: 775–776.
- Saez JC, Berthoud VM, Branes MC, Martinez AD, and Beyer EC (2003). Plasma membrane channels formed by connexins: Their regulation and functions. Physiol Rev. 83: 1359–1400.
- Saez CG, Eugenin E, Hertzberg EL, and Saez JC (1997). Regulation of gap junctions in rat liver during acute and chronic CCl4-induced liver injury. In: From Ion Channels to Cell-to-Cell Conversations. New York: Plenum Press, p. 380–383.
- Saez JC, Spray DC, Nairn AC, Hertzberg E, Greengard P, and Bennett MV (1986). cAMP increases junctional conductance and stimulates phosphorylation of the 27-kDa principal gap junction polypeptide. Proc Natl Acad Sci USA. 83: 2473–2477.
- Saito C, Shinzawa K, and Tsujimoto Y (2014). Synchronized necrotic death of attached hepatocytes mediated via gap junctions. Sci Rep. 4: 5169 .
- Shiojiri N, Niwa T, Sugiyama Y, and Koike T (2006). Preferential expression of connexin37 and connexin40 in the endothelium of the portal veins during mouse liver development. Cell Tissue Res. 324: 547–552.
- Shoda T, Mitsumori K, Onodera H, Toyoda K, Uneyama C, Imazawa T, and Hirose M (1999). The relationship between decrease in Cx32 and induction of P450 isozymes in the early phase of clofibrate hepatocarcinogenesis in the rat. Arch Toxicol. 73: 373–380.
- Shoda T, Mitsumori K, Onodera H, Toyoda K, Uneyama C, Takada K, and Hirose M (2000). Liver tumor-promoting effect of beta-naphthoflavone, a strong CYP 1A1/2 inducer, and the relationship between CYP 1A1/2 induction and Cx32 decrease in its hepatocarcinogenesis in the rat. Toxicol Pathol. 28: 540–547.
- Solan JL and Lampe PD (2005). Connexin phosphorylation as a regulatory event linked to gap junction channel assembly. Biochim Biophys Acta. 1711: 154–163.
- Solan JL and Lampe PD (2009). Connexin43 phosphorylation: Structural changes and biological effects. Biochem J. 419: 261–272.
- Söhl G, Gillen C, Bosse F, Gleichmann M, Müller HW, and Willecke K (1996). A second alternative transcript of the gap junction gene connexin32 is expressed in murine Schwann cells and modulated in injured sciatic nerve. Eur J Cell Biol. 69: 267–275.
- Söhl G, Theis M, Hallas G, Brambach S, Dahl E, Kidder G, and Willecke K (2001). A new alternatively spliced transcript of the mouse connexin32 gene is expressed in embryonic stem cells, oocytes, and liver. Exp Cell Res. 266: 177–186.
- Söhl G and Willecke K (2004). Gap junctions and the connexin protein family. Cardiovasc Res. 62: 228–232.
- Spray DC, Bai S, Burk RD, and Saez JC (1994). Regulation and function of liver gap junctions and their genes. Prog Liver Dis. 12: 1–18.
- Stümpel F, Ott T, Willecke K, and Jungermann K (1998). Connexin 32 gap junctions enhance stimulation of glucose output by glucagon and noradrenaline in mouse liver. Hepatology. 28: 1616–1620.
- Sugiyama Y and Ohta H (1990). Changes in density and distribution of gap junctions after partial hepatectomy: Immunohistochemical and morphometric studies. Arch Histol Cytol. 53: 71–80.
- Taub R (2004). Liver regeneration: From myth to mechanism. Nat Rev Mol Cell Biol. 5: 836–847.
- Temme A, Ott T, Dombrowski F, and Willecke K (2000). The extent of synchronous initiation and termination of DNA synthesis in regenerating mouse liver is dependent on connexin32 expressing gap junctions. J Hepatol. 32: 627–635.
- Temme A, Stümpel F, Söhl G, Rieber EP, Jungermann K, Willecke K, and Ott T (2001). Dilated bile canaliculi and attenuated decrease of nerve-dependent bile secretion in connexin32-deficient mouse liver. Pflugers Arch. 442: 961–966.
- Temme A, Traub O, and Willecke K (1998). Downregulation of connexin32 protein and gap-junctional intercellular communication by cytokine-mediated acute-phase response in immortalized mouse hepatocytes. Cell Tissue Res. 294: 345–350.
- Traub O, Drüge PM, and Willecke K (1983). Degradation and resynthesis of gap junction protein in plasma membranes of regenerating liver after partial hepatectomy or cholestasis. Proc Natl Acad Sci USA. 80: 755–759.
- Traub O, Look J, Paul D, and Willecke K (1987). Cyclic adenosine monophosphate stimulates biosynthesis and phosphorylation of the 26 kDa gap junction protein in cultured mouse hepatocytes. Eur J Cell Biol. 43: 48–54.
- Vinken M, De Rop E, Decrock E, De Vuyst E, Leybaert L, Vanhaecke T, and Rogiers V (2009a). Epigenetic regulation of gap junctional intercellular communication: More than a way to keep cells quiet? Biochim Biophys Acta. 1795: 53–61.
- Vinken M, Decrock E, De Vuyst E, De Bock M, Vandenbroucke RE, De Geest BG, Demeester J, Sanders NN, Vanhaecke T, Leybaert L, et al. (2010). Connexin32 hemichannels contribute to the apoptotic-to-necrotic transition during Fas-mediated hepatocyte cell death. Cell Mol Life Sci. 67: 907–918.
- Vinken M, Decrock E, Leybaert L, Bultynck G, Himpens B, Vanhaecke T, and Rogiers V (2012a). Non-channel functions of connexins in cell growth and cell death. Biochim Biophys Acta. 1818: 2002–2008.
- Vinken M, Decrock E, Vanhaecke T, Leybaert L, and Rogiers V (2012b). Connexin43 signaling contributes to spontaneous apoptosis in cultures of primary hepatocytes. Toxicol Sci. 125: 175–186.
- Vinken M, Doktorova T, Decrock E, Leybaert L, Vanhaecke T, and Rogiers V (2009b). Gap junctional intercellular communication as a target for liver toxicity and carcinogenicity. Crit Rev Biochem Mol Biol. 44: 201–222.
- Vinken M, Henkens T, De Rop E, Fraczek J, Vanhaecke T, and Rogiers V (2008). Biology and pathobiology of gap junctional channels in hepatocytes. Hepatology. 47: 1077–1088.
- Vinken M, Henkens T, Snykers S, Lukaszuk A, Tourwé D, Rogiers V, and Vanhaecke T (2007). The novel histone deacetylase inhibitor 4-Me2N-BAVAH differentially affects cell junctions between primary hepatocytes. Toxicology. 236: 92–102.
- Vinken M, Henkens T, Vanhaecke T, Papeleu P, Geerts A, Van Rossen E, Chipman JK, Meda P, and Rogiers V (2006a). Trichostatin a enhances gap junctional intercellular communication in primary cultures of adult rat hepatocytes. Toxicol Sci. 91: 484–492.
- Vinken M, Maes M, Cavill R, Valkenborg D, Ellis JK, Decrock E, Leybaert L, Staes A, Gevaert K, Oliveira AG, et al. (2013). Proteomic and metabolomic responses to connexin43 silencing in primary hepatocyte cultures. Arch Toxicol. 87: 883–894.
- Vinken M, Maes M, Vanhaecke T, and Rogiers V (2013). Drug-induced liver injury: Mechanisms, types and biomarkers. Curr Med Chem. 20: 3011–3021.
- Vinken M, Vanhaecke T, Papeleu P, Snykers S, Henkens T, and Rogiers V (2006b). Connexins and their channels in cell growth and cell death. Cell Signal. 18: 592–600.
- Wang ZS, Wu LQ, Yi X, Geng C, Li YJ, and Yao RY (2013). Connexin-43 can delay early recurrence and metastasis in patients with hepatitis B-related hepatocellular carcinoma and low serum alpha-fetoprotein after radical hepatectomy. BMC Cancer. 13: 306
- Xiao F, Waldrop SL, Khimji AK, and Kilic G (2012). Pannexin1 contributes to pathophysiological ATP release in lipoapoptosis induced by saturated free fatty acids in liver cells. Am J Physiol Cell Physiol. 303: C1034–C1044.
- Yang J, Ichikawa A, and Tsuchiya T (2003). A novel function of connexin 32: Marked enhancement of liver function in a hepatoma cell line. Biochem Biophys Res Commun. 307: 80–85.
- Yee AG and Revel JP (1978). Loss and reappearance of gap junctions in regenerating liver. J Cell Biol. 78: 554–564.
- Zhang D, Kaneda M, Nakahama K, Arii S, and Morita I (2007). Connexin 43 expression promotes malignancy of HuH7 hepatocellular carcinoma cells via the inhibition of cell–cell communication. Cancer Lett. 252: 208–215.
- Zhang JT and Nicholson BJ (1989). Sequence and tissue distribution of a second protein of hepatic gap junctions, Cx26, as deduced from its cDNA. J Cell Biol. 109: 3391–3401.
- Zhang M andThorgeirsson SS (1994). Modulation of connexins during differentiation of oval cells into hepatocytes. Exp Cell Res. 213: 37–42.
- Zou H, Zhuo L, Han T, Hu D, Yang X, Wang Y, Yuan Y, Gu J, Bian J, Liu X, et al. (2015). Autophagy and gap junctional intercellular communication inhibition are involved in cadmium-induced apoptosis in rat liver cells. Biochem Biophys Res Commun. 459: 713–719.